1 Introduction
Obesity is a multi-factorial disorder, a condition characterized by an excess of body fats. It is more prevalent in the developed countries, but in recent years, it dramatically increased in the developing countries [1]. If it continues by the same rate till 2030, the numbers could rise to a total of 2.16 billion overweight and 1.12 billion obese individuals, or 38% and 20% of the world's adult population, respectively [2]. Energy imbalance reflects a state of positive energy balance (Fig. 1) owing to the genetic predisposition of obesity over recent decades [3]. The genetic aspects of obesity lead to mutations in various genes responsible for controlling appetite and metabolism. Over the past two decades, several strategies have been employed for the identification of genetic determinants of obesity. It includes studies of severe forms of obesity, genome-wide linkage studies (GWLSs) as well as GWAS and analyses of candidate genes. Moreover, based on recent information, about 127 sites in the human genome have been reported to link with the development of obesity through GWAS findings [4]. Since 2005, GWAS approach has led to breakthrough and advancement in the insights into the genetic determinants of common obesity. Single-nucleotide polymorphism (SNP) and animal models suggest genome-wide screens to identify common genetic variants associated with obesity. In this perspective, non-synonymous single-nucleotide polymorphisms (nsSNPs) are used as a potential biomarker to identify the deleterious and neutral effects on protein function. Modern research tools and extensive studies will lead to an understanding of genes and their interaction to cause obesity, which may help with successful interference and treatment [5]. Among these GWAS findings, the first obesity-susceptible locus identified was the FTO (fat mass obesity associated) gene. This gene has the biggest effect on obesity phenotypes risk till date. Each risk allele in FTO was shown to be associated with a 1–1.5 kg increase in body weight and a 20–30% increase in obesity risk [6,7]. Gene–environment interactions (GEI) lead to cause obesity and several metabolic disorders [8]. Epigenetics is the study of heritable changes in gene function without modifications in DNA sequences [9]. Epigenetic changes like DNA methylation, chromatin remodelling, packaging of DNA around nucleosomes and histone modification may also lead to GEI, metabolic disorders and obesity [10–12]. In this review, it is planned to explore the current insights into the molecular genetics of human obesity, ORHC, and energy homeostasis.
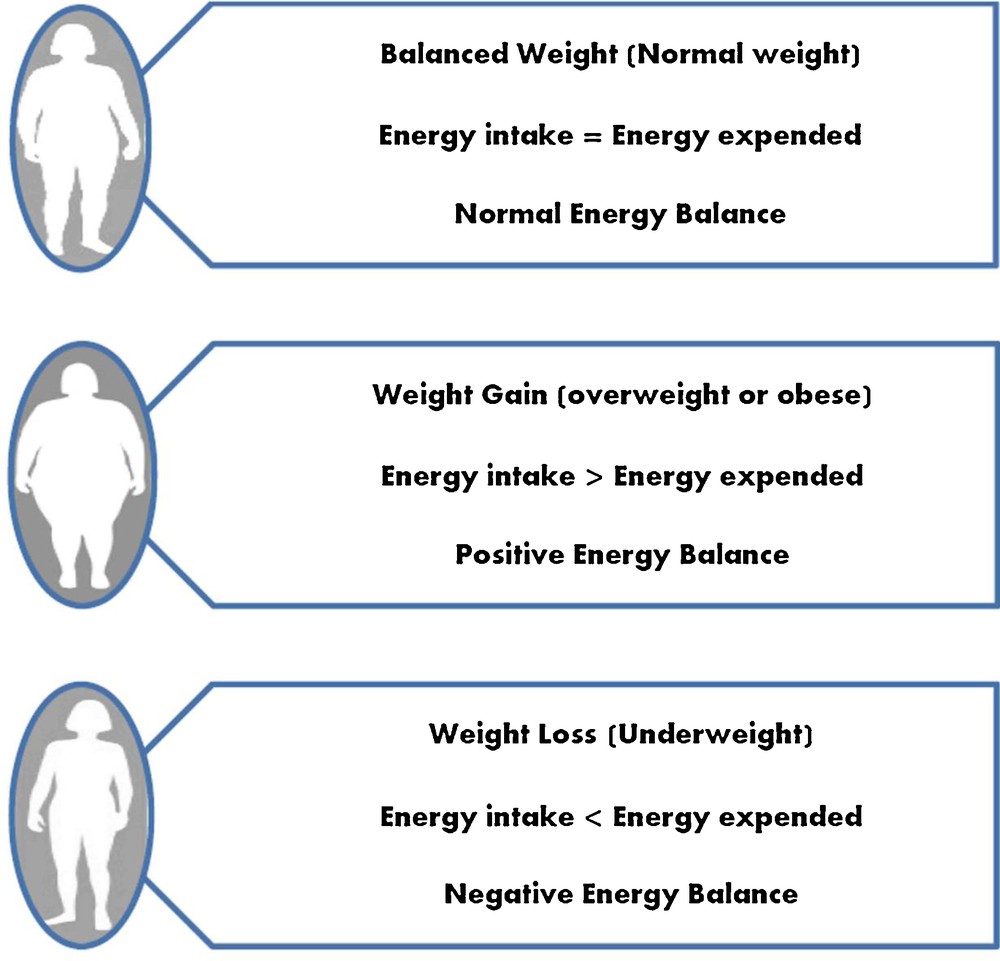
Energy balance: positive energy balance due to overeating, accumulation of fat and weight gain leads to overweight and obesity, whereas negative energy balance due to under-eating, loss of appetite, anorexia nervosa and some digestive diseases lead to underweight.
2 Overview of signalling molecules and regulation of feeding behaviour by central nervous system (CNS)
Two scientists, Hetherington and Ranson, have demonstrated that hypothalamus plays an important role in the regulation of energy metabolism [13]. During 1950s and earlier, it was hypothesized that the intake of food was closely related to the accumulation of fats in the body. But in the late 1970s and early 1990s, several satiety signals like pancreatic glucagon, bombesin and cholecystokinin were identified to check the presence of food [14,15]. The following signalling molecules play an important role in the feeding behaviour regulated by the hypothalamus of CNS:
- • Leptin: it is secreted by white adipose tissues mediated directly through the CNS and inhibits food intake. Leptin conveys information to the hypothalamus regarding the amount of energy stored in adipose tissues and helps in the suppression of appetite and stimulates energy expenditure [16]. It affects metabolic processes like fatty-acid oxidation by activating AMP-activated protein kinase [17]. It also controls the reproductive functions and puberty in females [18,19]. In addition, leptin also affects the immune response by influencing regulatory T (Treg) cell function [20]. With the help of leptin receptor (OB-R), leptin actions are mediated and regulated [21]. In humans, four different OB-R isoforms are there, out of which only the long form of the leptin receptor (OB-Rfl) is able to perform the complete signalling action of OB-R by means of JAK-STAT (Janus kinase-signal transducer and activator of transcription) pathway [22];
- • Insulin: it is secreted by pancreatic β-cells and functions similar as leptin for adiposity signal processing. It stimulates the uptake of glucose and deposition of glycogen in the liver and decreases the release of glucose from the liver. It interacts with specific receptors in the arcuate nucleus of the hypothalamus and reduces food intake and body weight regulation [23]. In one study, it was shown that glycogen metabolism is a mediator in the control of energy homeostasis and might contain glycogen accumulation in adipocytes associated with obesity and insulin resistance. Hypoxia conveys extracellular glucose to glycogen synthesis in human adipocytes [24]. Another study showed that APLN (apelin gene) rs3115757 variant was associated with obesity and insulin resistance [25];
- • NPY (Neuropeptide Y): it is an appetite stimulator, which directly sends the signal to the PVN (Para ventricular nucleus) to increase the feeding behaviour, body weight and adiposity in rats and limited to humans [26,27];
- • AgRP (Agouti related protein): it is also an appetite-stimulatory neurotransmitter antagonist to MC3R (melanocortin-3-receptor) and MC4R (melanocortin-4-receptor) in the hypothalamic nuclei. It controls feeding behaviour via melanocortin receptors (MCRs) and plays a role in weight regulation [28–30];
- • POMC (proopiomelanocortin): it is also an appetite inhibitory molecule that produces α-, β- and γ-MSH (melanocyte-stimulating hormone), and operates mainly through the MC4R and MC3R to inhibit appetite [31];
- • Adiponectin: it is also called gelatin-binding protein and is expressed and secreted from white adipose tissue. It acts as an insulin-sensitizing (it decreases insulin resistance) hormone, whose blood concentrations are reduced in obesity and type-2 diabetes [32]. (vii) Ghrelin: it is lipophilic peptide located in the mucosa of the stomach and its metabolic effects are opposite to those of leptin. It stimulates food intake, improves the use of carbohydrates and decreases fat utilization, enhances gastric motility and acid secretion [33].
