1 Introduction
The ability of the nervous system to synthesize steroids was originally discovered in mammals [1–3] and then extended to other vertebrates, including fish, amphibians, and birds [4–9]. This suggests that neurosteroidogenesis is a conserved property in vertebrates.
Many studies have revealed the important roles of the potential of neurosteroids in the mediation of several brain functions, including sexual differentiation and reproductive behavior [8,10]. Among the different enzymes involved in neurosteroid synthesis, a prominent role is played by cytochrome P450 aromatase (P450 aro), the key enzyme in the estrogen synthetic pathway, which irreversibly converts testosterone into estradiol. Aromatase is a member of the P450 cytochrome family encoded by the gene cyp19 [11]. Aromatase has been found in the brain of all vertebrates, from fish to mammals [12,13]. In fish, the localization of P450 aro has been described in the central nervous system of Atlantic halibut [14], Protandrous (black porgy fish) [15], plainfin midshipman [16], zebrafish [9,17,18], rainbow trout [19], pejerrey fish [20], and killifish [21]. Among amphibians, the brain expression of P450 aro mRNA is already detectable in the early developmental stages of Xenopus laevis [22,23] and Pleurodeles waltl [24], and it is highly expressed until metamorphosis. A detailed description of the anatomical distribution of aromatase enzyme is reported by Burrone et al. [25] in the brain of Pelophylax esculentus. In this species, sex-specific expression of the P450 aro gene has also been observed in the brain [26]. Furthermore, brain aromatase has been investigated in turtles [27,28], snakes [27], and two species of lizard (Anolis carolinensis and Cnemidophorus uniparens) [29–31].
In some vertebrates, brain aromatase has emerged as a potential factor contributing to the activation of sex-typical behavior (for reviews, see [12,32–34]). In the quail, hypothalamic aromatase modulates testosterone-induced aggressiveness by regulating the amount of estradiol available for receptor binding [13,35]. In songbirds, estrogens can organize and activate masculine neural circuits, such as those involved in vocalization [12]. However, the estrogens are assumed to possess the functional properties of neuromodulators, coordinating a variety of morphological, physiological and behavioral traits required for reproductive success [10]. At least in terms of male sexual behavior, brain estrogens appear to have a larger role in the rat and the mouse [13,32].
Birds and mammals both evolved from reptilian ancestors [36,37]; therefore, investigations on reptiles could elucidate the evolution of mechanisms regulating reproduction [38]. In this study, we investigate the distribution and activity of P450 aro in the brain of the adult male lizard (Podarcis sicula) together with the hormonal profile of sex steroids, testosterone, and 17β-estradiol during significant phases of the reproductive cycle. This species, being a seasonal breeder, provides a useful model to better understand the relationship between brain sex hormones levels and the phases of reproduction. Moreover, we have also recently investigated the localization and expression of P450 aro in the testis of P. sicula, discovering that it has a significant influence in the control of steroidogenesis and spermatogenesis [39], as in the other enzymes involved in steroidogenesis [40]. The localization of P450 aro in the Podarcis brain and the hormonal profile of testosterone and 17β-estradiol could underline the relevance of neural steroidogenesis in the control of reproduction in reptiles.
The study was performed in three different phases during the reproductive cycle: the pre-reproductive period (February–March), the reproductive period (May–June), and finally in the post-reproductive period (July–August). The pre-reproductive and post-reproductive stages are the periods defined by testicular activity blocking, characterized with typical estradiol peaks; the reproductive period is the mating period, which involves sperm production, preparation for ejaculation, and the production of high levels of testosterone [41,42].
2 Materials and methods
2.1 Animals
Sexually mature males of P. sicula were collected in Campania (southern Italy) during the pre-reproductive period (March 2013), the reproductive period (May 2013), and the post-reproductive period (July 2013). The animals, once collected, were maintained in a soil-filled terrarium and fed ad libitum with Tenebrio molitor larvae for approximately 15 days, the time required to recover from the capture stress. The experiments were approved by institutional committees (Ministry of Health of the Italian Government) and organized to minimize the number of animals utilized. Animals were killed by decapitation after deep anesthesia with ketamine hydrochloride (325 pg·g−1 body mass; Parke-Davis, Berlin, Germany). The sexual maturity of each animal was assessed by morphological parameters and histological analysis [43–47]. We utilized twelve animals for each period. The brains were quickly removed.
