1 Introduction
Abiotic stress conditions cause extensive losses to agricultural production worldwide and these have been the subjects of intense research [1]. Water deficits are one of the major abiotic stresses, which adversely affect the crop growth and yield. Coping with water deficit stress is a global issue to ensure survival of agricultural crops and sustainable food production. Conventional plant breeding attempts have changed over to use physiological selection criteria since they are time consuming and rely on present genetic variability [2]. Currently, protection of plant through application of plant growth regulators (PGR) attracts more attention. PGR play an important role in crop production and are being increasingly used to manipulate plant growth and yield [3].
Triazoles form the active ingredient of fungicides (propoconazole, penconazole, epoxiconazole) and growth regulators (paclobutrazol, triadimefon) commonly used within the horticultural, agricultural and forestry industries. Triazole compounds are new synthetic plant growth regulators that act as antigibberellins and known to inhibit shoot growth in plants [4]. Triazoles have been called plant multiprotectants because of their ability to induce tolerance to environmental and chemical stresses in plants [5]. Protection of plants from apparently unrelated stress by triazole is mediated by a reduction in free radical damage and increase in antioxidant potential [6,7]. Triazoles affect the isoprenoid pathway and alter the levels of certain plant hormones by inhibiting gibberellin synthesis, reducing ethylene evolution and increasing cytokinin levels [8]. Triazole treated plants have a more efficient free-radical scavenging system that enables them to detoxify active oxygen [9]. We have reported previously some of the morphological and physiological changes associated with triazole treatment in various plants, include the inhibition of plant growth, decreased internodal elongation, increased chlorophyll levels, enlarged chloroplasts, thicker leaf tissue, increased root to shoot ratio, increased antioxidant potentials and an enhancement in alkaloid production [3,10–13].
For the past several years, several scales of physiology have been applied to study responses to water deficit stress tolerance mechanisms and methods to overcome drought and salt stresses [14–16]. However, little information is gained about the physiological basis in terms of ABA accumulation and nucleic acid contents under drought stress and amelioration of drought by the application of PGR in medicinal plants. It seems necessary to do research related to the correlation between medicinal plants and drought stress for the increasing need of medicinal plants. So it seems valuable, to test the important medicinal plants for their drought tolerance capacity. Catharanthus roseus (L.) G. Don. (Family: Apocynaceae) is one of the highly exploited and studied medicinal plants. This plant contains alkaloids, which are valuable source of antitumour agents like vinblastine and vincristine used in chemotherapy of leukemia and in the treatment of Hodgkin's disease, and also a popular ornamental plant [13,17]. C. roseus is classed as a glycophyte. Despite the relative great number of reports on the medicinal aspects of C. roseus plants [11,17–25] there are only a few attempts to explain the physiological basis of stress effects [25–29]. To the best of our knowledge, no information on the physiological response in terms of growth hormones and nucleic acid contents of C. roseus to drought and TDM application is available. The purpose of this study was to provide additional information in the variations in the hormone levels, nucleic acid content and ATPase and protease activities of C. roseus under individual and combined drought and TDM treatments.
2 Materials and methods
2.1 Plant cultivation, drought stress induction and TDM treatment
Seeds of Catharanthus roseus (L.) G. Don. were collected from Department of Horticulture, Annamalai University, Tamil Nadu, India. The experiments were conducted at the Botanical Garden and Stress Physiology Laboratory, Department of Botany, Annamalai University.
The seeds were surface sterilized with 0.2 percent HgCl2 solution for 5 min with frequent shaking and then thoroughly washed with deionised water. Seeds were sown in plastic pots (300 mm diameter) filled with 3 kg of soil mixture containing red soil, sand and farmyard manure (FYM) at 1:1:1 ratio. Four seeds were sown per pot and the pots were watered to the field capacity with deionized water up to 20 days after sowing (DAS). After 30 days, the plants were grouped and withhold water supply for 5, 10, 15 and 20 days (days interval drought (DID) [1] for three different growth periods. An equal number of plants, 15 mg l−1 were given TDM to test the ameliorating effect of TDM on drought stress. Plants were uprooted randomly on 26, 31, 36 and 41 DAS and used for estimating the ABA level, DNA and RNA contents.