3 Melanocortin pathways and energy homeostasis
3.1 Outline of the melanocortin system
The central nervous melanocortin system forms a crucial point for the nutrient-sensing neural networks, directing appetite and metabolic responses [34]. Several reviews showed how molecular pathways, especially central melanocortin pathways, are involved in the control of energy homeostasis and eating habits in the context of obesity [33,35–40]. The melanocortin system plays a crucial role in the regulation of numerous natural functions like energy homeostasis and metabolism, stress responsiveness, sexual activities, pigmentation and inflammation [41] (Table 1). The melanocortin system consists of various types of signalling and biological molecules, namely the following ones:
- • Agonists: it includes adrenocorticotropic hormone (ACTH), cocaine, amphetamine and α-, β-, and γ-MSH produced by cleavage and post-translational processing of POMC polypeptide [40].
- • Antagonists: it includes agouti, AgRP [28–30] and NPY [26,27];
- • MCRs: they belong to the rhodopsin-like family, which is the largest and most intensively studied G-protein coupled receptor (GPCR) family [42]. There were five MCRs melanocortin-1, -2, -3, -4 and -5 receptor (MC-1, -2, -3, -4 and -5 R) identified based on molecular cloning and gene expression pattern in between the years 1992–1994 by different researchers [43–49]. MCRs are the unique members of the GPCR family because all MCRs have different binding affinities to their ligands. This type of uniqueness facilitates different types of biological activities [34];
- • Accessory proteins: MRAP1 and MRAP2, two accessory proteins, were identified for MCRs. These proteins are involved in cell surface targeting and regulate the transport of MCRs towards the cell membrane and ligand induced signalling [49,50]. Out of five MCRs, two MCRs (MC3R and MC4R) are widely expressed in the hypothalamus regions of CNS, and regulate feeding behaviour and energy homeostasis [36,51].
Expression of MCR family in the cell, most ligand binding affinity, and their major functions.
MCR family based on cloning order | Most ligand binding affinity towards | Region of cell expression | Major functions | References |
MC1R | α-, β-MSH and ACTH > γ-MSH | Melanocytes, skin, hair follicles, brain, macrophages, adipocytes, pituitary, placenta, immune cells | Skin pigmentation, melanocyte function, anti-inflammatory | [43,44,54–56] |
MC2R | ACTH | Adrenal gland, adipose tissue, skin | Adrenal gland development and steroidogenesis, neonatal-gluconeogenesis, cell proliferation | [44,57] |
MC3R | γ-, α-MSH and ACTH > β- MSH | Placenta, brain, duodenum, pancreas, heart, kidney, macrophages, stomach, CNS and hypothalamus, adipocytes, limbic region | Energy homeostasis, entrainment to meal intake, anti-inflammatory, food intake | [46,49,54,58,59] |
MC4R | α-, β-MSH and ACTH > γ- MSH | Brain, CNS and hypothalamus, muscle, adipocytes, astrocytes | Energy homeostasis, food intake, anti-inflammatory, drug tolerance, Sexual behavior | [46,60–64] |
MC5R | α- > β-MSH and β-MSH and ACTH > γ- MSH | Exocrine gland, pituitary, ovary, brain, liver, spleen, adipocytes, spleen, B-lymphocytes | Exocrine secretion, fatty acid oxidation, lipid metabolism | [48,65–67] |
The melanocortin signalling system is a complex signalling system due to the involvement of many signalling molecules. The mechanism behind the melanocortin signalling pathway is as follows. Melanocortins are mostly expressed in the pituitary and arcuate nucleus of the hypothalamus and then released into the blood stream through the sympathetic nervous system (SNS) [34]. The central melanocortin signalling pathway regulates by two ways (Fig. 2).
- • Leptin associated signalling: in this particular signalling, leptin is produced by the white adipocytes and, after crossing the blood brain barrier, binds to the specific leptin receptors in the arcuate nucleus of hypothalamus. Leptin processed through two sets of neurons, i.e. POMC/CART (cocaine and amphetamine regulated transcripts) and NPY/AgRP. Leptin enhances the POMC/CART and POMC produces α-, β-, and γ-MSH post-translationally, whereas it inhibits the actions of NPY/AgRP to regulate the feeding behaviour;
- • GPCR associated signalling: in this signalling, POMC peptide exerts its effect through a cyclic adenosine 3’,5’-monophosphate (cAMP)-dependent mechanism when they bind with the Gs family of G-proteins (Gα, Gβ and Gγ) to MCRs. At the cell membrane, MCRs are activated by ligand binding and undergo conformational changes, which trigger a complex intracellular network that translates extracellular signals into biological responses. As part of the GPCRs family, MCRs are functionally coupled with the Gs family of G-proteins and stimulate the cAMP/PKA/ERK1/2 pathway [52]. The Gs family conveys signals from MCRs to adenylate cyclase (AC), which converts the cytoplasmic ATP to cAMP. The increased levels of cAMP act as a second messenger to activate protein kinase A (PKA), which translocates to the nucleus where it phosphorylates the CREB family of transcription factors. Phosphorylated CREBs then induce or inhibit the expression of genes containing CRE (cAMP-responsive elements) consensus sequences in their promoter. Gβγ complex subunit modulates the ERK1/2 pathway. These types of modulations facilitate different kinds of biological functions developed by different MCRs (MC1R, MC2R, MC3R, MC4R and MC5R) (Fig. 2) [35,36,52,53].
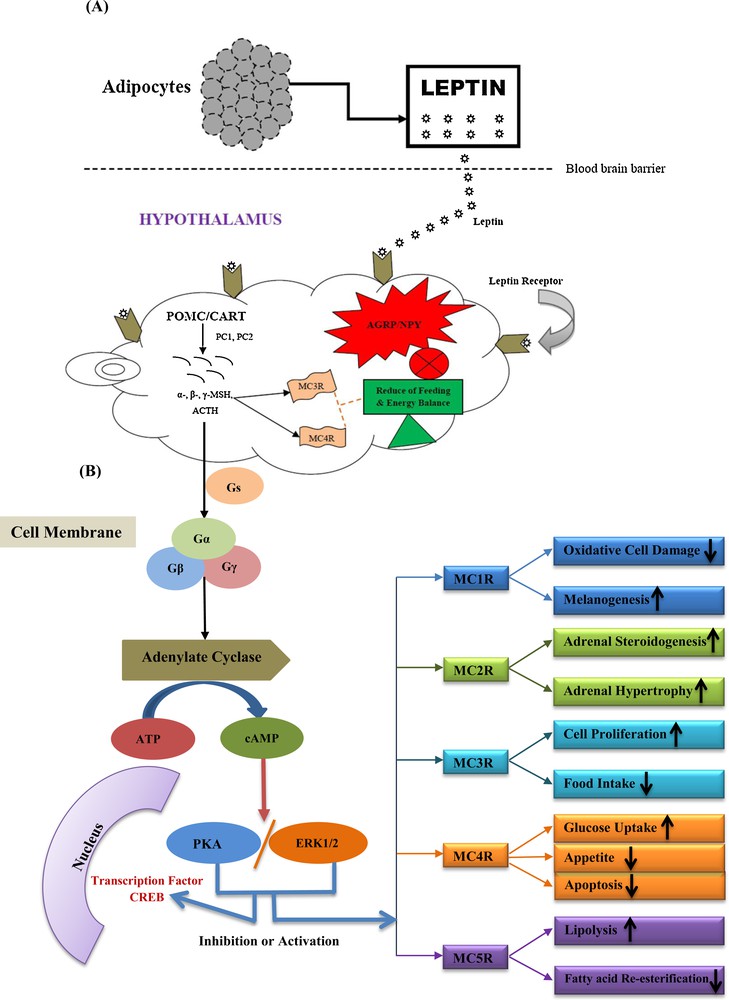
Melanocortin signalling pathway. (A) Leptin-associated. Leptin is secreted by adipocytes; it crosses the blood brain barrier and bind to leptin receptors in the arcuate nucleus of the hypothalamus. Leptin induces the expression of POMC/CART. Enzymes PC1 and PC2 cleave POMC post-translationally into smaller peptides i.e. α- β-, γ-MSH and ACTH. Leptin receptor also inhibits the expression of AGRP/NPY to control of feeding habits. (B) GPCR associated. At the cell membrane, MCRs are activated by ligand binding and undergo conformational changes, which trigger a complex intracellular network that translates extracellular signals into biological responses. As part of the GPCRs family, MCRs are functionally coupled to the Gs family of G-protein (Gα, Gβ and Gγ) and stimulate the cAMP/PKA/ERK1/2 pathway. The Gs family of G-protein (Gα, Gβ and Gγ) transmits the signal from MCRs to adenylate cyclase (AC), which catalyses the conversion of cytoplasmic ATP to cAMP. The increased levels of cAMP act as a second messenger to activate PKA, which translocate to the nucleus where it phosphorylates the CREB family of transcription factors. Phosphorylated CREBs then induce or inhibit the expression of genes containing CRE (cAMP-responsive elements) consensus sequences in their promotor. Gβγ complex subunit modulates the ERK1/2 pathway. These types of modulations facilitate different kinds of biological functions developed by different MCRs (MC1R, MC2R, MC3R, MC4R and MC5R).
