1 Introduction
Systemic lead (Pb) toxicity induced many effects, while acute and prolonged systemic exposure to Pb affects several body systems, such as the gastrointestinal, hematopoietic, cardiovascular, nervous, immune, reproductive, and excretory systems [1,2]. Several data have shown that Pb is able to disturb different neurotransmitter system functions such as the GABAergic, cholinergic, adrenergic, glutaminergic, serotoninergic, dopaminergic, and peptidergic systems [3–9]. Other studies convincingly demonstrated the neurotoxic potential of Pb and suggested it as a menace for PD development [10–12]. Pb is able to act at different levels; thus, chronic exposure to Pb in drinking water diminished TH-expression within SNc and the motor cortex [13]. The disturbance of striatal and cerebellar functions due to the impaired catecholaminergic system [dopamine (DA) and noradrenaline (NA)] could be involved in hyperactivity and motor coordination problems, which were associated with prenatal Pb exposure [13,14]. Furthermore, Pb modulates the release of DA [15]; in fact, after weaning, exposure to 50 and 150 ppm of Pb acetate increased meaningfully DA released after the injection of KCl into the nucleus accumbens [16]. Also, the exposure to a low level of Pb decreases the DA liberation in the nucleus accumbens of rats [17]. In addition, it was revealed that lifetime exposure to lead induces oxidative stress in the rats brain [18]; also, Pb exposure could disrupt synaptic transmission, whereas a study shows that Pb-chronic exposure impairs in vivo generation of long-term potentiation in rat hippocampal dentate [19].
Curcumin, a major compound in the rhizome of Curcuma longa, is commonly used as a nutriment additive in Indian and Chinese traditional medicine [20]. It is also very popular in the kitchen and considered as an integral and ubiquitous spice [21]. Curcumin-III (bisdemethoxycurcumin, BDMC) is an antioxidant [22], a potential antimicrobial agent [23], a potent anti-inflammatory [24], antimutagenic [25], and an antitumor [26]. Valuable properties of BDMC were defined beside neurodegenerative diseases, especially PD [27].
However, the BDMC outcomes against lead-prompted Parkinsonism in rodents have never been clarified. Therefore, we aim in this investigation to evaluate the locomotor performance (vertical and horizontal locomotion) in Meriones acutely exposed to Pb, in parallel with the possible dopaminergic and noradrenergic dysfunctions within SNc, VTA and LC, then to evaluate the putative neuroprotective effect of BDMC.
2 Materials and methods
2.1 Animals
Four-month-old male Meriones, 200–250 g, delivered by the central animal-care facilities of Cadi Ayyad University, Marrakech (UCAM), Morocco, were retained at constant temperature (25 °C) on a 12-h dark–light cycle. All animals have ad libitum access to water and food. All Meriones treatments were completed with respect to the UCAM guidelines. All procedures were in accordance with the European Directive No. 2010/63/UE of 22 September 2010 (adapted into French law by the 2013-118 decree of 1st February 2013, related to the ethical evaluation and authorization of projects using animals for experimental procedures, NOR: AGRG1238767A). Therefore, all efforts were made to minimize the number of suffering animals.
Meriones were separated into four groups:
- • group I: control Meriones (C) (n = 7) were injected intraperitoneally with a physiological saline buffer (NaCl, 9‰) for three consecutive days;
- • group II: Meriones (Pb) (n = 7) received an intraperitoneal injection of Pb (25 mg/kg BW) dissolved in distilled water for three consecutive days;
- • group III: Meriones (CurIII + Pb) (n = 7) pretreated with curcumin-III, dissolved in olive oil, at a dose of 30 mg/kg BW by oral gavage daily for three days. Animals of this group received an intraperitoneal injection with Pb (25 mg/kg BW) 2 h after they had been given the last dose of curcumin-III repeatedly for three days;
- • group IV: Meriones (Cur III) (n = 7) were treated orally with curcumin-III, dissolved in olive oil, at a dose of 30 mg/kg B.W. daily for 3 days and were injected i.p. with a physiological saline buffer (0.9% NaCl).