For some animals, the brain has been fixed for 24 h in Bouin's solution, dehydrated, and embedded in paraffin wax. Consecutive sections 7 μm in thickness were placed on polylysine glass slides (Menzel-Glaser, Braunschweig, Germany) and utilized for immunohistochemistry procedures. For the other animals, the brain was stored at −80 °C and used for biochemical measurements.
2.2 Immunohistochemistry
Lizard brains (N = 3) from each reproductive period were fixed in Bouin's solution, dehydrated in a graded ethanol series and embedded in paraffin as previously reported. Five-μm-thick sagittal sections of Bouin-fixed brain on poly-l-lysine slides were treated as previously reported [48–54]. Briefly, the sections were treated with 10 mM citrate buffer pH 6.0, and then incubated in 2.5% H2O2 in methanol for endogenous peroxidase blocking. The non-specific background was reduced with the incubation in normal goat serum (Pierce, Rockford, IL) for 1 h at room temperature. The sections were then treated overnight at 4 °C with a primary rabbit anti-human P450 aro antibody (Santa Cruz Biotechnology), previously validated in Podarcis [39], diluted 1:100 in normal goat serum. The reaction was revealed with a biotin-conjugated goat anti-rabbit secondary antibody and an avidin-biotin-peroxidase complex (ABC immunoperoxidase kit, Pierce), using DAB (Sigma-Aldrich) as chromogen. Three investigators individually blindly ranked the intensity of staining. Negative controls were carried out by omitting primary antibodies. The immunohistochemical signal was observed using a Zeiss Axioskop microscope; images were acquired by using AxioVision 4.7 software (Zeiss).
2.3 Sex steroid assays and measurement aromatase activity in the brain
Testosterone and 17β-estradiol determinations in lizard brains (N = 6, three pools of two brains each) were carried out in the pre-reproductive, reproductive, and post-reproductive periods. The brains were homogenized 1:10 (w/v) with PBS 1X. The homogenate was then mixed vigorously with ethyl ether (1:10 v/v) and the ether phase was withdrawn after centrifugation at 3000 g for 10 min. The upper phase (ethyl ether) was transferred to a glass tube and left to evaporate on a hot plate at 40–50 °C under a hood. The residue was dissolved in 0.25 mL of 0.05 M sodium phosphate buffer, pH 7.5, containing BSA at a concentration of 10 mg/mL, and then utilized for the ELISA assay (Diametra) [55–59]. The sensitivities were 32 pg/mL for testosterone and 15 pg/mL for 17β-estradiol.
Aromatase activity was measured by evaluating the in vitro conversion rate of testosterone into 17β-estradiol. Briefly, brain samples (N = 3 from each period) were weighed and homogenized (1:2 w/v) in cold 20 mM K2HPO4 buffer, 1 mM EDTA, 3 mM NaN3, 10% glycerol, 1 mM β-mercaptoethanol, pH 7.4. For routine assays, the following were added to the test tubes: 10 μL of a testosterone solution in propylene glycol (l0 μg/mL), 250 μL of brain suspension, and 100 μL of NADPH solution (3 mg/mL), supplemented with antibiotics (penicillin 50 IU/L, streptomycin 50 IU/L and nistatin 100 IU/L). The suspension was vortexed and incubated for 60 min at 22 °C. Control tubes contained all components, except testosterone. Incubations were stopped by rapid freezing in an ice bath. The steroids were then extracted three times with 10 mL of diethyl ether. The incubation times and temperatures were chosen based on preliminary data that indicated that testosterone conversion increased linearly in the range of 15–30 °C and 15–90 min incubation time. Ethers were pooled and dried, and samples were utilized for 17β-estradiol determination by the ELISA method (Diametra) [55–59].