In the preliminary experiments TDM treatment 5, 10, 15, 20 and 25 mg l−1 concentration were tried in combination with drought of which 15 mg l−1 was found to increase the dry weight more or less the level of control and the higher concentration slightly decreased the dry weight. Hence, 15 mg l−1 of TDM concentration was used to ameliorate the drought stress. The same concentration was used to treat the unstressed plants to determine the effect of TDM on the unstressed C. roseus plants.
2.2 Estimation of ABA content
ABA was extracted and purified following the method of Ciha et al. [30] with small modification. Fifty grams of fresh plant material was frozen in liquid nitrogen and immediately extracted with 80% methanol and the extract was stored at 4 °C for 24 h, later the extract was filtered through two layers of cheese cloth and the filterate was centrifuged at 1500 g for 20 min. The methanol was removed from the supernatant in vacuum at 35 °C. The supernatant was adjusted to pH 2.5 with 0.1 N HCl and partitioned with hexane (1:1) thrice and the aqueous phase was saved and the pH was adjusted to 9 using 0.1 N KOH and partitioned against methylene chloride (1:1) thrice and the aqueous phase was saved and this was again adjusted to pH 2.5 and partitioned with n-butanol thrice and the butanol fraction was saved and evaporated to dryness in a film flash evaporator and the dried fraction was eluted with 2 ml of methanol. The extract was purified using TLC technique and the corresponding region of authentic ABA was eluted with 2 ml of methanol and used for the quantitative analysis of abscisic acid through HPLC.
The methanolic extract was analysed with HPLC (Shimatzu model) using UV detector at 254 nm. The chromatography was done with methanol water (60:40) as solvent with the flow rate of 0–5 ml/min. In a C18 column of 14 cm × 4.6 mm diameter, quantification was done considering specific area of the peak comparing the retention time of the standard abscisic acid sample. The ABA standard was obtained from Sigma, USA. The results were expressed in nano gram−1 fresh weight.
2.3 Estimation of nucleic acid contents
Ribonucleic acid (RNA) and deoxyribo nucleic acid (DNA) were extracted and estimated according to the method of Nieman and Paulson [31]. Five hundred milligrams of fresh material was ground in a mortar and pestle with 5 ml of cold methanol at 4 °C and centrifuged at 800 g for 15 min. The supernatant was discarded and the residue was repeatedly washed with cold methanol to remove pigments and alcohol soluble phosphorus. To the residue, 5 ml of 5% ice cooled TCA was added and the contents were stirred thoroughly with a glass rod and centrifuged at 800 rpm for 15 min. The supernatant thus obtained was discarded. The residue was re-extracted with 5% cold TCA and centrifuged at 800 rpm and the supernatant was discarded to remove acid soluble phosphorus. The resulting residue was washed twice with 10 ml of 90% ethanol, ethanol ether mixture (2:1 v/v) and ether successively centrifuged at 800 rpm and the lipid phosphorus present in the supernatant was discarded for each extraction. The solvent was allowed to boil for 20 min. Then the residue was carefully dried, powdered and used for further extraction of RNA and DNA.
2.3.1 Estimation of RNA
The dried residue was suspended in 5 ml of 0.3 N NaOH and kept at 30 °C for 18 h. The sediment was removed by centrifuging at 5000 rpm and washed once with 5 ml of 0.3 N NaOH and then acidified to pH 1.0 with 15% perchloric acid (PCA) and held at 4 °C for 40 min and then centrifuged again. The supernatant contained RNA fraction. The pellet was then resuspended in 2 ml of water followed by the addition of 2 ml of 1 N PCA. The suspension was held at 4 °C for 20 min, centrifuged and the supernatant was added to the RNA fraction. The pellet was retained. The final RNA fraction was made upto 20 ml with distilled water and the extinction coefficient was measured at 260 nm against distilled water as blank in spectrophotometer. A standard curve was prepared with known amount of yeast RNA for calculation.
