1 Introduction
Most of the chemical paper pulps are produced by the kraft process that consists in wood delignification by a water solution of sodium hydroxide and sodium sulphide at high temperature (170 °C). Unfortunately, lignin removal is never complete and the residual lignin is eliminated in the bleaching process. Analysis of the structure of residual lignin is a difficult task. One problem is due to the fact that lignin is part of a carbohydrate matrix in which it represents only a few percent in weight. Although in situ characterisation has been tempted, lignin isolation is today the preferred approach. The most common technique is acidolysis in dioxane–water 82:18 (v/v) with 0.1 N HCl under reflux [1]. It gives a lignin sample of high purity, but at a yield which is generally lower than 50%. Whether or not the lignin obtained from acidolysis is sufficiently representative of the whole residual lignin has been a matter of discussion for several years. Moreover, some modification to the lignin structure does occur during this acidolysis technique [2]. Other extraction procedures have been developed, such as the enzymatic method where the carbohydrates are digested by an enzymatic cocktail containing various cellulases and hemicellulases [3]. Lignin is then left intact as a residue that can be recovered. This method has not gained much popularity so far because the lignin sample is heavily contaminated by proteins [2,4]. Recently, a mixed isolation technique combining enzymatic digestion of the carbohydrates and acidolysis under milder conditions has been proposed [5]. Yield and purity figures lie between those of the two previous methods.
A good knowledge of the structure of residual lignin would represent a real breakthrough in pulping and bleaching chemistry. One has to understand why some lignin does not dissolve during the kraft process and is then so difficult to be removed during the bleaching process. The occurrence of lignin–carbohydrates bonds has been recently demonstrated [6], but the extent to which they prevent lignin from dissolution during cooking is not known. Moreover, the bleaching chemistry has developed without any solid knowledge of the real structure of the substrate to be treated.
The purpose of this paper is to propose an alternative procedure for lignin isolation consisting in a mild acidolysis with ZnCl2 in an acetic acid–water (80:20) mixture, as already described in two previous papers [7,8].
2 Experimental
2.1 Pulp sample
The pulp sample was an unbleached softwood kraft pulp provided by a Swedish mill. The wood used in the mill is a mixture of Nordic softwoods (roughly 50% Picea abies, 50% Pinus sylvestris). This pulp was thoroughly washed with distilled water and air-dried. It was extracted first with acetone for 6 h and then with dichloromethane for 6 h to remove the extractives. Its lignin content was measured according to the Klason lignin procedure (acid insoluble + acid soluble) described in the TAPPI T222 OM-83 standard. The value was 3.7% (corresponding to a kappa number of 25).
2.2 Lignin-extraction procedures
Extraction of lignin by enzymatic hydrolysis of carbohydrates was performed by applying a commercial cellulase–hemicellulase preparation (Onozuka R-10, Yakult Pharmaceutical Industries, Tokyo, Japan) at pH 4.6 (acetic acid–sodium acetate buffer) and 37 °C [4]. This commercial preparation was a solution of α amylase (0.5 U mg−1, pectinase (0.1 U mg−1), endo-1,3 glucanase (0.2 U mg−1), xylanase (10 U mg−1), β glucosidase (0.04 U mg−1), protease (8 U mg−1), and monomeric sugars (60% on dry material). The content in N accounted for 4.2% (on dry material) only. Five successive enzyme treatments of 72 h each were carried out. For each treatment, the quantity of commercial solution represented about 10% of the quantity of pulp or solid residue (by weight). Consistency was 5%. The last residue was dissolved in dioxane–water (9:1) and after evaporation solubilized in DMF and then precipitated with ethyl ether. Part of the lignin went into solution during the enzymatic hydrolysis. It was recovered by acidification to pH 2 with HCl, dissolution in DMF and precipitation with ethyl ether.
Conventional acidolysis was performed according to the procedure described in [1]. The pulp sample (100 g dry weight) was refluxed for 2 h with 1000 ml of 0.1 M HCl in dioxane–water 82:18 (v/v). Thereafter the pulp was filtered and washed with dioxane–water 82:18. The filtrate was evaporated at 40 °C and then precipitated in water. The precipitate was then centrifuged, washed, and dried at 40 °C under vacuum using P2O5 as desiccant.
Acidolysis with acetic acid and ZnCl2 has been described in earlier papers [7,8]. ZnCl2 acts as a Lewis acid. 100 g of dry pulp was extracted under reflux with acetic acid (80% volume in water), containing ZnCl2 (35% on pulp). Extraction was carried out during 30 min. The mixture was filtered on a glass funnel (number-4 porosity index) and washed with ml fresh acetic acid–water liquor. The filtrates and washing solutions were mixed and evaporated at 40 °C. Concentration was stopped when the total volume reached 100 ml. The lignin was precipitated by addition of 500 ml of water. The precipitated lignin was separated by filtration through a fine porous glass filter (number 4 porosity index), washed with water and ethyl ether to eliminate acetic acid and dried at 40 °C.