2.4 Statistical analysis
ANOVA followed by a Student–Newman–Keuls's test was used to evaluate differences between groups as previously reported [60]. Differences were considered statistically significant at P < 0.05. All data were expressed as the mean ± S.D.
3 Results
3.1 Brain aromatase immunolocalization
Immunohistochemistry highlighted a wide distribution of P450 aro in the brain of P. sicula during the reproductive cycle. Indeed, immunolabeling was evident in the telencephalon (Fig. 1), the diencephalon (Fig. 2), the mesencephalon (Fig. 3), as well as in the rhombencephalon (Fig. 4).
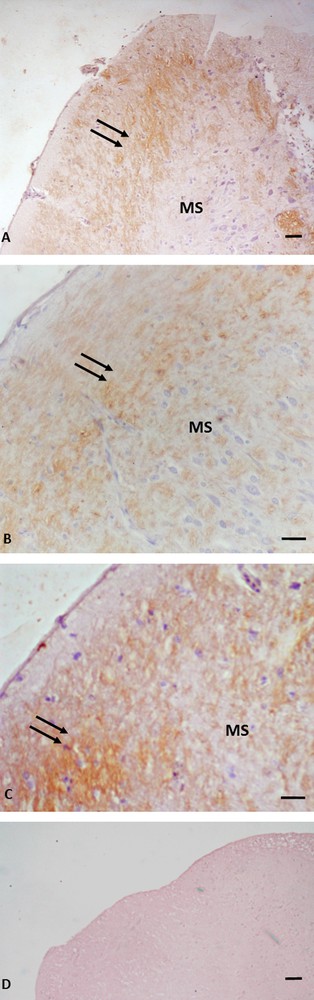
Immunohistochemistry for P450 aromatase in Podarcis telencephalon. Immunolocalization signal appears as brown areas. A. Pre-reproductive period. B. Reproductive period. C. Post-reproductive period. D. Control. Immunopositivity (arrows) is evident in cellular bodies and nerve fibers in rostral, ventral and dorsal to medial septum (MS) positions. No labelling is evident in the control. The scale bars correspond to 50 μm in A, D, 20 μm in B and C.
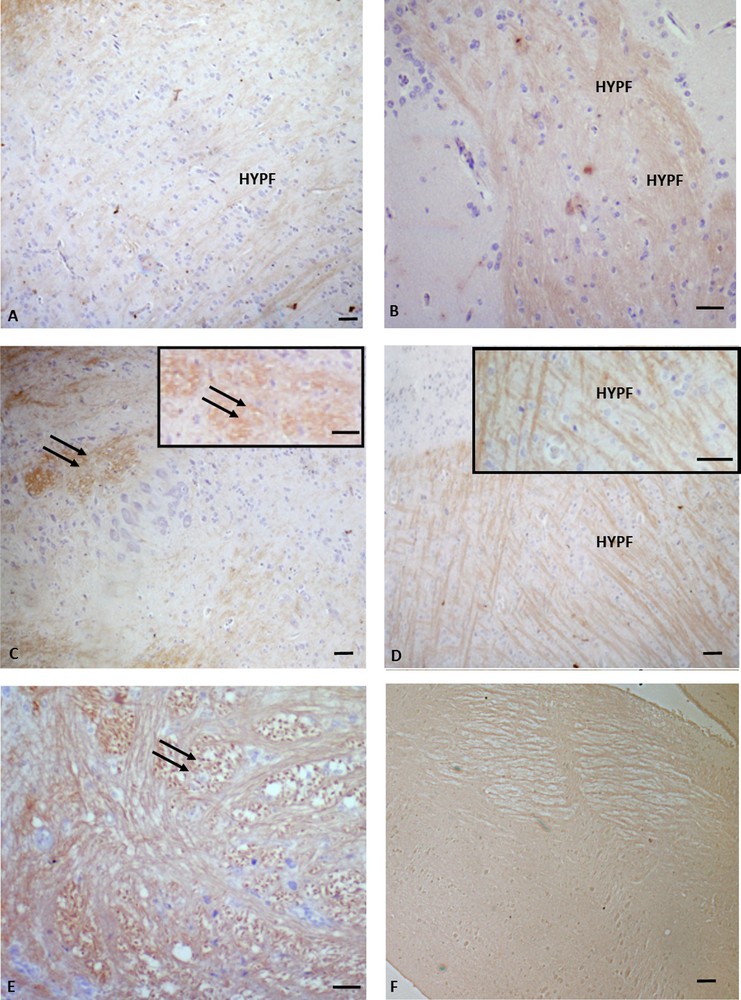