2.3.2 Estimation of DNA
The residual powder after RNA extraction containing DNA was suspended in 3 ml of 0.5 N PCA and then heated at 70 °C for 15 min, then centrifuged at 800 rpm at 2 °C and the residue was washed twice with 0.5 N PCA and the supernatant were pooled. Then final DNA extract was made up to 5 ml with 0.5 N PCA. The absorbance of the colourless solution was measured at 260 nm using a spectrophotometer against 0.5 N PCA as blank. A standard curve was prepared with calf thymus DNA for calculating the DNA content.
2.4 Determination of ATPase (Adenosine Triphosphatase, EC 3.6.1.3) activity
ATPase activity was assayed by the method of Evans [32]. Fresh plant material of one gram was placed in a precooled mortar and pestle and ground with 5 ms of 0.1 M Tris HCl buffer at pH 7.5 at 4 °C. The extract was passed through cheesecloth and centrifuged at 17,000 rpm for 10 min. The final volume of the supernatant was made upto 10 ml with 0.1 M Tris HCl buffer and used for assay. The incubation mixture in a total volume of 2.0 ml contained 1.0 ml of 0.1 M Tris HCl buffer (pH 7.5), 0.1 ml of 0.0075 M ATP solution, 0.1 ml of each 0.1 MgCl2 0.1 M Drought, 0.1 M KCl, 0.1 M CaCl2 and 0.1 ml of extract. The incubation was carried out at 37 °C for 20 min and reaction was terminated by the addition of 1.0 ml of 10% TCA. Control tubes received 1.0 ml of 10% TCA solution before the addition of the enzyme extract. The suspension was centrifuged and the inorganic phosphorus content of the supernatant was assayed as described by Jaffe and Galston [33]. The enzyme activity (one unit) was defined as the amount of enzyme liberating one μ mole Pi min−1 mg−1 protein.
2.5 Assay of protease
Protease was extracted and assayed using the method of Prisco et al. [34]. One gram of fresh plant tissue was macerated 10 ml of 0.1 M sodium phosphate buffer (pH 7.6) containing 1% NaCl and 7% PVP. The homogenate was centrifuged. The supernatant was made upto 10 ml with the extraction buffer and used as the enzyme source. One milliliter of enzyme extract was incubated with 5 ml of 1% casein solution in 0.1 M sodium phosphate buffer (pH 6.0) at 30 °C. The reaction was stopped after 30 min by adding 1 ml of 40% TCA. The reaction mixture was centrifuged at 4000 rpm for min. The supernatant was saved to estimate the TCA soluble amino nitrogen released, by the activity of protease. Lee and Takahashi [35] method was followed for estimation of the ammonical nitrogen. Five milliliters of ninhydrin reagent was added to 0.5 ml of supernatant and the tubes were heated in boiling water bath for 12 min with vigorous shaking and they were cooled and measured at 570 nm in a spectrophotometer against a blank prepared with 40% TCA. A standard curve was prepared by using glycine as standard. The enzyme activity was expressed in terms of enzyme units. One enzyme unit is equal to micrograms NH2 released hour−1 mg−1 protein. For all the enzymatic calculations protein was determined by the method of Bradford [36], using bovine serum albumin (BSA, Sigma, USA) as the standard.
2.6 Statistical analysis
Statistical analysis was performed using one way analysis of variance (ANOVA) followed by Duncan's Multiple Range Test (DMRT). The values are mean ± SD for six samples in each group. P values ⩽ 0.05 were considered as significant.