2.2 Chemicals
Lead (II) acetate trihydrate was supplied by (Panreac Quimica S.L.U., Spain; code No. 2061044, lot No. 0000450930).
Curcumin-III: (1E,6E)-1,7-bis(4-hydroxyphenyl)hepta-1,6-diene-3,5-dione (C19H16O4) provided by Sigma-Aldrich Chemie GmbH, Germany ‘code No. B6938’.
2.3 Open-field test
This test was used to evaluate locomotor performance. The apparatus comprises 25 equal boxes of 20 cm per side (100 cm × 100 cm × 40 cm). Each Meriones was put in the center of the arena, then, the rearings (vertical locomotion) and the total crossed quadrangles (Horizontal locomotion) by each Meriones were checked during 5 min. Prior to the test, Meriones were given habituation periods for 10 min for three continual days [28].
2.4 Immunohistochemistry
After all experiments, Meriones were sacrificed 24 h after the last Pb-injection for the immunohistochemical investigation. Meriones were anesthetized using urethane (40 mg/kg i.p.), then, perfused transcardially with a cool physiological saline buffer (NaCl 0.9%) (Sigma-Aldrich, St. Louis, MO, USA) and paraformaldehyde (PFA) (4%) (Panreac Quimica SA, Barcelona, Spain) in a phosphate saline buffer (PBS, 0.1M, pH7.4) (Riedel-de Haen, Seelze, Germany). Afterward, brains were detached and post-fixed in PFA (4%) for 12 h at 4 °C, then dehydrated through a graded ethanol series (70–100%), passed via polyethylene glycol serial (Merck-Shuchardts, Hohenbrunn, Germany) (PEG: 40 to 100%) solutions and embedded in pure PEG. Coronal sections of 20 μm were made using a microtome according to the rat brain in stereotaxic coordinates and put in a phosphate saline buffer (PBS). Slices were chosen all over the midbrain, through substantia nigra compacta (SNc), ventral tegmental area (VTA) (bregma, 5.3 mm), locus coeruleus (LC) (bregma, 9.6 mm) and the dorsal striatum (bregma, 0.20 mm). The slices were preincubated for 2 h in PBS with 0.3% triton and 0.1% bovine serum albumin (BSA) (Sigma-Aldrich, St. Louis, USA) with agitation; then, they were incubated overnight at 4 °C in a solution of monoclonal TH antibody (Santa Cruz, CA, USA), diluted to 1:1000, comprising PBS (0.1 M, pH 7.4), Triton (0.3%), and BSA (1%). After that, the slices were washed three times by PBS (0.1 M, pH 7.4) during 5 min, before incubation with the secondary antibody (rabbit anti-immunoglobulins, 1/500) (Vector Labs, Burlingame, CA, USA) for 2 h at room temperature. Subsequently, the slices were washed three times and incubated for 2 h in a PBS buffer containing Triton (0.3%) and the avidin–biotin peroxidase complex (Kit ABC 1/500) (Vector Laboratories Burlingame, CA, USA). The revelation of TH-immunoreactivity was performed by the enzymatic reaction of peroxidase in the presence of the 3,3-diaminobenzidine (0.03%) (Sigma-Aldrich, Oakville, Canada) and hydrogen peroxide (0.006%) in Tris buffer (0.05 M pH 7.5). Next, the sections were dehydrated and mounted using Eukit for microscopy analysis and observation. The immunoreactive materials specificity was tested and revealed that the primary antibodies used against TH display specific labeling, as described in our previous studies [28–33].
2.5 Immunolabeling quantification
We performed the quantification of TH-immunoreactivity (TH-IR) in all brain structures, including substantia nigra compacta, ventral tegmental area, locus coeruleus, and dorsal striatum, using Vilaplana and Lavialle's protocol [34]. Images digitization and storage were realized using a Zeiss-Axioskop 40 microscope (Carl Zeiss; Oberkochen, Germany) and a mounted-Nikon digital camera. Images were digitized into 512 × 512 pixels with eight bits of gray resolution and were stored in TIFF format. Image processing and quantification were performed using Adobe Photoshop v.6.0 (Adobe Systems, San Jose, CA, USA). After conversion of each image to the binary mode, the percentages of black pixels were obtained using the image histogram option of Adobe Photoshop. This percentage corresponds to the TH-immunopositive area throughout the whole nucleus or the projections. Five sections from each animal of each group were randomly chosen for the quantification.