3.2 MC3R- and MC4R-mediated energy homeostasis
Energy homeostasis involves using chemical and neuronal signals in the human body to hold the amount of energy flow and regulate the energy intake for the sensation of hunger. It is the balance between energy intake and energy expenditure [68]. MC3R and MC4R are involved in regulating energy homeostasis and food intake behaviour [69,70]. Human linkage and polymorphism studies showed that MC3R plays a crucial role in energy homeostasis [71–73]. Some studies also showed the role of MC3R in food intake behaviour [58,59,70]. The MC4R controls both food intake and energy expenditure [60,74]. The peptides and signalling molecules of central melanocortin pathway are involved in the regulation of energy balance and homeostasis. The central melanocortin pathway regulates energy balance and homeostasis by activating or inhibiting the leptin and its receptor mediated by two subsets of neurons as well as MC3R and MC4R in the arcuate nucleus of hypothalamus. The mechanism as follows (Fig. 3): Adipocytes (adipose tissue forming cells) secrete leptin (a polypeptide), which crosses the blood brain barrier and binds to its specific receptors in two subsets of neurons in the arcuate nucleus of the hypothalamus. The first subset of neurons NPY/AgRP, which increases the appetite known as orexigenic is inhibited by the leptin receptor [55,75,76]. The second subset of neurons POMC/CART, which decreases the appetite known as anorexigenic is enhanced by the leptin receptor [75,76]. In this mechanism POMC/CART processed post-translationally by prohormone convertase 1 and 2 (PC1 and PC2) as a tissue-specific mode and produces neuropeptides [31]. PC1 itself produces ACTH, whereas PC1 and PC2 together produce α-, β-, and γ-MSH. The POMC-derived α-, β-, and γ-MSH activate the signals to the family of MCRs (MC1R to MC5R). The activated MC3R and MC4R play crucial roles in the regulation of energy balance and homeostasis [69,70,76].
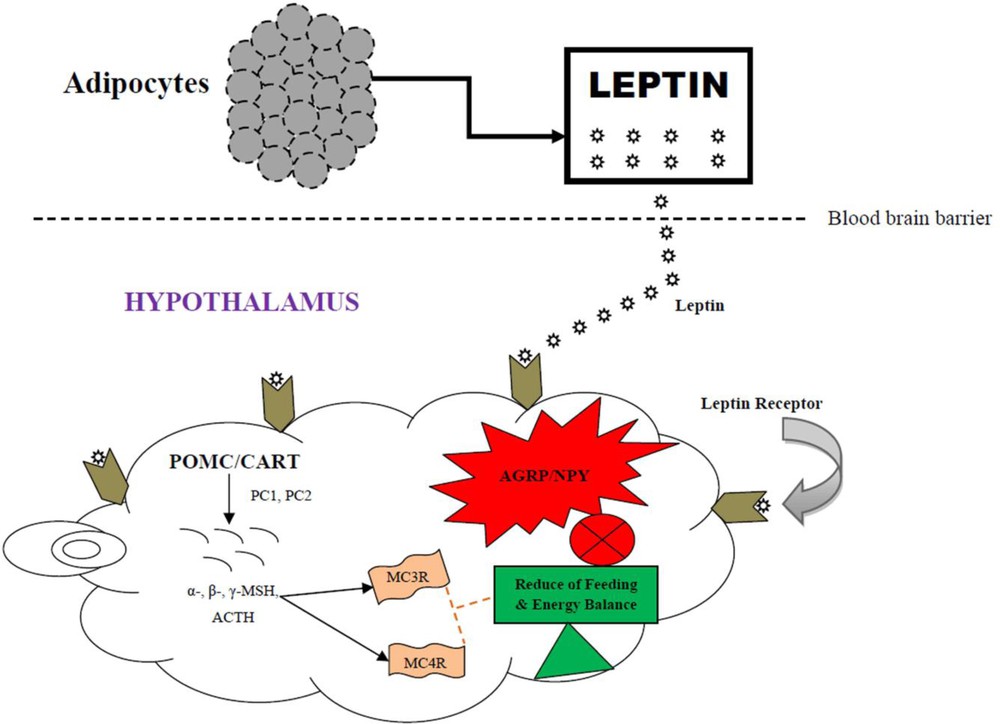
MC3R- and MC4R-mediated energy homeostasis in a nutshell: Adipocytes secrete leptin, which crosses the blood brain barrier and binds to leptin receptors in the hypothalamus. Leptin induces the expression of POMC/CART. Enzymes PC1 and PC2 cleave POMC post-translationally into smaller peptides i.e. α- β-, γ-MSH, and ACTH. These signalling molecules activate the MC3R and MC4R, which in turn helps in the reduction of feeding behaviour and energy balance. In the same pathway, leptin receptor inhibits the expression of AGRP/NPY via MC3R and MC4R, which leads to feeding control and weight regulation.
4 Human ORHC
Obesity is a very common serious health problem and epidemic threat worldwide. It leads to many serious health disorders (Fig. 4). Every year, due to obesity and ORHC, a number of people lose their lives worldwide [77]. In this review, we will discuss some of the disorders related to human obesity, viz. cardiovascular disorders [78,79], hypertension [80,81], type-2 diabetes [82], osteoarthritis [83], late onset of Alzheimer's [84], certain types of cancer [85–88], lower back pain, and lumbar disc degeneration [89,90].
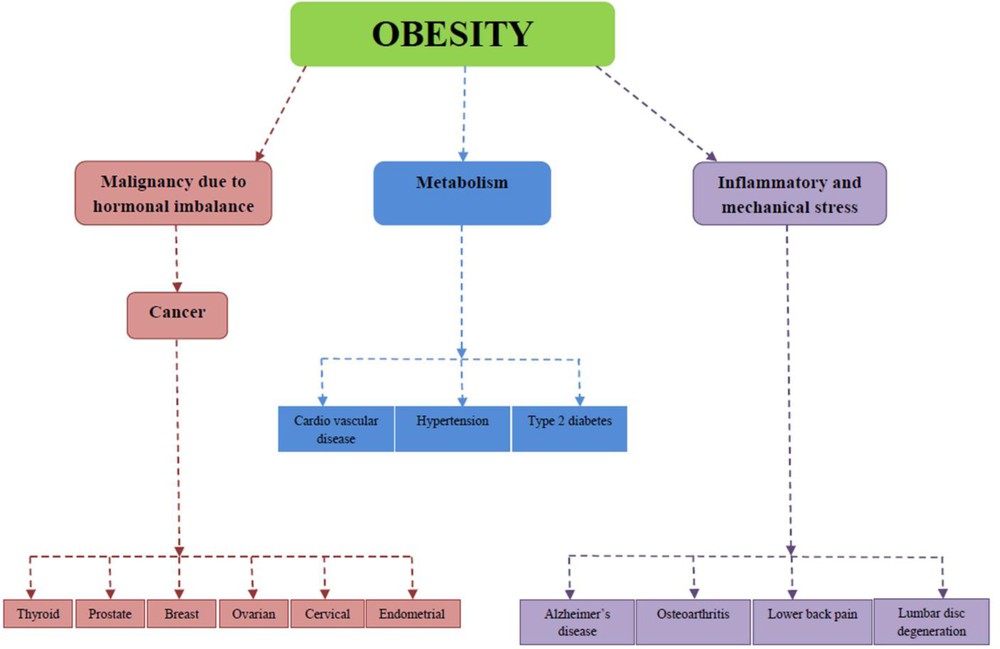
Obesity-associated health complications.