2.3 Lignin analysis
Elemental analysis was performed by CNRS (Vernaison, France). Carbohydrate content in lignin was determined by using the procedure described in [4]. Methoxyl group determination was performed according to the Zeisel method.
The 13C NMR spectra have been recorded on a Bruker AC300 spectrometer. The samples were dissolved in DMSO-d6 (300 to 350 mg in 1.8 ml), and placed in 10 mm tubes. The inverse gate decoupling (INVGATE) sequence, which allows quantitative analysis, has been used with the following recording parameters: pulse angle , pulse delay 12 s, sweep range 250 ppm, 7000 scans for each sample. The quaternary carbons were obtained by the QUAT sequence, as described in [9]. Signal assignment was made according to [10]. Lignin acetylation was performed according to the procedure given in [9].
2.4 Model compound
1-(4-hydroxy-3-methoxyphenyl)-2-(2′-methoxyphe noxy)-propanol-1 (Fig. 1) was chosen as a model for β-O-4 aryl–ether structure in lignin. It was synthesized according to the procedures described in [11,12]. Its structure was confirmed by proton NMR spectroscopy at 500 MHz (Bruker AM 500) in CDCl3. The conversion yield of the model compounds under the conditions used for lignin extraction was assessed by measuring guaiacol (1) by GC/MS (HP 5995 apparatus) ().
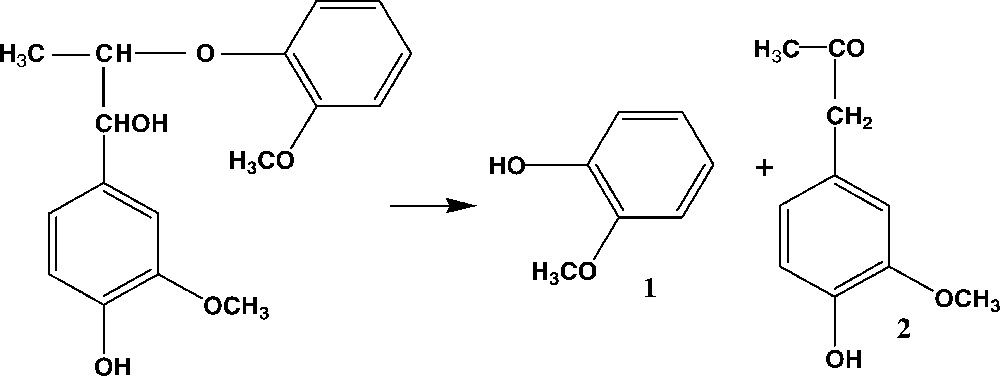
1-(4-hydroxy-3-methoxyphenyl)-2-(2′-methoxyphenoxy)-propanol-1 and its main reaction products after acidolysis. 1: Guaiacol, 2: 1-(4-hydroxy-3-methoxyphenyl)propanone-2.
3 Results and discussion
The three isolation techniques have been first characterised by the yield of the lignin samples extracted from the softwood kraft pulp and by the degree of purity of these samples (Table 1). It is shown that the enzymatic procedure gives by far the highest quantity of crude lignin (80%). However, in this case, the degree of purity is lower. Impurities are mainly carbohydrates and proteins. Most of the carbohydrates originate from the pulp. Further tentative purification in order to reduce the content in carbohydrates failed. Although there is no proof that lignin is covalently linked to these carbohydrates, these results would support this hypothesis. Most of the N impurity must belong to the proteins of the enzymatic cocktail. However, the development of microorganisms during the enzymatic hydrolysis cannot be excluded. A rough calculation gives a corresponding protein contamination close to 6%. Again this level could not be reduced by further purification steps. The possible responsibility of some residual DMF (used in the purification steps) for the presence of nitrogen in the lignin sample has been excluded by carrying out the same purification on other lignin samples containing no nitrogen. The high S content is due to the use of Na2S during the kraft delignification process. According to the lignin chemistry, the SH− anion adds to some lignin structures (quinone-methide) and favours the cleavage of adjacent ether linkages.
Yield and purity of the lignin samples extracted from a softwood kraft pulp by three different procedures
Enzymatic | Dioxane–water–HCl | Acetic acid–water–ZnCl2 | |
Yield (% in pulp lignin) | 80 | 35 | 62 |
N (% in lignin sample) | 0.9 | 0 | 0 |
Protein (% in lignin sample)* | 5.62 | 0 | 0 |
Carbohydrates (% in lignin sample) | 10.5 | 0.8 | 3 |
S (% in lignin sample) | 1.01 | 1.00 | 0.78 |
* Calculated according to protein = 6.25 × N.