2.6 Statistical analysis
Our data were subjected to a one-way analysis of variance (ANOVA). Post-hoc differences between group means were tested with the Tukey test. Data are reported as mean ± S.E.M. and P-values < 0.05 were considered significant. Statistical analyses were performed using computer software SPSS 10.0 for Windows (SPSS, IBM, Chicago, IL, USA).
3 Results
3.1 Outcomes of acute Pb exposure and curcumin-III on locomotor performance
The acutely Pb-intoxicated animals, presented a severe decrease (P < 0.005) of the traversed quadrangles number (horizontal locomotion) and the same for the rearing number (vertical locomotion); such impairments implied an injury of locomotor performance in Pb-intoxicated Meriones (5 ± 0.57) compared to the controls (130 ± 1.7) (Fig. 1A and B). Co-treatment by curcumin-III improved (P < 0.005) the locomotor activity of Pb-treated Meriones (145 ± 1.45); however, no significant variation was proved by Meriones treated by curcumin-III (153 ± 1.7) alone (Fig. 1A and B), when compared to the control group.
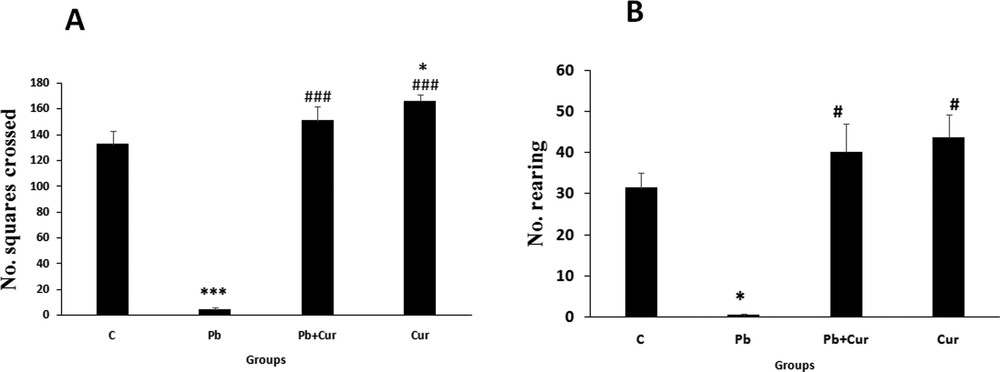
Histograms showing the number of crossed squares during observation in Meriones for 5 min using the open-field test (A; horizontal movement) and the number of rearings (B; vertical movement). C: control, Pb: Pb-treated, Cur + Pb: curcumin-III + Pb-treated, Cur: curcumin-III treated. Data are shown as group mean values ± S.E.M. ***P < 0.005 vs. controls and ### P < 0.005 vs. the Pb group.
3.2 Pb neurotoxicity and restorative potential of curcumin-III on altered nigrostriatal dopaminergic and noradrenergic system
An immunohistochemistry study was performed to assess TH-IRin SNc, VTA, LC and the dorsal striatum. A significant (P < 0.05) increase of TH-IR was observed in Pb-intoxicated Meriones, within SNc (52 ± 0.33) (Fig. 2B), VTA (24 ± 0.55) (Fig. 3B), LC (24 ± 0.88) (Fig. 5B), and the striatum (24 ± 0.66) (Fig. 4B). Both neurons and fibers in intoxicated Meriones were highly immunoreactive compared to controls (Fig. 2A and B). Oral gavage of curcumin-III to Pb-intoxicated Meriones seem to protected DAergic and noradrenergic innervations by restoring TH-IR within SNc and VTA nuclei (P < 0.05) (Figs. 2C, 3C and 5C), also within fibers in striatum (Fig. 4C). In fact, both cells and processes are clearly immunostained, whereas TH-immunoreactivity in Pb-treated Meriones was significantly (P < 0.05) repaired within SNc (25 ± 0.55), VTA (13 ± 0.5) (Figs. 2C, 3C), LC (12 ± 0.55) (Fig. 5C), and in the dorsal striatum (11 ± 0.57) (Fig. 5C). By contrast, curcumin-III alone induced a non-significant augmentation of TH-IR in comparison to the control group (Figs. 2D, 3D, 4D and 5D).
