1 Introduction
Transcription factor p53 has been intensively investigated due to its potential role in the deletion of non-ovarian and ovarian cells with DNA aberrations that can induce malignant transformation. It targets a number of genes related to cell cycle, reparation, apoptosis, insulin-like growth factor signaling, and cancerogenesis. Transcription factor p53 stops the proliferation of defective cells, promotes their DNA repair, and, if this repair is not successful, induces their apoptosis and death [1,2]. The downregulation or mutation of this transcription factor often results in the promotion of cell proliferation, suppression of apoptosis, and tumorigenesis [3]. There are only a few and contradictory evidences regarding p53 involvement in the control of healthy animals’ ovarian cells. Transfection of healthy porcine ovarian granulosa cells with cDNA constructs encoding p53 prevented cell proliferation (accumulation of the proliferation markers PCNA and cyclin B1) and induced apoptosis (accumulation of the apoptosis-related peptide bax). Furthermore, it changed hormone secretion by these cells: inhibited progesterone (P4) and prostaglandin E (PGE) and increased oxytocin (OT) and prostaglandin F (PGF) release [4,5]. Pharmacological blockage of p53 in cultured macaque granulosa cells decreased steroidogenic enzymes and the release of P4 and pregnenolone, but increased estradiol synthesis, and did not affect markers of proliferation (including PCNA) [6]). These studies performed on porcine and monkey cells reveal that p53 can be involved in the control of healthy animal ovarian granulosa cell proliferation, apoptosis, and hormone release, but that important differences in p53 effects among various species can occur. The role of p53 in the control of functions of healthy human ovarian cells has not been studied, yet.
The aim of the present study was to examine the role of p53 in the control of basic healthy human ovarian cell functions, namely, in proliferation, apoptosis, and hormone release. For this purpose, women ovarian granulosa cells were transfected with a cDNA construct encoding p53. The intracellular accumulation of p53, of the proliferation marker PCNA [7], and of the apoptosis marker bax [8], as well as the release of P4, insulin-like growth factor I (IGF-I), OT, PGF, and PGE, the known hormonal markers and regulators of ovarian functions [9,10], were analyzed.
2 Material and methods
2.1 Preparation, transfection, culture, and processing of granulosa cells
Granulosa cells were aspirated from ovaries of women aged 36–42 years with normal ovarian cycles and morphology, after ovariectomy due to non-metastatic cancer of the uterine cervix, 1–5 days after spontaneous ovulation. Informed consent was obtained from all patients, in accordance with EU and Slovak regulations, under agreement and supervision of the local ethical committee. Granulosa cells were isolated, processed, and transfected as described previously [4,5]. Briefly, granulosa cells aspirated from 3–5 mm ovarian follicles were isolated by centrifugation for 10 min at 200 g, rinsed in the culture medium DMEM/F-12 1:1 supplemented with 2% fetal calf serum (all from Sigma, St. Louis, MO, USA), and transfected with the plasmid pcDNA3/pc53-SN3, containing a gene conferring resistance to ampicillin, or with the “scramble” control plasmid without p53 insertion (control), kindly provided by Dr. N. D. Perkins (University of Dundee, UK), and with the marker reporter plasmid pEGFP-N1, for the expression of the enhanced green fluorescent protein (EGFP), providing resistance to kanamycin (Clontech, Mountain View, CA, USA). All constructs were subcloned as reported by Sirotkin et al. [4,5]. Transfection was performed using the lipofection reagent Roti Fect (Carl Roth GmbH + Co. Karlsruhe, Germany), according to the manufacturer's instructions. After transfection, cells (1 × 105 cells/ml) were cultured in DMEM/F-12 1:1 medium supplemented with 2% fetal calf serum and 1% antibiotic-antimycotic solution (all from Sigma, St. Louis, MO, USA) in 24-well Falcon plates (Becton Dickinson, Lincoln Park, NJ, USA), with 2 ml of medium per well, and in chamber slides (Nunc Inc. Naperville, TN, USA), with 200 μl medium per well, at 38 °C and 5% CO2 in humidified atmosphere. After 2 days of pre-culture, the medium was replaced with fresh medium. Two days later, cell numbers and viability were determined by Trypan blue staining and counted in an hemocytometer (the viability was 85–93%). No statistically significant differences in these indices between control and experimental groups were observed. Thereafter, the medium or cells were collected and stored as previously described [4,5] for quantitative immunocytochemistry or RIA.