3 Results
3.1 Effect of drought, TDM and their combination on ABA level
Drought treatment increased the ABA level to more than twofold in all parts of the plants. TDM treatment to the drought stressed plants showed the highest ABA level when compared with control and all other treatments. TDM treated plants also showed an increased ABA level when compared with control (Fig. 1). However, it was lower than that of other treatments. The leaf showed a higher ABA level, which was followed by root and stem in all treated plants.
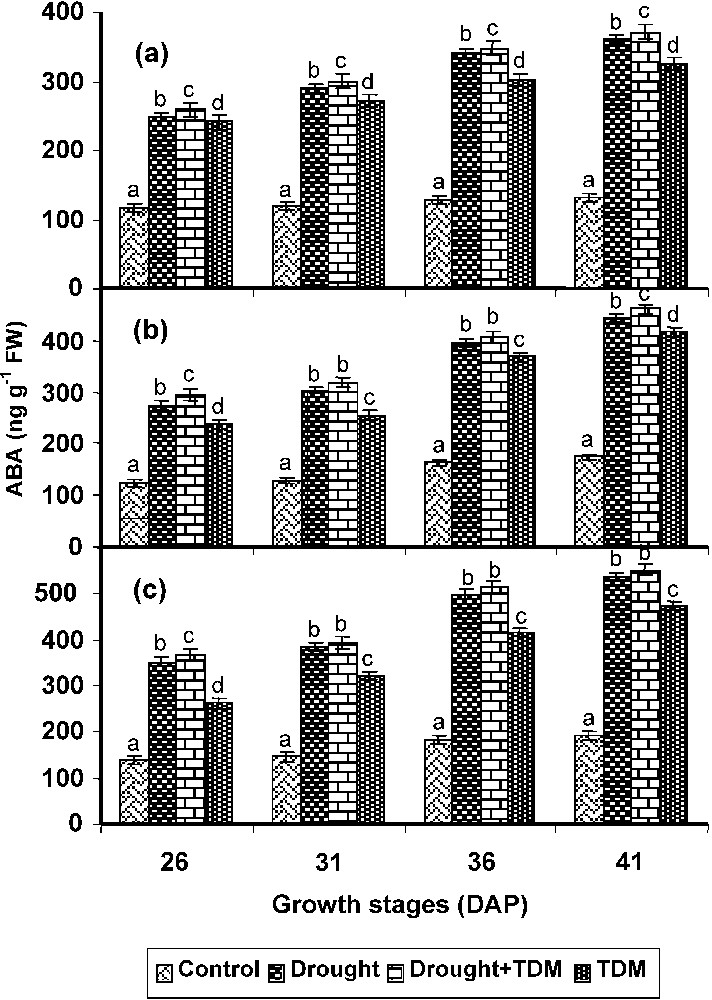
Effect of drought, TDM and their combination on ABA accumulation in Catharanthus roseus. Values are given as mean ± SD of six samples in each group. Bar values are not sharing a common superscript (a, b, c, d) differ significantly at p⩽0.05 (DMRT).
3.2 Effect of drought, TDM and their combination on DNA content
In roots, stem and leaves drought stress caused a decrease in the DNA content when compared with control and other treatments (Fig. 2). Treatment with TDM in combination of drought increased the DNA content to the level of control roots. TDM treated plants also showed an increase in the DNA content was higher when compared with control and other treatments.

Effect of drought, TDM and their combination on DNA content in Catharanthus roseus. Values are given as mean ± SD of six samples in each group. Bar values are not sharing a common superscript (a, b, c, d) differ significantly at p⩽0.05 (DMRT).
3.3 Effect of drought, TDM and their combination on RNA content
Drought stress decreased the RNA content of in all parts of the plants as compared to control. Addition to TDM to the drought stressed plants increased the RNA content to a level even above that of control. TDM treated plants showed an increase in the RNA content of the root when compared to control and other treated plants (Fig. 3).

Effect of drought, TDM and their combination on RNA content in Catharanthus roseus. Values are given as mean ± SD of six samples in each group. Bar values are not sharing a common superscript (a, b, c, d) differ significantly at p⩽0.05 (DMRT).