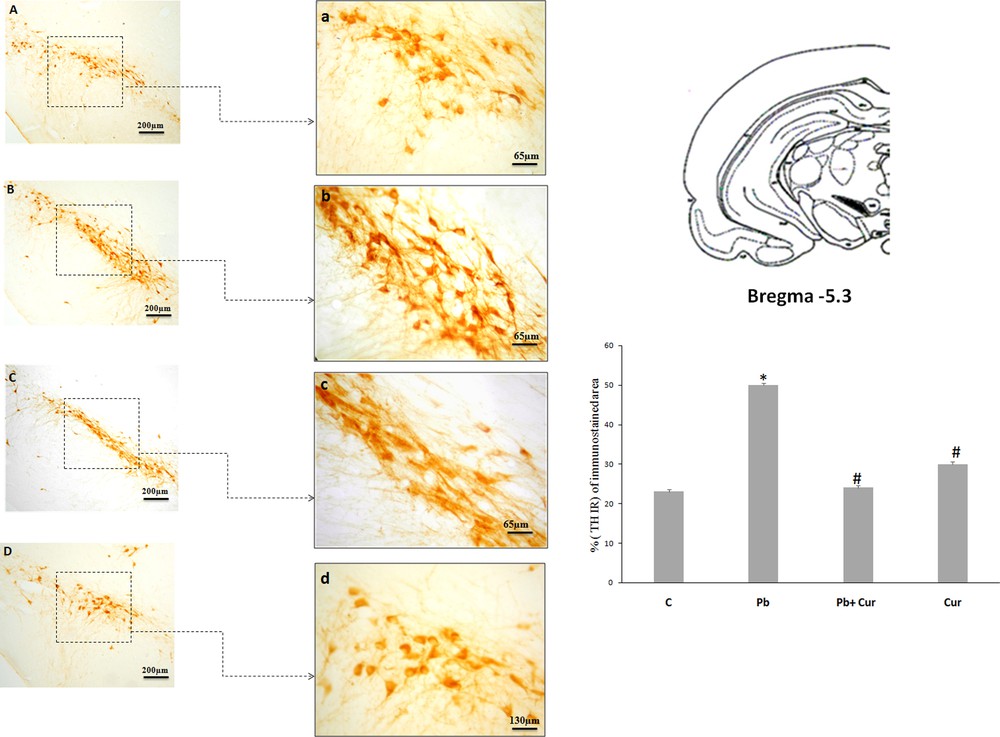
Light micrographs of frontal sections through substantia nigra compacta (SNc) immunolabelled with antiserum against tyrosine hydroxylase (TH) in control (A), Pb-treated (B), Pb + Cur (C), Cur (D): a, b, c, d: high magnification of (SNc) in control, Pb-treated, Pb + Cur treated, and Cur treated, respectively. Data are shown as group means ± S.E.M. * P < 0.05 vs. controls; and # P < 0.05 vs. the Pb group.