2.2 Immunocytochemical analysis
Determination of the presence and quantification of p53, PCNA, and bax were performed by immunocytochemistry as it was described previously [4,5] using primary mouse monoclonal antibodies (Santa Cruz Inc., Santa Cruz, CA, USA; dilution 1:500) reacting with the corresponding antigens of human, rat, mouse, and yeast origin. Porcine polyclonal antibodies against mouse IgGs, labelled with fluorescein isothiocyanate (FITC) (Sevac, Prague, Czech Republic), were used as secondary antibody. After staining, cells were embedded in Vectashield mounting medium (Vector Laboratories, Inc., Burlingame, CA, USA). The specificity of primary antibodies and the molecular weights of the ligands were previously confirmed by western blotting [5]. Cells treated with the secondary antibody but not with the primary antibody were used as negative controls. The presence of antigens/FITC and EGFP in cells was detected by fluorescence microscopy. The percentage of cells containing visible antigen was assessed.
2.3 Radioimmunoassay
The concentrations of P4, IGF-I, OT, PGF, and PGE were determined by RIA in 25–100 μl samples of incubation medium. The release of P4 and IGF-I was assayed using RIA kits from Immunotech (Marseille, France), according to the manufacturer's instructions; OT, PGF, and PGE were analyzed by our own RIA systems [11,12]. The characteristics of these assays are presented in Table 1.
Characteristics of the immunoassays used in the experiments.
Hormone assayed | Specificity of assay (cross-reactivity of antiserum) |
Sensitivity of assay (ng/ml) |
Progesterone | <0.001% to cortisol, corticosterone, cortisol, androstenediol, pregnenolone, estradiol, testosterone | 0.12 |
Oxytocin | <0.01% to arginine-vasopressin, lysine.-vasopressin, arginine-vasotocin, somatostatin | 0.015 |
PGF-2 alpha | <0.01% to PGA-1, PGA-2, PGB-1, PGB-2, <0.1% to PGE-1, PGE-2, 66% to PGF-1, 100% to PGF-2 | 0.005 |
PGE-2 | <28.0% to PGA-1, <7.0% to PGA-2, <0.6% to PGB-1, <1.4% to PGB-2, <5.0% to PGF-1, <1.5% to PGF-2, 165% to PGE-1, 100% to PGE-2 | 0.015 |
IGF-I | <1.9% to IGF-II, <0.01% to insulin, proinsulin, <0.001% to EGF, oxytocin and progesterone | 0.3 |
2.4 Statistics
Each series of experiments was performed in triplicate. The data shown are the mean values obtained in these three experiments, performed in distinct days with separate groups of granulosa cells, each obtained from 5–8 donors. For immunocytochemistry, 500 cells were counted in each chamber (three per group); therefore, each value represents the mean of 3 wells × 3 experiments = 9 replicates (i.e. a total of 4500 cells per group). For each group, the percentage of cells positive for the antigen was calculated. For RIA, each experimental group was represented in four culture wells, i.e. each value represents the mean of 4 wells × 3 experiments = 12 replicates. The assays for hormone quantification were performed in duplicate. The values of blank controls (serum-supplemented medium incubated without cells) were subtracted from the specific values determined by RIA in cell-conditioned medium to exclude any non-specific background (less than 8% of total values). Rates of secretion were calculated per 106 viable cells/day. The statistical significance of differences between treatments was evaluated by two-way ANOVA followed by Tukey's or Chi2 test, using Sigma Plot 11.0 (Systat Software, GmbH, Erkhart, Germany). Differences at P < 0.05 were considered significant.
3 Results
After transfection, the cultured ovarian cells expressed p53 and markers of proliferation (PCNA), apoptosis (bax), and transfection (EGFP). All the antigens were located mainly in the cell cytoplasm. Approximately 50% of the granulosa cells produced EGFP (Fig. 1). The cells secreted substantial amounts of P4, IGF-I, OT, PGF, and PGE.