3.4 Effect of drought, TDM and their combination on ATPase activity
In roots, stem and leaves drought stress caused an increase in the ATPase activity when compared with control and other treatments. Treatment with TDM in combination of drought decreased the ATPase activity to the level of control roots. TDM treated plants also showed decreased ATPase activity when compared with control (Fig. 4).
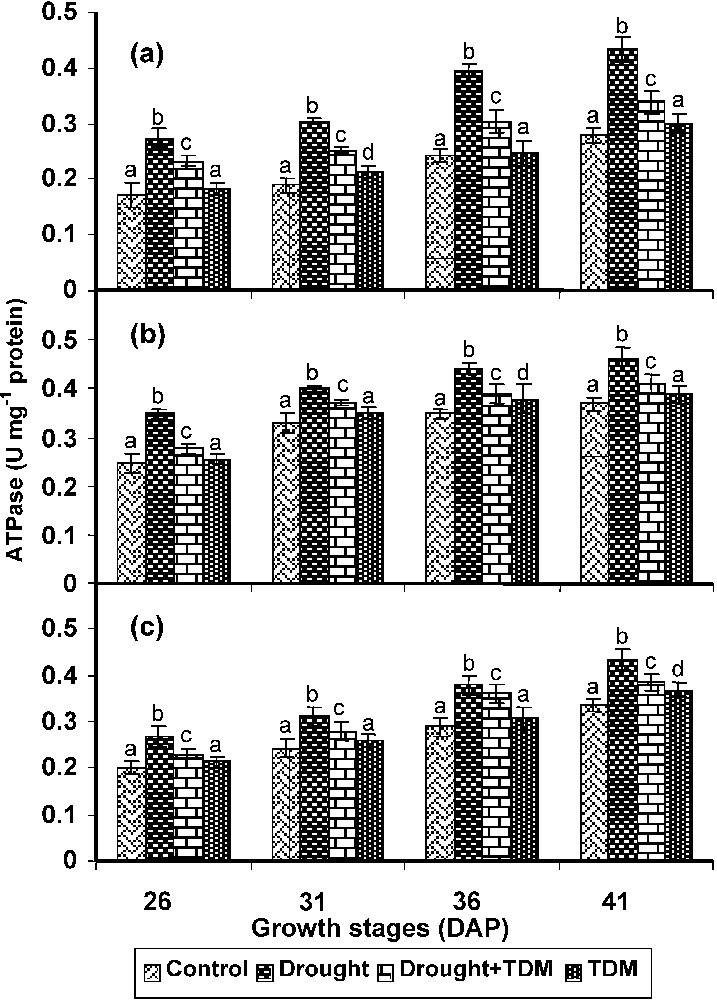
Effect of drought, TDM and their combination on ATPase activity in Catharanthus roseus. Values are given as mean ± SD of six samples in each group. Bar values are not sharing a common superscript (a, b, c, d) differ significantly at p⩽0.05 (DMRT).
3.5 Effect of drought, TDM and their combination on protease activity
In roots, stem and leaves drought stress caused an increase in the protease activity when compared with control and other treatments. Treatment with TDM in combination of drought inhibited the protease activity to the level of control roots. TDM treated plants also showed a decrease in the protease activity when compared with control (Fig. 5).

Effect of drought, TDM and their combination on protease activity in Catharanthus roseus. Values are given as mean ± SD of six samples in each group. Bar values are not sharing a common superscript (a, b, c, d) differ significantly at p⩽0.05 (DMRT).