4.1 Cardiovascular disease (CVD)
The American Heart Association underlined that obesity is a major CVD risk factor [91]. Due to obesity, there is a rise in total blood volume and cardiac output, which is caused by enhanced metabolic demand, associated with excess body weight [92]. In the obesity condition, there was a higher right heart filling pressure, cardiac output, systolic pressure, and pulmonary vascular resistance index reported in 43 obese patients examined due to heart failure [93]. According to the American Heart Association (heart disease and stroke statistics–2015 update, National Health and Nutrition Examination Survey, 2009–2012), the prevalence of CVD in adults (≥ 20 years of age) among different age groups was as follows: age 20–39 years (men: 11.9%; women: 10.0%); 40–59 years (men: 40.5%; women: 35.5%); 60–79 years (men: 69.1%; women: 67.9%); 80+ years (men: 84.7%; women: 85.9%) [94]. Several studies have also shown that an increased BMI was significantly associated with men and women, with symptoms of CVD like myocardial infarction (MI), angina, heart failure (HF), and sudden death [95,96]. Romero-Corral et al. (2006) found 250,000 patients with coronary heart disease (CHD) in a large meta-analysis of 40 cohorts. In this study, Romero-Corral has also demonstrated that there was a possible adjusted mortality in the overweight and obese subgroup [97]. In another study, Das et al. (2011) have studied a cohort of 50,149 patients with ST segment elevation myocardial infarction (STEMI), having BMI (30–35 kg/m2) and extreme obesity with lowest mortality [98]. However, Dhoot et al. (2013) have reviewed a cohort of 400,000 patients with STEMI and non-STEMI in 2009 (hospital-based study). Herein, Dhoot (2013) has found a possible adjusted mortality associated even with morbid obesity (BMI ≥ 40 kg/m2; compared with < 40 kg/m2 aggregated) [99].
4.2 Hypertension
Hypertension is also regarded as high blood pressure (BP) (systolic BP ≥ 140 mmHg and/or diastolic BP ≥ 90 mmHg) affecting around 1 billion people globally; WHO (World Health Organization) proposes this will rise to 1.5 billion by 2020 [100]. Obesity is associated with hypertension, as evidenced by several studies [80,81]. It is mainly associated with arterial hypertension where changes in the renal function and structure lead to activation of the SNS, renin-angiotensin system, and sodium retention [80,81,101–103]. Obesity-related hypertension leads to several other complications, like left ventricular hypertrophy, atrial fibrillation, congestive heart failure, coronary artery disease, cerebrovascular disease, atherosclerosis, and renal insufficiency [102–105]. Kannel (1993) has reported that obesity itself causes essential hypertension of 78% and 65% in men and women, respectively, from data from a Framingham cohort [106]. Genetics, genetic variations and polymorphisms in many genes are an important cause of essential hypertension. Padmanabhan (2012) has reviewed SNPs and GWAS studies of monogenic syndrome, loci of hypertension and BP, which would be helpful to understand their genetic basis [107].
4.3 Type-2 diabetes
Obesity also plays an important role in diabetes, especially in type-2 diabetes [108,109]. Due to the links between obesity and type-2 diabetes and their interdependence, a common term coined for both epidemics is “diabesity” [110]. More than 360 million people worldwide are suffering from type-2 diabetes and if the current trends continue, then approximately 10% of the global adult population will be affected by 2030. Type-2 diabetes caused more than 3.5 million deaths in middle-income countries [111,112]. Obesity and type-2 diabetes often occur together and studies found that about 60–90% of patients with type-2 diabetes have been obese [113,114]. Warram (1990) has found that the normal glucose tolerance of the offspring of diabetic parents were with insulin resistance, excessive body weight, and increased risk of type-2 diabetes [115].
4.4 Osteoarthritis
Osteoarthritis is the most common musculoskeletal disorder whose pathogenesis is unclear and the subject of debate. However, a number of risk factors are associated with this disorder, in which obesity or overweight is one of the most prominent risk factors [83,116,117]. Several studies have been confirmed that obesity is associated with hand, knee, and hip osteoarthritis [83,116–119]. Visser et al. (2014) showed that subcutaneous and visceral fat (30% of the total student population) was associated with hand osteoarthritis [83]. In this study, they found that adipose tissue and its products may induce osteoarthritis in the hand. They were also able to explain that visceral fat secretes some bioactive cytokines that have been suggested to act locally in the joint tissues and might be associated with osteoarthritis of the hand on the basis of previous reports [120,121]. Obesity is also associated with knee or hip osteoarthritis. Sowers (2008) has found that BMI and body composition were associated with radiographically defined knee osteoarthritis in women. Herein, Sowers (2008) has demonstrated a total of 41% fat mass associated with knee osteoarthritis in women [119]. In the other study, Holliday (2011) has found a risk of knee osteoarthritis associated with BMI higher in women (adjusted odds ratio 3.23, 95% confidence interval 2.62–3.98, P < 0.001) than in men (adjusted odds ratio 2.20, 95% confidence interval 1.81–2.66, P < 0.001) [118]. He has also been able to found that the risks of hip osteoarthritis were almost similar between men and women (women: adjusted odds ratio 1.69, 95% confidence interval 1.41–2.04, P < 0.001; men: adjusted odds ratio 1.51, 95% confidence interval 1.26–1.80, P < 0.001). Hence, the author has found that women were more prone than men to knee osteoarthritis. Thus, from the above explanation, we can say that obesity is one of the most crucial risk factor for osteoarthritis in men and women.
4.5 Late-onset Alzheimer's disease
Late-onset Alzheimer's disease is the most common form of dementia, which accounts for 70–90% of all cases [122]. But the possible mechanisms linking obesity to late-onset Alzheimer's disease are not yet clear. Central obesity in middle age is associated with an increased risk of dementia, including late-onset Alzheimer's disease [123–125]. According to Luchsinger et al. (2012) central obesity in the elderly age people is related to late-onset Alzheimer's disease. These authors have found, in their adjusted follow-up study of 5734 people aged 65 years and more, that only 145 people had Alzheimer's disease [84]. Among obesity measures, here only the higher waist–hip ratio was strongly and significantly associated with late-onset Alzheimer's disease, rather than BMI and waist circumference (WC). In the measures of central obesity, mainly the waist–hip ratio appears to be a better predictor of cardiovascular results compared to BMI [126]. Thus, the reports of Yusuf (2004) and Luchsinger (2012) showed that the waist–hip ratio was strongly and significantly associated with late-onset Alzheimer's disease.
4.6 Cancer
Obesity has also been shown recently to play a crucial role in the development of some types of cancer, including thyroid cancer [85], prostate cancer [87], female malignancies like breast cancer, endometrial cancer, ovarian cancer, and cervical cancer [86,88]. According to Oberman et al. (2015), obesity and type-2 diabetes favours the development of differentiated thyroid cancer [85]. They carried out a randomized case-control study of normal patients and differentiated thyroid cancer patients from 2005 to 2012. In this study, Oberman et al. (2015) found that obesity was more significantly associated with differentiated thyroid cancer as compared to type-2 diabetes (lesser extent). Here, BMI was a strong prognostic variable for differentiated thyroid cancer. In another study, Agalliu et al. (2015) showed that obesity is strongly related to prostate cancer as compared to the diabetic prostate cancer patients [87]. Prostate cancer is the second foremost cause of lethal cancer in developed countries [127,128]. Agalliu et al. (2015) found there were 25.6% people with obesity and 18.7% people with diabetes in the cohort. In another study, Benedetto et al. (2015) found out that obesity and BMI are related to female malignancies like breast cancer, endometrial cancer, ovarian cancer, and cervical cancer [86]. Here, Benedetto et al. (2015) demonstrated that obesity induces the risk of endometrial, ovarian, and postmenopausal breast cancer, whereas BMI was associated with cervical cancer. Wasserman et al. (2004) has also identified that obesity was associated with the occurrence and the recurrence of breast cancer in postmenopausal women [88]. They presumed that it was possibly because of increased exposure to oestrogen, insulin, and insulin-like growth factors that breast cancer developed in postmenopausal women. They have done a cross-sectional sampling of a total of 301 postmenopausal women with breast cancer (age: 34.5–70.8 years; BMI: 17.8–54.6 kg/m2) [88].
4.7 Lower back pain and lumbar disc degeneration
Obesity is a potential risk factor for low back pain and lumbar disc degeneration [89,90], but this will need more studies, reviews, and successful inferences. Dario et al. (2015) have reported, in a systematic review with meta-analysis, five studies linked with lower back pain and seven with lumbar disc degeneration [89]. Dario et al. (2015) reviewed and demonstrated that the risk of lower back pain was associated with individuals having higher levels of BMI or whose weight was almost twice that of individuals with a lower BMI. A meta-analysis (cross-sectional and cohort study) by Shiri et al. (2010) showed that obesity and overweight were associated with increased prevalence (odds ratio = 1.33, 95%; confidence interval: 1.14, 1.54) of low back pain [90].