Considering the level of impurities in enzymatic lignin, the lignin yield obtained by the acetic acid–ZnCl2 procedure (62%) is not significantly lower. This represents one of the advantages of this latter extraction technique compared to conventional acidolysis.
The lignin samples have also been characterized by 13C NMR. This technique makes it possible to quantitatively measure the main functional groups in lignin. The values have been corrected for the proteins and carbohydrates present as impurities. One way to express the results is to give the number of groups per aromatic ring in lignin (Table 2). These measures have been performed on the acetylated samples. The number of aliphatic alcohol groups in enzymatic lignin is higher than those observed in acidolysis lignin. Although some corrections for impurities have been made, part of the difference may be explained by the presence of carbohydrates in enzymatic lignin. On the contrary the number of phenolic groups is higher in the acidolysis lignins. However, the difference is significant only in the case of the conventional acidolysis procedure. This would suggest that some cleavage of aryl–ether bonds takes place during conventional acidolysis. However, one may argue that in neither procedure the total quantity of lignin is removed from the pulp and that the two lignins might come from different origin in the fibres. In order to confirm the effect of conventional acidolysis on the ether bonds, the enzymatic lignin has been submitted to acidolysis under the conventional conditions and analysed after treatment. Results in Table 3 indicate that new phenols are produced, most likely because of the cleavage of aryl–ether bonds. The lower extent of aryl–ether bond cleavage during acidolysis with ZnCl2 in acetic acid medium is another advantage of this new technique.
Number of some functional groups in lignin samples extracted from a softwood kraft pulp, measured by 13C-NMR after acetylation (values given per aromatic unit)
Enzymatic | Dioxane–water–HCl | Acetic acid–water–ZnCl2 | |
Primary alcohol | 0.47 | 0.33 | 0.36 |
Secondary alcohol | 0.47 | 0.23 | 0.28 |
Free phenol | 0.47 | 0.55 | 0.50 |
Aromatic quaternary carbon | 3.35 | 3.30 | 3.35 |
Methoxyl on aromatic unit | 0.77 | 0.75 | 0.78 |
Effect of conventional acidolysis on enzymatic lignin (values given per aromatic unit)
Enzymatic | Enzymatic followed by dioxane–water–HCl | |
Primary alcohol | 0.47 | 0.33 |
Secondary alcohol | 0.47 | 0.22 |
Free phenol | 0.47 | 0.56 |
Quaternary carbon | 3.35 | 3.35 |
Methoxyl on aromatic unit | 0.77 | 0.76 |
13C NMR of non-acetylated lignin sample was also performed. In the case of the extraction by acetic acid/water–ZnCl2 acidolysis, the spectrum shows a signal at 169.6 ppm corresponding to a CO carbon in acetylated primary alcohol group. A quantitative analysis based on the comparison between the CO and the OCH3 signals indicates that the extracted lignin possesses an average of 0.2 acetyl group per aromatic ring. The fact that partial acetylation takes place during lignin extraction has to be taken into account, but is not going to cause any problem for the determination of lignin chemical structure.
1-(4-hydroxy-3-methoxyphenyl)-2-(2′-methoxyphenoxy)-propanol-1 has been submitted to the extraction conditions prevailing in the three methods used above in an attempt to assess their effect on aryl–ether linkage of the β-O-4 type. The results are given in Table 4, in which the conversion yields are reported. It is shown that the conventional acidolysis is the most aggressive medium towards β-O-4 linkages. The acetic acid–water–ZnCl2 gives intermediate degradation results. Therefore these results are in accordance with the observations made by 13C NMR.
Cleavage of the aryl–ether linkage in 1-(4-hydroxy-3-methoxyphenyl)-2-(2′-methoxyphenoxy)-propanol-1 under the lignin extraction conditions
Applied treatment | Conversion yield (%) |
Acetic acid–water–ZnCl2 | 18 |
Dioxane–water–HCl | 70 |
Enzymatic | 0 |
4 Conclusion
In conclusion, this study established that conventional acidolysis not only does not extract lignin from kraft pulp in a good yield, but also causes some cleavage of aryl–ether linkages. The use of an acetic acid–water–ZnCl2 medium appeared more suitable for extracting lignin from pulps, since aryl–ether cleavage was less and extraction yield was higher. The resulting partial acetylation of primary alcoholic groups has to be regarded as a non-negative point when considering further analytical characterisation, such as size-exclusion chromatography or 13C NMR. Other applications of the acetic acid–water–ZnCl2 medium are already envisaged, such as purification treatment for enzymatic lignin contaminated with proteins and carbohydrates.
☆ Many thanks to Bernard Monties for the enthusiasm he has been able to transmit to us and for his immense knowledge of the state-of-the art in wood chemistry, from which we all benefited.