Immunohistochemistry for P450 aromatase in Podarcis diencephalon. Immunolocalization signal appears as brown areas. A. Pre-reproductive period. B, C, C insert. Reproductive period. D, E. Post-reproductive period. F. Control. Immunopositivity is evident in the hypothalamic nerve fibers (HYPN) as well as in the periventricular parvocellular and magnocellular periventricular nuclei (double arrows). No labelling is evident in the control. The scale bars correspond to 50 μm in A, C, D, F, 20 μm in B and E, 10 μm in C (inset) and D (inset).
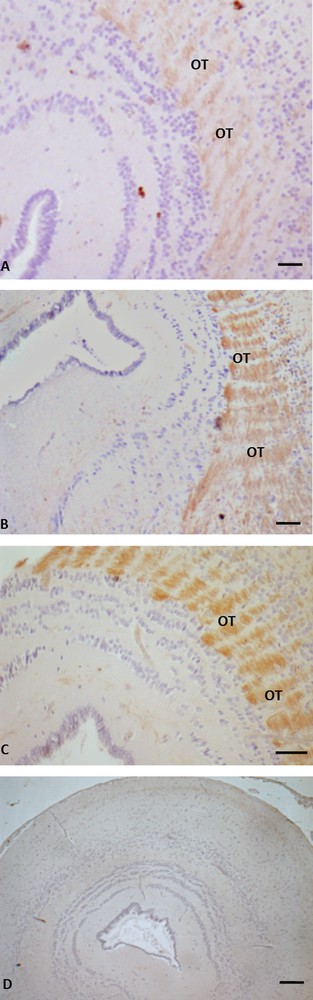
Immunohistochemistry for P450 aromatase in Podarcis mesencephalon. Immunolocalization signal appears as brown areas. A. Pre-reproductive period. B. Reproductive period. C. Post-reproductive period. D. Control. Immunoreactivity is evident in the tectum opticum district (OT); no labelling is evident in the control. The scale bars correspond to 20 μm in A, D, and B, 10 μm in C.
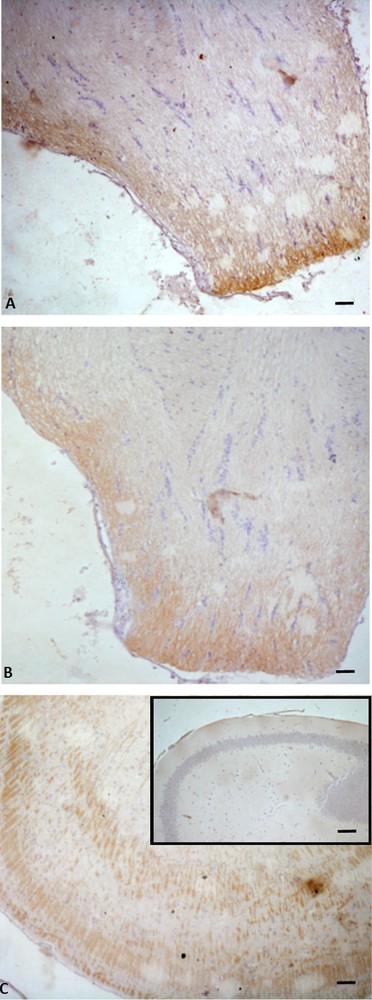
Immunohistochemistry for P450 aromatase in Podarcis rhombencephalon. The immunolocalization signal appears as brown areas. A. Pre-reproductive period. B. Reproductive period. C. Post-reproductive period. C (inset). Control. Scale bars correspond to 50 μm in A, B, C (inset) and C.