5 Molecular genetics of human obesity
Genetic characteristics of obesity lead to mutation in several genes, key for controlling appetite and metabolism. Obesity is determined by both genetic and environmental factors. BMI, waist circumference and waist-to-hip ratio are regarded as commonly used methods to distinguish between overall obesity (measured by BMI) and central adiposity (measured by waist circumference and waist-to-hip ratio). A new study on Chinese population showed the impact of the distribution of fats (subcutaneous and visceral) among obese individuals imaged by magnetic resonance imaging (MRI) technique [129]. According to Wang et al. (2016), GWAS has uncovered a number of variants associated with BMI, waist circumference and waist-to-hip ratio. In this regard, Wang (2016) has genotyped 56 validated variants of BMI, waist circumference, and waist-to-hip ratio in 2958 subjects from China, and carried out a linear regression analysis to determine the association with visceral fat area (VFA) and subcutaneous fat area (SFA) imaged by MRI. From their analyses, Wang (2016) found significant associations with the VFA and the VFA-SFA ratios in all subjects with genetic variations in SNPs, rs671 in ALDH2, rs17782313 near MC4R and rs4846567 near LYPLAL1 gene [129].
A study has been carried out very recently on the Drosophila melanogaster model to identify human metabolic diseases, including obesity, to explain the mechanisms of transgenerational effects of maternal obesity and their impact on offsprings [130]. The genetics of obesity has two forms, i.e. syndromic and non-syndromic (Fig. 5), associated with various genes, genetic and clinical consequences. A new study demonstrated that genetic syndromes were associated with obesity with or without developmental delay [131]. Prader–Willi syndrome, fragile X syndrome, SIM1 deficiency, Bardet–Biedl syndrome, Cohen syndrome and Albright's Hereditary Osteodystrophy (AHO) syndrome are associated with obesity with developmental delay. Moreover, Alstrom syndrome, congenital leptin deficiency, leptin receptor deficiency, POMC deficiency, PC1 deficiency, MC4R deficiency and SH2B1 deficiency are associated with obesity without developmental delay [131]. Henceforth, the identification of a genetic cause of obesity can be useful for genetic counselling and may evaluate the optimal treatment possibilities.
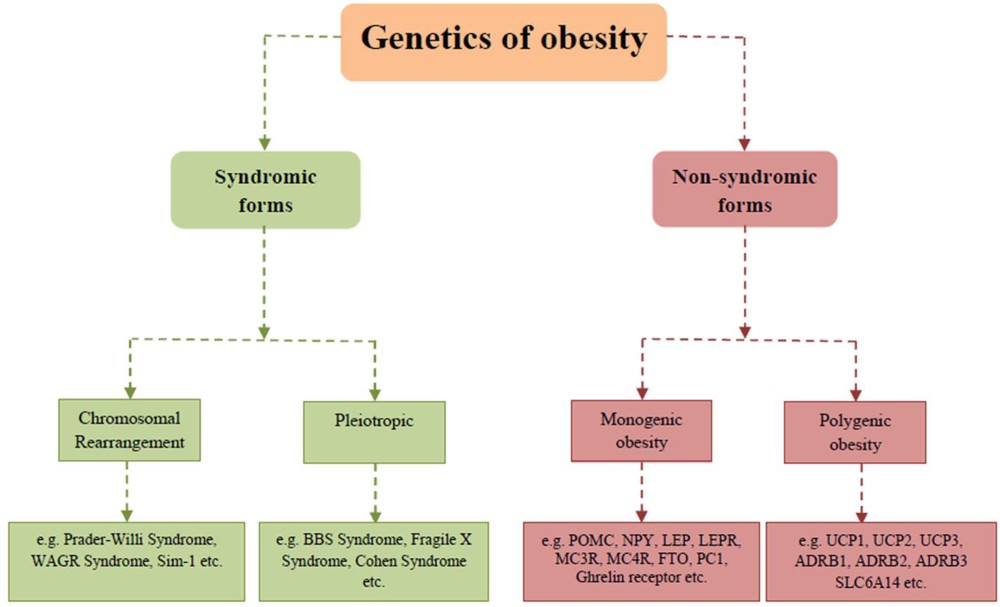
Genetics of obesity: Syndromic and non-syndromic forms of obesity.
Over a few decades, in the course of the advancement of modern research, genetic tools had an impact on the finding of new genetic traits of obesity. In this context, studies of severe forms of obesity, genomewide linkage studies and GWAS helped in the founding of candidate genes. To date, 127 novel loci have been investigated to linked with common forms of obesity in different populations of diverse ethnicities and ages based on GWAS studies [4]. Moreover, SNPs and animal model studies help researchers to find out the genetic variants associated with obesity [5]. The Human Genome Project and the Hap-Map Project are the major achievements that have notably enhanced our knowledge on genetic variations in the human genome. Apart from GWAS-associated studies, new findings explored the interactions between genetic factors, and environmental conditions might accelerate the translation of genetic findings into more successful preventive and therapeutic measures [4,8].
5.1 GWAS approach to find out obesity susceptibility locus
GWAS is a candidate gene association study design where a large number of genetic markers (SNPs) across the entire genome are identified, associated with the phenotypes of interest. Studying populations of different ancestries is globally very beneficial to the identification of genes, and their pathways correspond to more striking targets for prevention, diagnosis, and therapeutic interference in obesity. The majority of the GWAS analyses of obesity and BMI till date have identified in a Caucasian population due to low genetic variation in the genome. GWAS approach helps in the identification of common SNPs that contribute to a relatively low risk (as measured by odds ratio [OR] < 1.5) of rising complex diseases and phenotypes, including obesity-related phenotypes, for instance diabetes and hypertension [132]. A number of obesity-susceptible gene loci have been identified in different populations by the GWAS approach (some examples are given in Table 2).
Examples of obesity-susceptible loci identified by GWAS.
Gene | Chromosomal location | Phenotype | Population studied | References |
FTO | 16q12 | BMI; WC; Fat percentage; extreme obesity | European | [6,7,133,134] |
MC4R | 18q21 | BMI; WC; extreme obesity | European: Indian Asian | [134–136] |
MC3R | 20q13.2-13.3 | Obesity | Caucasian population, Hispanic population | [137,138] |
SLC6A14 | Xq23 | Obesity | Finish, French | [139–141] |
POMC | 2p23.3 | BMI | European | [142–144] |
BDNF | 11p4 | BMI; extreme obesity | European | [143,145,146] |
TMEM18 | 2p25 | BMI; extreme obesity | European | [146–148] |
NEGR1 | 1p31 | BMI | European | [143,146,148] |
PCSK1 | 5q15-q21 | BMI | East Asian | [142] |
GNPDA2 | 4p12 | BMI | European | [143,148] |
MAP2K5 | 15q23 | BMI | European | [142,143] |
SEC16B | 1q25 | BMI | European | [143,146] |
5.2 Non-syndromic forms of human obesity
5.2.1 Monogenic obesity
Monogenic obesity is caused by a single gene mutation. The cases are rare, very severe, generally of early onset, and are found in childhood [149]. These types of obesity involved body weight regulation and stability by energy intake, energy expenditure (energy homeostasis and energy balance) and adipogenesis. The mutation in the following genes leads to monogenic obesity.
5.2.1.1 POMC and PC1 gene mutations
POMC is an appetite inhibitory gene, which produces α-, β-, γ-MSH and ACTH controlled through MC3R and MC4R, inhibiting appetite [31]. POMC and ACTH defects in obese children since birth may correspond to an acute adrenal cortex deficiency. Two studies have identified homozygous and heterozygous children with POMC gene mutation and ACTH defects, leading to the genetic characteristics like resting metabolic rate, mild hypothyroidism, ginger and red hair, and pale skin due to lack of α-MSH [150,151]. In a study, 475 subjects were genotyped to search for Arg236Gly mutation of POMC gene in obese and non-obese subjects. In this particular study, there was only one obese subject with heterozygosity for Arg236Gly (0.4%), and no control subject with the mutation identified [152].
PC1 helps in the post-translational processing of POMC, and displays severe early-onset obesity with postprandial hypoglycaemia, hypogonadism, hypocortisolaemia, and, to a lesser extent, of small intestinal dysfunction [153–155]. Benzinou (2008) showed that three tag nsSNPs – rs6232 (Asn221Asp), rs6234 (Gln665Glu) and rs6235 (Ser690Thr) – were associated with adult and child obesity in a total of 13,659 individuals of European ancestry [153].