Images of cultured human granulosa cells expressing p53 (A, cells transfected with the p53 cDNA construct), proliferating cell nuclear antigen (PCNA) (B, control cells), bax (C, cells transfected with the p53 cDNA construct), and enhanced green fluorescent protein (EGFP) (D, control cells). Fluorescence microscopy. The detected molecules are marked by either fluorescein isothiocyanate (FITC) (A–C) or EGFP (D); green fluorescence. Scale bars = 10 μm.
Transfection of granulosa cells with the cDNA construct encoding p53 resulted in a significant increase in the percentage of cells containing p53 (Fig. 2A) and bax (Fig. 2B) and in a decrease in the proportion of PCNA-positive cells (Fig. 2C). No statistically significant differences were observed between non-transfected and p53-transfected cells regarding the release of P4 (Fig. 2D), IGF-I (Fig. 2E), or OT (Fig. 2F). Transfection with p53 reduced PGF (Fig. 2G) and promoted PGE (Fig. 2H) output.
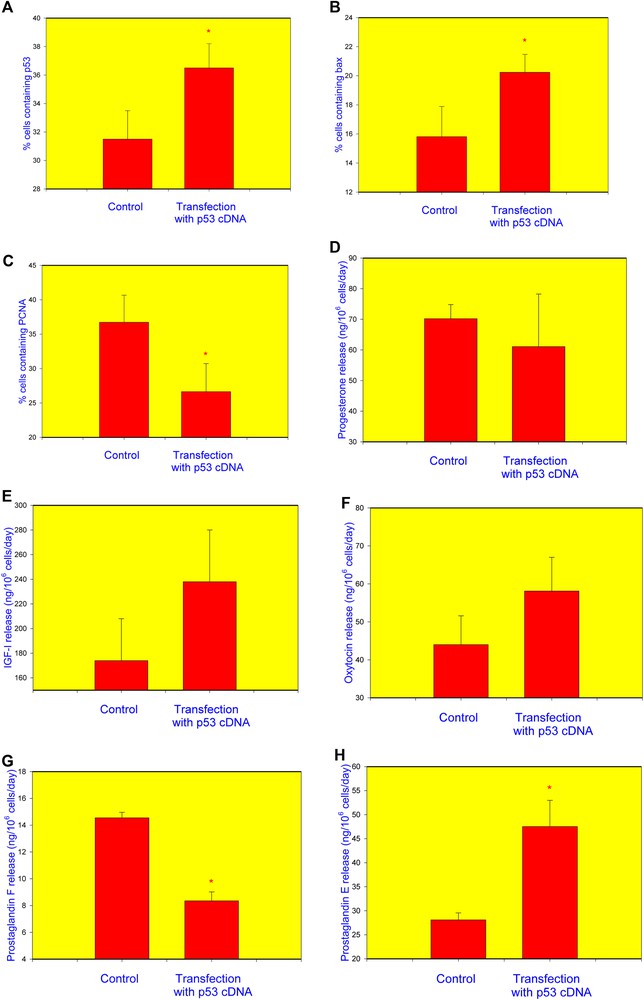
Influence transfection of healthy human granulosa cell with the p53 cDNA construct on the accumulation of p53 (A), bax (B), PCNA (C), and release of progesterone (P4) (D), insulin-like growth factor I (IGF-I) (E), oxytocin (OT) (F), prostaglandin F (PGF) (G), and prostaglandin E2 (PGE) (H). Data from quantitative immunocytochemistry (A–C) and RIA/IRMA (E–H). *p < 0.05, between corresponding groups of cells transfected and non-transfected with the p53 construct.
4 Discussion
Cell attachment to the well bottom, formation of a cell monolayer, high cell viability, presence of proliferation markers, EGFP production, and hormone release in both transfected and non-transfected cells indicated that the ovarian cells used in the experiments and subjected to transfection and culture were viable and suitable for further experiments. The presence of the transfection marker EGFP, as well as the increased accumulation of p53 in the cells transfected with the p53 gene construct indicated a successful transfection, integration, and expression of the used gene constructs.