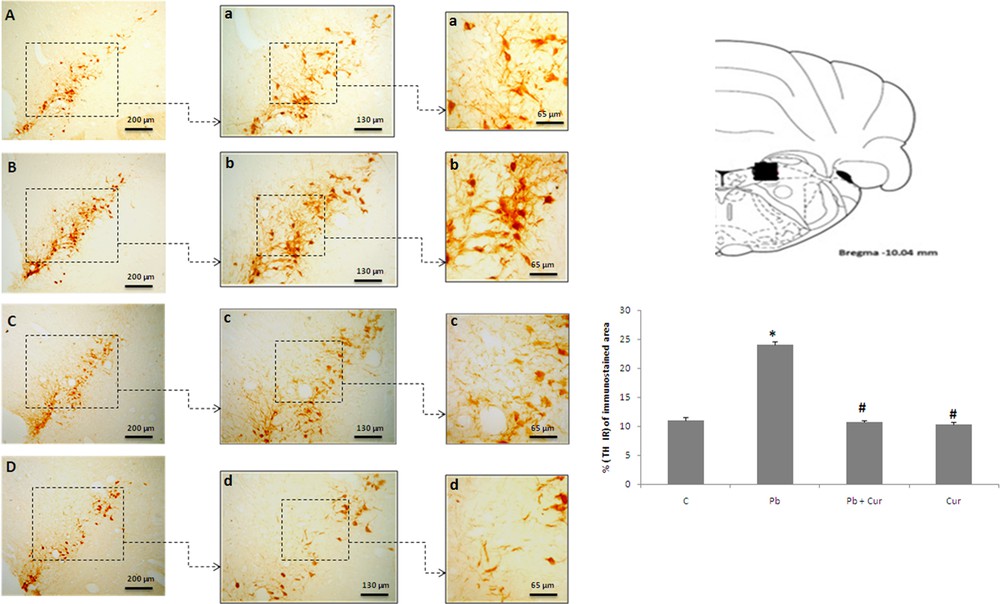
Light micrographs of frontal sections through ventral tegmental area (VTA) immunolabelled with antiserum against tyrosine hydroxylase (TH) in control (A), Pb-treated (B), Pb + Cur treated (C), Cur treated (D). Data are shown as group means ± S.E.M. * P < 0.05 vs. controls; and # P < 0.05 vs. the Pb group.
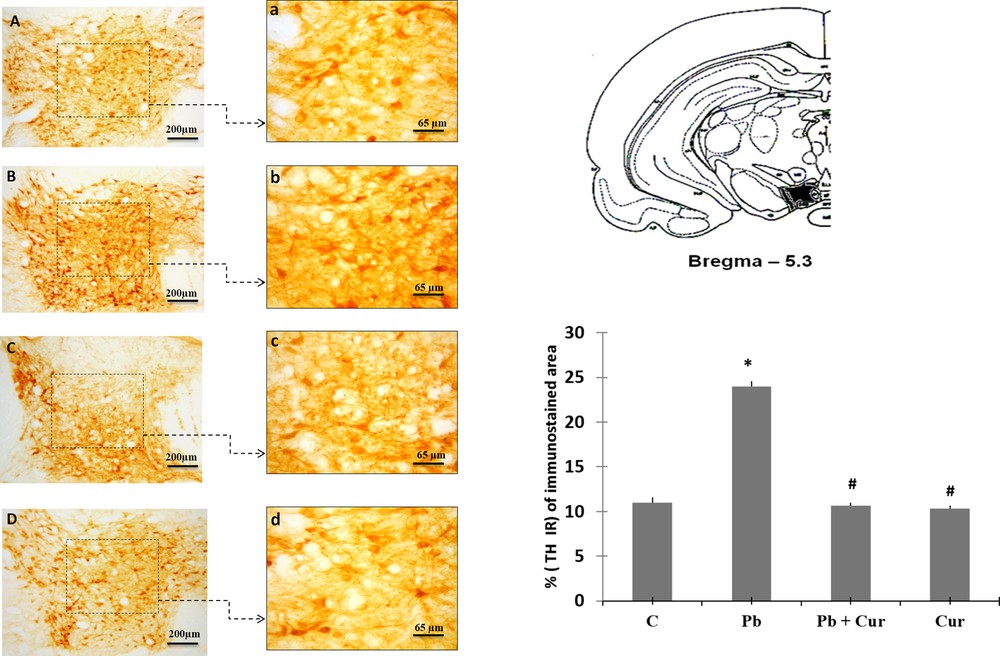
Light micrographs of frontal sections through locus coeruleus (LC) immunolabelled with antiserum against tyrosine hydroxylase (TH) in control (A), Pb-treated (B), Pb + Cur treated (C), Cur treated (D). Data are shown as group means ± S.E.M. * P < 0.05 vs. controls; and # P < 0.05 vs. the Pb group.