5.2.1.2 Leptin and leptin receptor gene mutations
Leptin and leptin receptor mainly expressed by white adipose tissues and a regulator of fat metabolism and energy intake. In the arcuate nucleus of hypothalamus, leptin binds to its receptor and inhibits the NPY/AgRP pathway. Leptin increases the appetite, and this is the reason why it is called “orexigenic” [55,75,76]. In addition, leptin and leptin receptor activate POMC/CART, which decreases appetite; they are called “anorexigenic” [75,76,156,157]. It is the product of the human homolog of the mouse obese gene that causes hereditary homozygous mutation in mice. The role of leptin and leptin receptor gene in the uniqueness of human obesity still has to be defined. Besides, Farooqi (2005) has demonstrated and showed inherited human leptin deficiency in the subjects with severe early-onset obesity (8 years and 86 kg, or 2 years and 29 kg) due to a frame-shift mutation in the homozygous obese gene (deletion of G133) and truncated protein [150]. Many families have been identified as the involvement of leptin and leptin receptor gene mutations [149,158–160]. Recently, two polymorphisms of leptin receptor gene, rs1137100 (Lys109Arg) and rs1137101 (Gln223Arg) were reported in Yogyakarta (Indonesia). There were a total of 110 subjects containing 55 obese and 55 healthy adult subjects as controls. BMI, waist circumference and leptin level in the obese group were significantly higher than in the control group. The frequency of Arg103Arg homozygote in obese group was higher than in the control group, while Gln223Gln homozygote in obese group was lower than in the control group. Thereby, polymorphism of rs1137100 (Lys109Arg) and rs1137101 (Gln223Arg) were associated with obesity and leptin level [161].
5.2.1.3 NPY gene mutations
NPY is an appetite stimulator, a neurotransmitter that plays a crucial role in the hypothalamic control of energy balance. Although there are extensive evidences of the NPY gene present in energy regulation in the mouse model, while evidences in humans are limited. The association of NPY gene variants with obesity has been confirmed by many studies. Bray (2000) has identified common human sequence variants (−880I/D) in the regulatory and coding sequence of NPY gene associated with obesity in a Mexican-American population of Texas [162]. In addition, a number of studies showed that SNP rs16139 (Leu7Pro) has been strongly associated with obesity and related complications like increased BMI [163], hypertension [164], high plasma LDL-c, serum triglycerides among school children [165,166], obesity [167], and coronary artery disease [168].
5.2.1.4 Ghrelin receptor gene mutation
Ghrelin receptor is a lipophilic peptide located in the mucosa of the stomach. It stimulates various biological functions and its metabolic effect is opposite to that of the leptin. Ghrelin receptor regulates various physiological processes, including food intake and energy expenditure, glucose metabolism, cardiovascular functions, gastric acid secretion and motility, and immune function. Several human genetic studies conducted in populations originated from Europe, Africa, South America, and East Asia identified rare mutations and single nucleotide polymorphisms that might be associated with human obesity and short stature [169]. A mutation in this gene leads to obesity in humans during puberty and very short height. In this context, two SNPs (Ala204Glu and Phe279Leu) were reported, which were associated with human obesity as well as with very short height [170].
5.2.1.5 MC3R gene mutation
This gene has single exon and located on chromosome No. 20 (20q13.2–q13.3). It belongs to the rhodopsin-like GPCR family and mainly expressed in hypothalamus, brain, placenta, stomach, duodenum, pancreas, kidney as well as macrophages [171]. Human linkage and polymorphism studies showed that MC3R plays a crucial role in energy homeostasis [71–73]. Some studies also showed the role of MC3R in food intake behaviour [58,59,70]. It also acts as an anti-inflammatory response [54]. According to the human gene mutation database (HGMD; http://www.hgmd.cf.ac.uk/ac/), 15 missense mutations out of 18 of the total mutations are reported for this gene. Lee (2002) has firstly reported MC3R gene mutation associated with human obesity. The author has found a novel mutation Ile183Asn (T548A) present in heterozygosity in a 13-year-old obese girl (fat, 49%) and her father (fat, 30%) of Indian origin in Singapore [172]. In this particular study, the whole coding region of MC3R gene was screened and sequenced in 41 unrelated obese children and 121 DNA samples from non-obese individuals, and analysed by allele specific PCR for novel sequence variants Ile183Asn (T548A). Zegers (2011) has found three novel heterozygous, nonsynonymous coding mutations (Leu299Val, Asn128Ser and Val211Ile) in obese children [173]. Several reports on the functional characterization of Ile335Ser variants were identified from morbidly obese subjects in the USA, Italy, and Poland [174–177]. Yang (2012) has identified nine novel naturally occurring MC3R mutations (Ser69Cys, Ala70Thr, Ile87Thr, Met134Ile, Leu249Val, Ala260Val, Thr280Ser, Met275Thr and Leu297Val) in Singaporean patients [171]. Yang (2015) showed that the naturally occurring mutation Thr280Ser has reduced cell surface expression and dramatic functional defects in both ligand binding and intracellular cAMP generation [178].
5.2.1.6 MC4R gene mutation
MC4R is one of the most important MCR in the melanocortin pathway. It is also a GPCR family with seven transmembrane domains, expressed in hypothalamus, brain, muscle, adipocytes, astrocytes and helps in energy homeostasis, food intake, anti-inflammatory, drug tolerance, and sexual behaviour [60–64,179]. A mutation in the MC4R gene leads to obesity, firstly identified as frame-shift mutation in 1998 [180,181]. According to HGMD (http://www.hgmd.cf.ac.uk/ac/), 118 missense mutations out of 139 of the total mutations are reported for this gene. GWAS studies showed that SNPs near in this gene are associated with increased risk of severe early-onset obesity [135,136,182]. SNP (rs1778231) and SNP (rs129070134) have been reported in association with obesity/BMI in Europeans and Indian Asians respectively [136]. Pathogenicity of MC4R mutations is more common in northern European populations than in Mediterranean or Asian populations [183]. The severity of MC4R mutations in homozygous obese patients is more than in heterozygous obese patients [184]. Variant Val103Ile is a common polymorphism associated with abdominal obesity having a minor allele frequency of 2–4% in heterozygotes (Val103Ile) as compared to homozygotes (Val103Val) [185]. According to Lv et al. (2015), SNP (rs17782313) was associated with obesity and obesity-related traits (P < 0.005), with odds ratios ranging from 1.22 to 2.15 in the Chinese children (n = 2977, 853 obese and 2124 controls, aged 7–17 years) [186]. An additive interaction with salt preference was also found in SNP (rs17782313) on obesity [186]. In another study, Khalilitehrani (2015) has also identified SNP (rs17782313) associated with high-energy intake (P < 0.001), and low carbohydrate and protein intakes (P < 0.001 and P < 0.01, respectively) in Iranian adults (n = 400, age > 22 years) [187]. In addition, the significant association between variant rs17782313 and fat intake disappeared after adjusting for energy [187]. Thereby, a novel association was suggested between this polymorphism (rs17782313) and dietary intake [186,187].
5.2.1.7 FTO gene mutation
FTO is a large gene containing nine exons having more than 400 kb. This gene is widely expressed in foetal and adult tissues in humans, mice with highest expression in the brain [7]. In the brain, the FTO gene expression is relatively high in hypothalamic nuclei, where the control of energy homeostasis is mediated [7]. The mouse model has also evidenced the fact that the FTO gene plays an important role in controlling food intake, energy homeostasis, and energy expenditure [188,189]. GWAS studies have suggested that FTO gene is the most significant candidate gene contributing to obesity in both children and adults (Table 2). This gene affects together adults and child obesity as well as type-2 diabetes in European and Asian populations [190,191]. According to Claussnitzer et al. (2015), FTO gene variant rs1421085 (T-C), which is associated with obesity, can interrupt ARID5B repressor binding, which results in depression of IRX3 and IRX5 during early adipocyte differentiation. This process may direct to a cell-autonomous shift from white adipocyte browning and thermogenesis to lipid storage, enhanced fat stores, and weight gain [192]. A study of 306 women with BMI (18–53 kg/m2) of the FTO gene variant rs9939609 is related to fat cell lipolysis and adipose tissue gene expression may help in body weight regulation [193]. According to Shahid et al. (2013), rs9939609 variant is associated with BMI and risk of obesity in adult Pakistani females with increased levels of fasting blood glucose and plasma insulin [194]. Our previous in silico study of FTO gene revealed that there were four nsSNPs, i.e. rs139000284, rs139577103, rs368490949, and rs373076420, found to be significantly deleterious with PolyPhen 2.0 and PANTHER tools, which might play a crucial role in the development of human obesity [195]. Recently, Phani (2016) has demonstrated that FTO gene variant (rs9939609) was associated with obesity-mediated type-2 diabetes susceptibility in an Indian population (n = 1036, cases (type-2 diabetic + obese) = 518, controls = 518) [196]. Phani (2016) has stratified the type-2 diabetes subjects according to obesity status and found that the association with type 2 diabetes was significant only in the obese diabetic group (OR = 1.27, 95% CI = 1.03–1.54, P = 0.02) as opposed to the non-obese group with type-2 diabetes (OR = 1.18, 95% CI = 0.35–3.94, P = 1.0) [196]. In another study, FTO gene variant (rs9939609) AA genotype was significantly associated with pre-pregnancy, excessive weight in Brazil [197]. Illangasekera (2016) has found an association of the FTO gene variant (rs9939609) risk genotypes (AA + AT) with obesity (OR = 1.69, 95% CI = 1.11–2.56, P = 0.01) in urban and rural population (n = 535, aged 18–70 years) of Sri Lanka [198].