In our studies, the transfection-induced p53 overexpression was associated with the promotion of bax, inhibition of PCNA accumulation, and changes in PGF and PGE, but not in P4, IGF-I, or OT release. This is the first demonstration of the involvement of p53 in the control of healthy human ovarian cell functions. These observations are in line with previous evidence concerning pro-apoptotic and anti-proliferating p53 action on healthy porcine ovarian cells [4,5] and human cancer ovarian cells [1–3]. On the other hand, this action in healthy ovarian cells in humans differed from that in macaques, where no effects of p53 on proliferation markers have been detected. Furthermore, our studies demonstrated the principal species-specific differences in p53 action on the secretory activity of healthy ovarian cells. In macaques, p53 suppressed P4 release [6], but in women it had no effect. In pigs, p53 inhibited P4 and PGE release and increased that of OT and PGF [4,5], whereas the opposite effect in PGF and PGE release and no effect on P4 and OT have been observed in women. These data demonstrate the main differences in the role of p53 in the control of ovarian functions in different species and, probably, its role in the prevention and treatment of ovarian cancer. From a practical viewpoint, these data raise doubts on the usefulness of animal models for the investigation of p53 action on human ovarian functions, cancerogenesis, and its treatment.
The hierarchical functional interrelationships between different parameters affected by p53 require a better understanding. It is possible that p53 affects ovarian cell proliferation and apoptosis via changes in hormone release. IGF-I, OT, steroid hormones (including P4), PGFs and PGE are known regulators of proliferation and apoptosis and are hormones released by healthy [9,10] and cancer [1,13–15] ovarian cells. On the other hand, in our experiments, the p53 action on proliferation and apoptosis markers was not associated with changes in P4, IGF-I, or OT, whilst, in ovarian cancer cells, no p53 influence on hormone release has been reported yet. These data suggest that both p53 and the hormones P4, IGF-I, and OT can be involved in the control of both healthy and cancer ovarian cell proliferation and apoptosis, but that they regulate these processes in an independent way. In our experiments, PGF and PGE were affected by p53. These prostaglandins can be involved in the proliferation and apoptosis of healthy ruminant [9] and human cancer [12,13] cells. Therefore, it cannot be excluded that p53 can affect human ovarian cells via PGs. This hypothesis needs to be addressed with further experiments.
Taken together, the present data are the first demonstration of p53 involvement in the control of healthy human ovarian cells. In both healthy and malignant human ovarian cells, p53 exerts mainly an inhibitory action: promotion of apoptosis and suppression of proliferation. In addition, the present results show, for the first time, the involvement of p53 in the control of human ovarian hormones (prostaglandins) that can be potential mediators of p53 action. These data indicate that drugs targeting p53 could be applicable not only in the treatment of ovarian cancer, but also in the regulation of healthy ovarian cell functions and fecundity.
Contribution of authors
A. V. Sirotkin generated the general concept of the study and the resulted manuscript, A. Benco performed the in-vitro experiments, M. Mlyncek provided biological material for experiments, A. H. Harrath and S. Alwasel provided funding, contributed to generation and editing the manuscript, J. Kotwica performed hormones analysis.
Disclosure of interest
The authors declare that they have no competing interest.
Acknowledgements
The authors express their gratitude to Dr. N. D. Perkins (University of Dundee, Dundee, UK) for kindly providing the construct for p53 expression and the control plasmid, as well as the colleagues from the Research Institute for Animal Production, Luzianky, Slovakia: Dr. D. Vasicek, for plasmid cloning, and Ms. K. Tothová and Ž. Kuklová, for technical assistance. Furthermore, the authors express their gratitude to Professors G. Kotwica (University of Warmia and Mazury, Olsztyn, Poland), W. W. Thatcher (University of Florida, Gainesville, FL), and W. J. Silvia, (University of Kentucky, Lexington, USA) for kind provision of antisera against OT, PGE2, and PGFM. This work was supported by the Slovak Research and Development Agency (APVV), under the contract numbers APVV-14-0001 and APVV-15-0296, by the Slovak Grant Agency of the Ministry of Education, Science and Sport and of the Slovak Academy of Science (VEGA), the project VEGA 1/0392/17, and the Deanship of Scientific Research at King Saud University through RG-164.