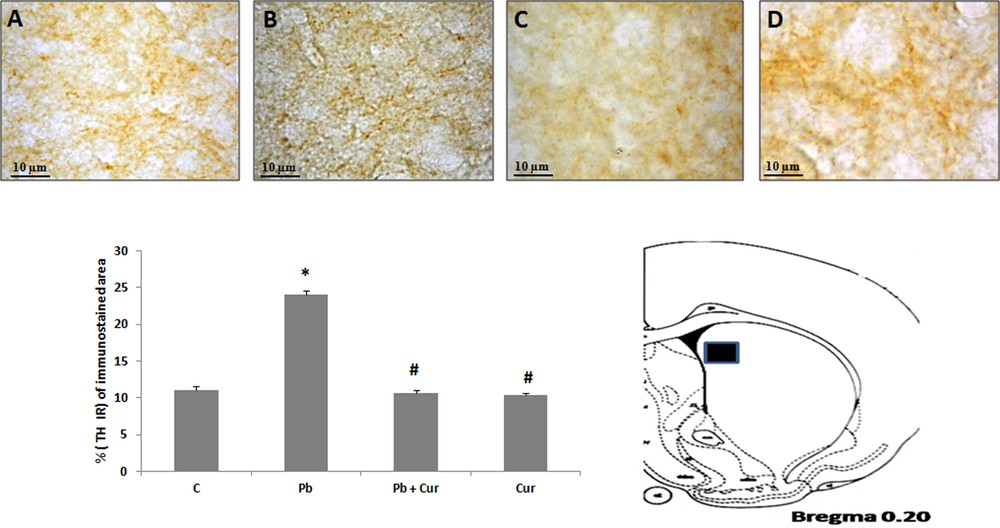
Light micrographs showing tyrosine hydroxylase (TH)-immunopositive fibers in the dorsal striatum of control (A), Pb-treated (B), Pb + Cur treated (C), Cur treated (D) Meriones. Data are shown as group means ± S.E.M. * P < 0.05 vs. controls; and # P < 0.05 vs. the Pb group.
4 Discussion
4.1 Pb intoxication increased TH-IR and locomotor activity
Our results showed that systemic Pb-toxicity increased TH-IR within SNc, VTA, and noradrenergic neurons in locus coeruleus as well as it increased TH-IR and density fibers within the striatum. These alterations may possibly be the cause of locomotor damages perceived in Pb-intoxicated Meriones. Studies support this finding; in fact, it has shown that prenatal exposure to Pb in rats at different ages induces synaptosomal increase of DA in the cerebellum, the hippocampus, and the cerebral cortex [3,35]. Similarly, chronic Pb post-weaning exposure (11 weeks and/or 11 months) showed increased DA in the nucleus accumbens [16]. Another study showed enhanced striatal DA release following systemic acute Pb intoxication at a dose of 6 g/kg [36]. Furthermore, maternal exposure to lead induces variations in the monoamine amount, with increases in dopamine and serotonin or their metabolites [5]. Activation of TH implicated calcium calmodulin-dependent phosphorylation; thus, studies have reported that lead increases calmodulin activity in the brain [37]. So, by competing with calcium, Pb is able to enhance TH activation [38].