5.2.2 Polygenic obesity
Polygenic obesity is also called common obesity. Monogenic obesity studies have given significant insights into the biology of obesity, mostly the severity of obesity phenotypes. Hypothetically, the variants of polygenic obesity differ specifically from one obese individual to another [199, 200]. Due to this reason, the study of polygenic obesity is more complex than monogenic obesity. Polygenic obesity does not play a significant role in obesity characteristics as compared to monogenic obesity. However, after the discovery of new technological advents like GWAS, GWLSs, candidate gene association studies played a major role in identifying the polygenic basis of obesity. Different genes and their mutations are associated with the polygenic form of obesity as given below.
5.2.2.1 β-adrenergic receptor family gene mutation
β-adrenergic receptor is a class of the GPCR family and a target of catecholamines, especially epinephrine (adrenaline) through SNS. This receptor plays an important role in physical fitness–inflammation association among the obese individuals by regulating the levels of inflammatory cytokines [201]. β-Adrenergic receptor has three classes: β1-adrenergic receptor, β2-adrenergic receptor and β3-adrenergic receptor. A number of signalling molecules are involved in the β-adrenergic receptor activation, which helps us to understand the β-adrenergic receptor family gene mutation and energy expenditure (Fig. 6).
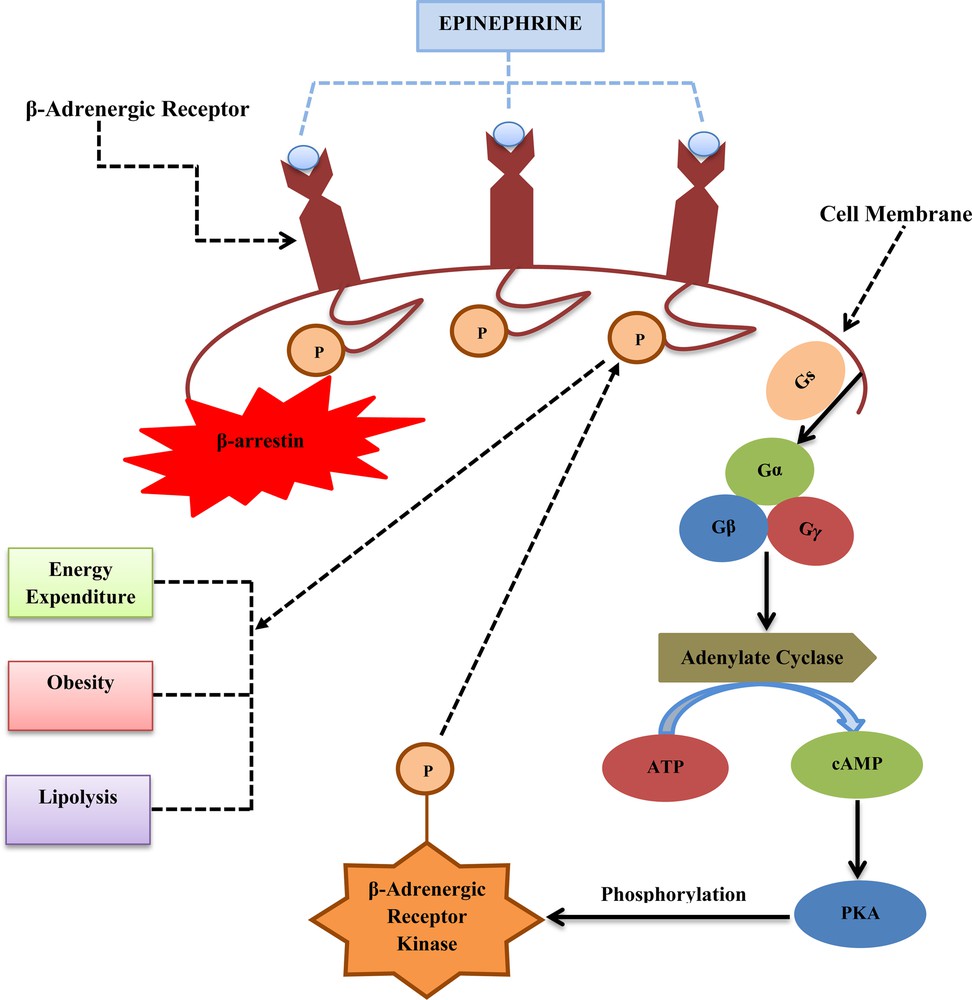
Schematic representation of β-adrenergic receptor pathway: this figure depicts the β-adrenergic receptor, which is stimulated by a neurotransmitter, epinephrine, which triggers a Gs protein family Gα. This Gα subunit binds to AC and converts cytoplasmic ATP to cAMP where cAMP activates PKA, which phosphorylates serine and threonine residues on β-adrenergic receptor kinase. β-adrenergic receptor kinase phosphorylates serine and threonine residues on the β-adrenergic receptor, which helps to activate β-arrestin to stop the action of epinephrine by restricting the further stimulation of the Gs protein. By this mechanism, the above pathway helps to monitor energy expenditure, lipolysis and β-adrenergic receptor gene polymorphisms in humans.
The mechanism of action of β-adrenergic receptor is as following: β-adrenergic receptor follows the PKA/cAMP pathway activation through SNS. Firstly, epinephrine (neurotransmitter) stimulates the β-adrenergic receptor, which in turns activates a Gs protein family, especially Gα. Then, Gα binds to adenylate cyclase (AC) to catalyse the conversion of cytoplasmic ATP to cAMP. After that, cAMP activates cAMP-dependent kinase, PKA that of phosphorylate serine and threonine residues on β-adrenergic receptor kinase. β-adrenergic receptor kinase itself is a serine/threonine kinase which will then phosphorylate serine and threonine residues on the β-adrenergic receptor itself. This will lead to β-arrestin activation, which shuts down the action of epinephrine and restricts the extra stimulation of the Gs protein [202]. Finally, this pathway helps to regulate the energy expenditure, lipolysis and β-adrenergic receptor gene polymorphisms in humans [203,204].
ADRB1 (β1-adrenergic receptor), ADRB2 (β2-adrenergic receptor) and ADRB3 (β3-adrenergic receptor) receptor genes are included in the β-adrenergic receptor family. Mutation in these genes leads to obesity phenotypes. ADRB1 is regarded as the probable candidate gene for obesity and plays a role in the catecholamine-induced energy homeostasis. It helps in the energy expenditure and lipolysis in adipose tissues. ADRB1 gene variant Arg389Gly polymorphism interacted to cause obesity from childhood to adulthood [205]. Linne (2005) has identified that two nsSNPs polymorphisms (Ser49Gly and Gly389Arg) cause long-term weight gain and adult-onset overweight in women [206]. ADRB2 gene polymorphism has been identified to cause obesity in women and lipid metabolism on codon 27 (Gln27Glu) [203,207–210]. Allele 27Glu is linked with increased BMI, subcutaneous fat and elevated levels of leptin and triglyceride in men, while in women it is associated with increased BMI, body fat mass and waist–hip ratio [209–211]. ADRB3 gene plays an important role in adipocyte metabolism, anti-diabetes and anti-obesity properties [212,213]. A common polymorphism Trp64Arg in this gene has been associated with a decrease in lipolytic activity and lipid accumulation in the adipose tissue with 18.3% of adolescent obesity variation [214].
5.2.2.2 Mutation in uncoupling proteins (UCPs) gene family
The UCPs gene family has different members like UCP1, UCP2 and UCP3. UCP1 is expressed in brown adipose tissue and helps in thermogenesis, UCP2 is ubiquitously present in any tissue and UCP3 is expressed in skeletal muscle and brown adipose tissue. UCP1, UCP2 helps in energy metabolism [212,213]. UCP2 and UCP3 are regarded as obesity gene candidates. UCP2 gene variants have been identified: variant Ala55Val on exon 4, 45 base pair insertion/deletion in the untranslated region of exon 8 and 2866G/A mutation in the promoter region associated with obesity [212,213,215].