In the telencephalon, a rostral and continuum population of immunoreactive neurons, starting in the medial septum and continuing dorsally up to the caudal-most portion of the septum in the posterior telencephalon, was observed in all the examined periods (Fig. 1A, B, C). In the diencephalon, immunoreactive P450 aro ir-neurons were localized in the preoptic periventricular (periventricular parvocellular and magnocellular periventricular) nucleus, and the signal was evident both in cell bodies (Fig. 2C, C inset, E) and in hypothalamic nerve fibers (Fig. 2A, B, D, D, inset). The more caudal population of P450 aro ir-neurons extended rostro-caudally from diencephalon to mesencephalon, where immunopositivity occurred in the tectum opticum (Fig. 3 A, B, C), roughly at rhombencephalon, where immunolabelled neurons occurred in the medulla oblongata and cerebellum (Fig. 4A, B, C).
Furthermore, the immunolabeling signal became more and more intense, moving from the pre-reproductive (Fig. 2A) to the reproductive (Fig. 2B, C), and finally to the post-reproductive period (Fig. 2D, E). Such a modification occurred also in the mesencephalon (Fig. 3) and the rhombencephalon (Fig. 4). Controls obtained by omitting the primary antibody were not immunolabelled (Figs. 1A, 2F, 3D, 4C insert). Fig. 5 schematizes the distribution of P450 aro in the brain of P. sicula.
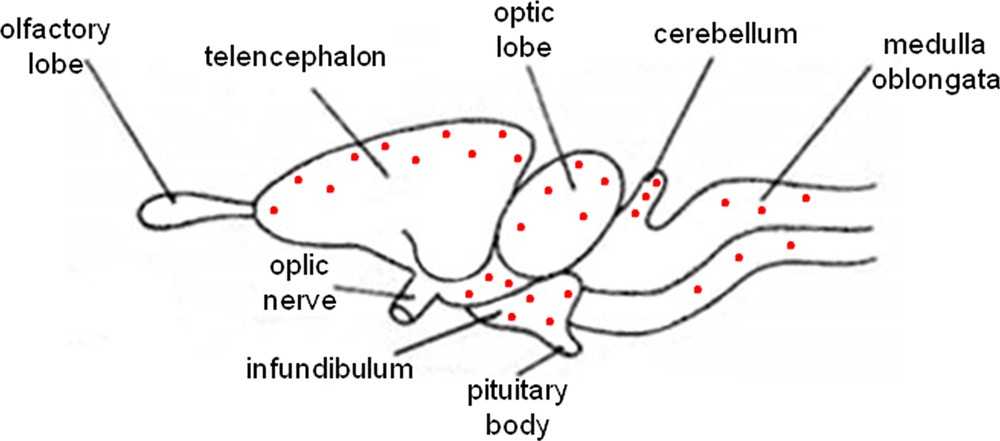
Schematic sagittal section of lizard brain depicting the distribution of P450 aromatase immunoreactivity.
3.2 Brain aromatase activity and sex steroid assays
A comparison of the sex hormone levels estimated in the lizard's brain during the main phases of the reproductive cycle revealed that the testosterone levels in the brain were the highest (P < 0.05) during the pre-reproductive period and the lowest (P < 0.05) in the reproductive and post-reproductive periods. The hormonal profile was greater in the reproductive than in the post-reproductive phase (Fig. 6A). Conversely, brain estradiol levels were significantly higher (P < 0.05) during the post-reproductive period than during the pre-reproductive and reproductive phases (Fig. 6B). However, during the reproductive phase, the amounts of brain estradiol levels were greater (P < 0.05) than in the pre-reproductive period.