We observed a diminution of locomotor performance in Pb-intoxicated Meriones, which is reliable to the neuromodulatory effect of dopamine in the nigro-cortico-striatal conduits in locomotor behavior [39]. Other studies support our data, revealing that systemic Pb disrupts locomotor activity, also, Pb-exposed mice at a dose of 100 mg/kg for two days decreased locomotor behavior [40]. In addition, the observed hypoactivity was described by other studies showing that exposure to Pb at a dose of 5 g/L for three months reduced significantly locomotor activity [13]. Some investigations have linked neurobehavioral impairments to the neurotoxic outcomes of Pb on the dopaminergic, cholinergic, and serotoninergic neurotransmitters systems. We suppose that Pb disturbs locomotion in Meriones by touching dopamine synthesis or its release [41]. Studies have shown that prenatal exposure to Pb, which causes alteration in locomotor activity revealed by the open-field, can be attributed to disturbances of the cholinergic system and aminergic systems (DA, NA) at the hippocampus [3,42]. It has also showed that other metal elements decreased locomotor performance, especially copper (Cu), by disturbing TH-expression [28–31].
4.2 Curcumin-III restored DAergic, noradrenergic, and locomotion deficit in Pb-intoxicated Meriones
Our study showed a neuroprotective potential of curcumin-III against DAergic neurotoxicity generated by Pb. Curcumin-III recovered TH-IRin SNc, VTA, LC and dorsal striatum, then improved the locomotor performance in Pb-intoxicated Meriones. Curcumin-III itself, compared to the control animals, shows a slight effect on horizontal locomotion, but several studies showed that the group treated only by curcumin did not demonstrate high significant change of locomotor activity [30], also in a rat model of postoperative pain; all the doses of curcumin did not expressively alter the spontaneous locomotor activity in a rat model of postoperative pain [43]. Furthermore, curcumin showed no significant effect on open-field locomotor activity [44]. This result is consistent with a previous study showing that curcuminoids [curcumin (CUR), demethoxycurcumin (DMC)], and BDMC provide significant protection against neuronal degeneration in nigrostriatal system damage in 6-OHDA-induced PD in Wistar rats [27]. This molecule has demonstrated a potent neuroprotective effect against lead-induced neurotoxicity in Wistar rats [45]. Furthermore, a study showed that curcuminoids provide neuroprotective effects against neurodegenerative disorders, while a standard extract of curcuminoids containing 78.1% of curcumin, 16.5% of demethoxycurcumin, and 5.4% of bisdemethoxycurcumin displayed a neuroprotective potential against inflammation-mediated dopaminergic neurodegeneration in the MPTP Model of PD [46]. However, studies showed that the protective effect of BDMC was not due to the central effect only, while BDMC have a strong systemic anti-inflammatory effect [24]. This neuroprotective effect of BDMC in the nigrostriatal system might be explained by many mechanisms. Some studies revealed an inhibitor effect of different curcuminoids on the β-amyloid protein, while BDMC could reduce the proteolysis of site APP cleaving enzyme 1 (BACE1) mRNA and protein levels in swAPP HEK293 cells; BDMC could be used for modulation of BACE1 and as a treatment of Alzheimer's disease (AD) [47]. Also, curcumin and its metabolite tetrahydrocurcumin have an inhibitory effect on MAO-B in the MPTP model of Parkinson's disease in mice [48]. A recent study described an inhibitor effect of curcumin on human MAO-A and MAO-B activity [49]; at that point, MAO-A and MAO-B are implicated in depression, PD, and AD [50,51]. In addition, an antioxidant power could be involved in the neuroprotective effect of curcumin-III. In fact, it has been shown that curcuminoids including BDMC have an antioxidant potential and may protect PC12 rats from pheochromocytoma [52]. Furthermore, BDMC defends against oxidative stress in rats [53]. Curcumin can also defend against ROS production via its antioxidant potential [54]. On the other hand, it prevents neurotoxicity through its scavenging capacity of metal elements [45], then improved locomotor performance in two animal models of copper-intoxication-induced locomotor deficiency [28,30].
5 Conclusion
Our study revealed an obvious neurotoxic consequence of Pb exposure on both the dopaminergic and noradrenergic systems together with locomotor activity disturbance. These toxic effects were restored by BDMC. As a result, curcumin-III could be used as a healing compound against Pb neurotoxicity, especially for DAergic and noradrenergic systems disorder.
Disclosure of interest
The authors declare that they have no competing interest.
Acknowledgements
Moroccan/Tunisian Action. Reference: M/T: 03/17.