5.2.2.3 SLC6A14 gene mutation
The SLC6A14 gene is a novel candidate for obesity because it encodes an amino acid transporter that regulates tryptophan accessibility for serotonin synthesis and may affect appetite control and energy balance [140]. It is highly expressed in the CNS, especially in those regions of the hypothalamus where feeding behaviour is regulated and act by modulating tryptophan availability for the serotonin synthesis [216]. This gene having SNP rs2071877 is strongly associated with polygenic obesity in the Finnish population. In addition, a haplotype including this SNP, together with SNP rs2011162 (located in intron 12) showed a significantly different allele frequency in obese versus control subjects [139,140]. Our recently published in silico study of SLC6A14 gene demonstrated that there were five nsSNPs i.e. rs369615885, rs199531433, rs200351649, rs182839907 and rs376518536 predicted as deleterious with PolyPhen 2.0, SIFT dbSNP138 and I-Mutant 2.0 tools, which might play a key role in the development and causal effect of human obesity phenotypes [217].
5.3 Syndromic forms of obesity
5.3.1 Due to chromosomal rearrangement
5.3.1.1 Prader–Willi syndrome
This type of obesity syndrome is characterized by lack of the paternal segment 15q11.2-q12, either by deletion of the paternal critical segment or by the loss of the complete paternal chromosome 15 [218]. The prevalence of this syndrome is one in 25,000 of births [219]. In this type of syndrome, ghrelin increases the appetite by interacting to POMC/CART and NPY neuronal pathways in the hypothalamus leads to obesity phenotype. It is associated with obesity, mild mental retardation, short stature and an unusual facial appearance [149].
5.3.1.2 WAGR syndrome
Wilms tumour, anorexia, ambiguous genitalia and mental retardation (WAGR) syndrome are associated with a deletion at chromosome 11p13 (location of the WT1 and PAX6 gene) [149,220]. This syndrome has been also associated with a deletion in the brain-derived neurotrophic factor (BDNF) gene leads to obesity phenotype.
5.3.1.3 Sim-1 syndrome
Single minded-1 (Sim-1) syndrome is characterized by the deletion at chromosome 6q. In humans, deletion or disruption of the SIM1 region results in early-onset obesity linked to hyperphagia, developmental delay and hypotonia [221].
5.3.2 Pleiotropic
5.3.2.1 Bardet–Biedl syndrome (BBS)
BBS is related to early-onset obesity and associated with polydactyly, learning disabilities, dyslexia, progressive rod–cone dystrophy, hypogonadism and progressive renal abnormalities [150]. About 14 loci (BBS genes) and many mutations have been identified at the molecular level [218,222].
5.3.2.2 Fragile X syndrome
This syndrome is associated with severe mental retardation, large ears, macrocephaly (big head), prominent jaws, high pitch in voice, and mild obesity. Molecular cloning of the fragile X locus showed unbalanced growth of polymorphic CGG trinucleotide repeats present in the FMR1 (fragile X mental retardation) gene [223].
5.3.2.3 Cohen syndrome
It is an autosomal recessive and genetically homogeneous disorder characterized by childhood mild obesity, mental retardation, small stature and microcephaly (small head). Evidences of this syndrome are present in the Finnish population and worldwide also [224]. In this type of syndrome, COH1 gene is responsible for the mutation, located at chromosome 8q22 [149]. At this genetic locus, COH1 gene causes mutations in different ethnic groups [225].
6 Obesity versus epigenetics and GEI
There are some evidences of epigenetic parameters of metabolic diseases sustaining a relation between genes and environment. This type of relation between genes and environment, epigenetics and metabolic disorders influence the epigenome and is one of the reasons for the development of obesity (Fig. 7) [10–12]. The GEI with the epigenome intervene the expression of genes linked with increased adiposity has been reported [226]. Obesity-related genes have been linked to GEI and epigenome, for instance; 2-oxoglutarate-dependent nucleic acid demethylase, an enzyme related to FTO, eliminates the methyl groups from DNA [227]. Apart from this, MC4R and leptin genes have also been involved in altering the methylation patterns due to high fat diet [228,229]. Many other genes are also concerned with GEI and epigenetics targets for obesity: these types of genes are called epi-obesogenic genes [11].
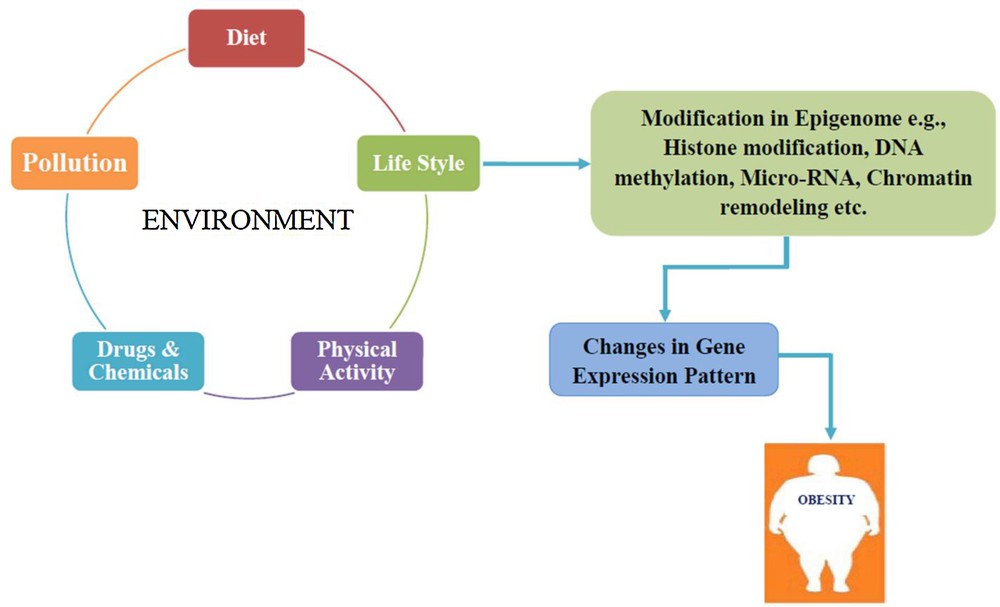
Schematic representation of the relation between GEI, epigenetics and obesity: environmental factors and epigenetic modifications such as histone modification, DNA methylation, micro-RNA and chromatin remodelling caused changes in the gene expression pattern, leading to the development of obesity.
7 Concluding remarks
Obesity is a very common, complex, epidemic, and serious health problem worldwide. The materials presented in this review in combination with molecular genetics of human obesity, ORHC and energy homeostasis are supporting the current scenario of modern research and the prevalence of this epidemic. Melanocortin system plays an important role in the regulation of energy balance and homeostasis processes. Imbalance of energy intake and energy expenditure lead to obesity, an epidemic. Nowadays, less physical exercise, excessive food intake, sedentary lifestyles, junk food and alcohol consumption are crucial factors for the development of adult as well as child obesity. Apart from these habits, many obesity susceptible genes (127 sites on human genome) have been identified by GWAS studies as being responsible for the development of human obesity. Recent genotyping techniques focusing on low-frequency and rare genetic variants, studying populations of wide-range ancestry, will become even more important, as such variants are more likely to be ancestry-specific.
Our most recent in silico studies of FTO and SLC6A14 genes based on several computational tools predicted four nsSNPs (rs139000284, rs139577103, rs368490949 and rs373076420) in the case of FTO and five nsSNPs (rs369615885, rs199531433, rs200351649, rs182839907 and rs376518536) in the case of SLC6A14 gene as deleterious. These predicted nsSNPs along with future functional in vitro and in vivo studies might be helpful to better understand their causal effect on obesity phenotypes.
Genes that are predisposed to obesity in combination with environmental factors help to better understand disease etiology, treatment, and prevention. There have been a few reports only identified in GEI and epigenetics studies in relation to human obesity. Thus, future studies should give more information about new technologies and analytical interventions in order to understand and improve the complexity of human obesity due to GEI and epigenetics. Henceforth, this review might be supported in the current insights into the genetic concept of human obesity, ORHC, and energy homeostasis.
Disclosure of interest
The authors declare that they have no competing interest.
Acknowledgements
The authors are grateful to VIT University, Vellore, Tamilnadu, India, for allowing them to carry out this work. Mr. Rajan Kumar Singh is thankful to the VIT university for providing an institutional research fellowship. Mr. Permendra Kumar is also thankful to UGC, Govt. of India for getting a UGC–RGNF–SRF fellowship.