4 Discussion
Drought treatment increased the ABA level to more than two folds in all parts of the plants TDM treatment to the drought stressed plants showed the highest. Increased ABA level was observed in C. roseus plants under drought stress and the increase is 2.5 times higher than that of control. Drought and cold stress resulted in elevated endogenous ABA levels [37]. ABA has been shown to mediate various responses such as proline accumulation, stomatal closure and shoot growth inhibition [38]. ABA might act as modulator of the response to salt stress mostly through its involvement in the osmotic process [39]. It has been suggested to play a role in the protection against damages provoked by oxidative stress conditions [40]. TDM treatment to the drought stressed C. roseus plants also showed an increased ABA level when compared to control. Similar observation was made in water stressed bean [41]. ABA has been implicated as the initial trigger in the hardening process for various types of plant stresses [37]. TDM treatment to the unstressed plants also increased the ABA content to a large extent when compared with control. An increase in the concentration of ABA was found in the uniconazole treated Phaseolus vulgaris [42]. The increased ABA in the stressed and TDM treated plants can be well correlated with the increased stomatal resistance in C. roseus plants. ABA also induced protein synthesis and the synthesis of new mRNAs in the wilted vegetative tissues [43]. The increased nucleic acid and protein content can be correlated with the increased ABA content.
In roots, stem and leaves drought stress caused a decrease in the DNA content when compared with control and other treatments. Treatment with TDM in combination of drought increased the DNA content to the level of control roots. Drought stress decreased the RNA content of in all parts of the plants as compared to control. Addition to TDM to the drought stressed plants increased the RNA content to a level even above that of control. Drought stress lowered the DNA and RNA contents to a large extent in the C. roseus plants when compared to control and other treated plants. Reduction in nucleic acid content was observed in the salt stressed Vicia faba [44]. The reduction may be due to the increased activities of DNase and RNase as reported by Tewari and Singh [45]. The decreased DNA and RNA content can be well correlated with the reduction in dry weight of the drought stressed plants and it also can be correlated with the reduction in number of cells per unit area in the leaves of the drought stressed C. roseus plants. TDM treatment to the drought stressed and unstressed plants increased the nucleic acid content to a higher level than that of control. TDM and triadimenol treatments increased the nucleic acid content in the barley leaves [46]. The increased dry weight and number of cells per unit area in the TDM treated stressed and unstressed C. roseus plants can also be positively correlated.
The ATPase activity was found to be increased in the drought stressed plants and its activity was very high in the roots when compared to other organs. A similar result was made in the abiotic stresses like salt stress in Vigna unigulata [47]. It has been suggested that H+-ATPase play major role in the osmoregulation under saline conditions [48]. The increase in ATPase activity will increase the hydrolysis of ATP and produce more energy to absorb water and other minerals against the concentration gradient through the drought-injured membrane of the root system in the drought stressed plants.
TDM treatment to the drought stressed and unstressed plants decreased the ATPase activity when compared with drought stressed plants. However, it was higher than that of control. Penconazole and Flusilazol inhibited the ATPase activity with increase in concentration of these triazole compounds in Acerpseudoplatanus [49]. The TDM treated Picea glauca needles showed an inhibited plasma membrane ATPase activity with altered composition of plasma membrane, which led to the maintenance of higher water potential and lower electrolyte leakage under osmotic stress [50]. Maintenance of membrane integrity with TDM treatment might have lead to a lower ATPase activity in the drought stressed C. roseus.
An increased activity of the protease was found in all stages of growth in C. roseus under drought stress. Increased activity of protease was reported in mulberry plants [51] under stress and our results support the observation of the above authors. The increased protease activity with decreased protein content and increased aminoacid and proline content can be well correlated. TDM treatment to the drought stressed and unstressed C. roseus plants lowered the protease activity when compared with drought stressed plants. However, it was higher than control.
5 Conclusion
Water stress after planting is generally recognised as a major factor resulting in death of newly transplanted plants. Results of this investigation indicate applications of triazole derivatives, as a soil drench induces a suite of physiological adaptations that confer a greater degree of drought tolerance and aid in the recovery from drought induced damage. It is suggested that triazole induced protection of C. roseus from damage caused by drought stress is mediated by increased hormonal and enzyme activities.