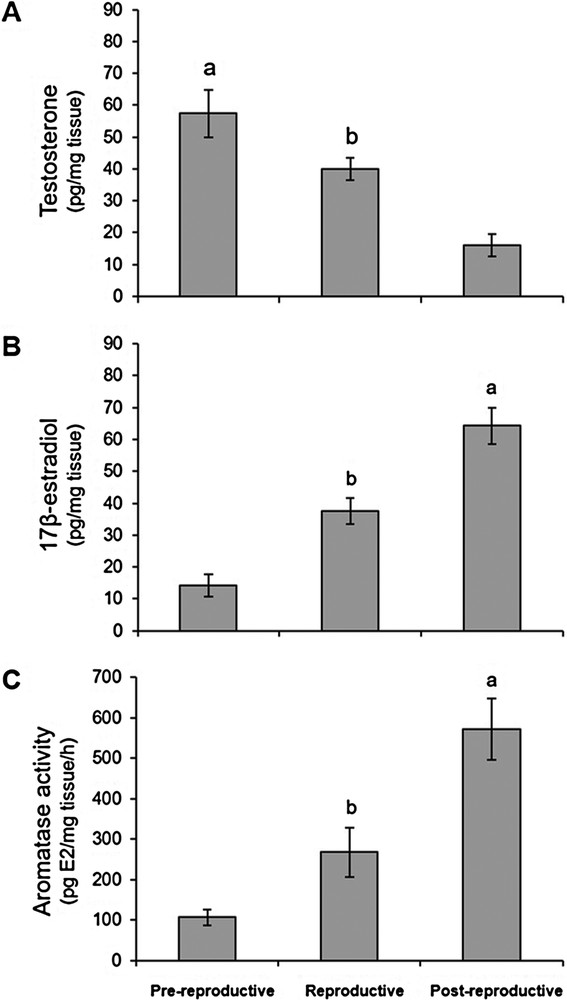
Testosterone (A) and 17β-estradiol (B) levels in the brain from lizards at the pre-reproductive, reproductive, and post-reproductive stages. (C) Aromatase activity evaluated as the in vitro conversion rate of testosterone into 17β-estradiol in the brain of the male lizard Podarcis, at different reproductive phases. a,b P < 0.05.
Furthermore, aromatase activity, evaluated as the quantity of brain estradiol produced when testosterone was used as substrate, was greater (P < 0.05) in the post-reproductive period than in the other two examined phases; it was also higher (P < 0.05) in the reproductive phase than in the pre-reproductive one (Fig. 6C). Aromatase activity found in the lizard's brain during the three different periods of the reproductive cycle was positively correlated with the changes in the endogenous levels of estradiol in the brain, whereas it was negatively correlated with those of testosterone during the different phases of the reproductive cycle.
4 Discussion
The distribution of P450 aro in the brain has been extensively described in fishes, birds, and mammals. P450 aro localization has been detected in various telencephalic and mesencephalic areas, including the medial preoptic area, the ventro-medial nucleus of the hypothalamus, and the amygdala, i.e. regions involved in sexual behavior and reproduction [61–63]. In quails, morphological studies have revealed that the preoptic aromatase is specifically expressed in the sexually dimorphic medial preoptic nucleus, a structure where testosterone action is fundamental to activate male sexual behavior [64]. The present investigation demonstrates that, in the brain of P. sicula, P450 aro ir-cells are widely represented in the telencephalon, the diencephalon, the mesencephalon, and the rhombencephalon in the pre-reproductive, reproductive, and post-reproductive periods. Moreover, we show that, also in the brain of Podarcis, a neurosteroidogenic process occurs, leading to the synthesis of 17β-estradiol. It is noteworthy that P450 aro immunolabeling was particularly evident in the diencephalic, mesencephalic, and rhombencephalic regions, during the reproductive and post-reproductive periods. However, specific immunolabeling was observed in the medial septum, the preoptic area, and the hypothalamus. These results are consistent with the function of these sites in the sexual behavior of male lizards [65–67]. Indeed, it has been reported that lesions of the hypothalamus-preoptic area and amygdala in green anoles inhibit masculine courtship and copulation, impairing male sexual behaviors [65–67]. On the other hand, the localization of P450 aro reported here is the same as that reported in lizard brain areas involved in neurosteroid synthesis [8]. Interestingly, the Cyp17 gene, encoding the enzyme required for biosynthesis of androgenic precursors, is widely distributed in different parts of the brain of Cnemidophorus uniparens, including the preoptic area and the hypothalamus [31], which, in the brain of P. sicula, are immunolabelled by the P450 antibody. Moreover, we observed a strong association between the distribution of P450 aro in the brain of P. sicula and the expression of Cyp19, the gene encoding the P450 aro in the brain of both the whiptail lizard (Cnemidophorus uniparens) and the green anole lizard (Anolis carolinensis) [29,31].
Our data also showed that the activity of brain aromatase, which is low during the pre-reproductive period, increases progressively during the reproductive phase, reaching the greatest activity during the post-reproductive period. The aromatase profile during different phases of the reproductive cycle positively correlates with the levels of estradiol in the brain. These data overlap with those observed in the testis of P. sicula during the reproductive cycle [39]. The increase of aromatase activity, which, at the level of testis, causes an arrest of the spermatogenesis, at the encephalic level corresponds to the higher aromatase expression in specific brain areas and could have a pivotal role in the specific sexual behavior of animals during the reproductive cycle.
It is interesting to note that the increased activity of P450 aro in the brain correlates at the cytological level with the presence of a P450 aro immunohistochemical signal, which progressively becomes more and more intense from the pre-reproductive phase until the post-reproductive phase. It is noteworthy that Cohen and Wide [31] found an increased density of aromatase in the preoptic area from breeding lizards compared to non-breeding lizards. Seasonal differences in brain aromatase expression have been found in frogs [26,68], birds [69,70], and bats [71].
In contrast to estradiol, the profile of testosterone measured in the brain in the different phases of the reproductive cycle shows a diverse distribution from what has been observed at the testicular level [39]. In fact, at the encephalic level, the most relevant synthesis of testosterone occurs in the pre-productive phase, while at the testicular level, the highest testosterone levels were found during the reproductive phase. The highest levels of brain testosterone recorded during the pre-reproductive phase could have a dual role in promoting the reproductive activity of lizards by:
- • stimulating the activity of aromatase, testosterone being the substrate of this enzyme;
- • determining the aggressive behavior typical of this species in the pre-reproductive phase.
In fact, the androgens have a pivotal role in regulating the aggressive behavior of males, which in this phase are engaged in fights with the other males. It is well documented that male P. sicula emerges from the winter shelters in early March (pre-reproductive period) with high levels of testosterone, inducing aggressive behavior, so that they can engage in struggles between males for male dominance. Subsequently, in April–May (reproductive period), testosterone and aggression decrease, and the males can be involved in couplings with females [42].
The above considerations report that the role of brain aromatase activity in the male lizard progressively increases from the reproductive to the post-productive phase with a consequent rise in estradiol levels and may account for explaining male reproductive behavior in P. sicula. Furthermore, it is possible that aromatase, increasing the estradiol level could contribute to slowing delay the spermatogenic process during the post-reproductive period.
5 Conclusion
Our data show that sex steroid hormones are synthesized in the brain of P. sicula, as in other vertebrates, in a process called neurosteroidogenesis. It shows dynamic changes during the different phases of the reproductive cycle. The presence and the distribution of P450 aromatase in specific brain regions strongly suggests the involvement of this enzyme in sexual behavior. Furthermore, the natural changes of its activity in the brain during the different phases of the reproductive cycle of P. sicula could have a pivotal role in balancing the local levels of testosterone and estradiol, confirming an active role of neurohormones in the neuroendocrine/behavioral regulation of the reproductive axis.
Acknowledgments
This work was supported by the Federico II Naples University and Luigi Vanvitelli Campania University.