1 Introduction
Several extensive reviews of the location of double bonds in conjugated alkadienes by GC/MS have been published, in particular by using ion–molecule reactions with CI reactant gas such as (CH3)3CCl is particularly effective 〚1〛, but there is no derivatisation method really effective for the location of double bonds in conjugated alkadienes.
The use of dimethyl disulfide (DMDS) addition in alkadienes has been studied and has shown that a six-, five- or four-membered cyclic thioether is synthesised if the number of methylene groups that separate the double bonds is smaller than 4 〚2–12〛. The reaction between dimethyl-disulfide (used in excess at 80 °C) and conjugated alkadienes yields five-membered cyclic thioethers. These conjugated alkadienes, after electronic ionisation (EI), give specific even-electron fragment ions, obtained from two bond cleavages in the cyclic thioether. The possible simultaneous formation of one monoderivatised compound allows determination of the position of the double bonds 〚13〛. However, the low yields of these reactions sometimes make the derivatised compounds undetectable by the GC/MS apparatus; this method is not effective for conjugated alkadienes substituted on the ethylenic carbons.
The use of ethanethiol to derivatise alkynes has allowed the location of the triple bond, whereas the use of DMDS did not. The use of DMDS does not always permit the location of the unsaturation after EI, unlike the use of ethanethiol, which has always permitted the location of the double bond for highly substituted alkenes 〚14〛.
This ethanethiol method has not been tested for the location of the double bonds of substituted conjugated alkadienes. The present paper deals with the behaviour of some substituted conjugated alkadienes listed in Table 1, after addition of ethanethiol, and the resulting possibility of the location of the unsaturation by studying the adducts by GC/MS.
The conjugated alkadienes studied.
Compounds | Formulae |
2-methyl-1,3-pentadiene | |
3-methyl-1,3-pentadiene | |
2,3-dimethyl-1,3-butadiene | |
2,4-dimethyl-1,3-pentadiene | |
2,5-dimethyl-2,4-hexadiene | |
2,4-dimethyl-2,3-pentadiene (allene) |
2 Experimental
25 μL of each compound were treated by adding 100 μL of DMDS or 1 mL of ethanethiol, depending on which derivatising agent was used, and 20 μL or 2 mL of iodine solution (6 mg of iodine in 1 mL of diethyl ether). The reaction was carried out in a closed tube for two days at 50 °C with DMDS or 6 h with ethanethiol. The excess iodine was reacted with sodium thiosulfate solution (5% in water). The organic phase was removed and the excess of DMDS or ethanethiol evaporated. The dry extract was diluted with 50 μL of hexane and then analysed by GC/MS.
Electron ionisation mass spectra were obtained using a Nermag R10-10 quadrupole mass spectrometer coupled to a Varian Model 3400 gas chromatograph. The sample was injected in the septum-equipped programmable injector (SPI) mode. The column employed was a fused-silica capillary (30 m × 0.25 mm i.d., 0.2 mm film-thickness) coated with DB5 phase (Chrompack). The column temperature was increased by 10 °C per minute from 100 to 280 °C.
3 Results and discussion
The use of DMDS to locate the double bonds in the compounds listed in Table 1 is not effective. The yield of the derivatisation is very small, for example in the case of 2-methyl-1,3-pentadiene, because the amount of the five-member cyclic thioether, essential for the location of the double bonds 〚13〛, is very small (about 1%); so this method cannot be carried out on a few nanogrammes. In the other cases studied here, the derivatisation is not possible with this experimental protocol, because no DMDS derivative resulting from the addition of methylthio groups across the double bonds was observed.
The use of ethanethiol instead of DMDS seems more efficient. Indeed, GC/MS shows the presence of two products after derivatisation of 2-5 dimethyl-2,4-hexadiene (Fig. 1). Molecular ions at m/z 234 and 172 indicate that the adducts formed correspond to the addition of two CH3CH2SH (m/z 234) across the two double bonds (di-adduct) or the addition of one CH3CH2SH (mono-adduct at m/z 172). The EI spectrum of the di-adduct (Fig. 2) shows the loss of CH3CH2S from the molecular ion at m/z 173 and the loss of CH3CH2SH from this fragment ion to give the fragment ion at m/z 111, and permits confirmation of the fact that this adduct results from the double addition of C2H5SH across the double bonds. The fragment ions at m/z 103 and 131 (very small), on the one hand, and the fragment ion at m/z 117, on the other hand, allow the location of the double bonds. It is interesting to note the loss of CH3CH2SH from the fragment ions resulting from the cleavage between the original ethylenic carbons (m/z 69, 41 and m/z 55).
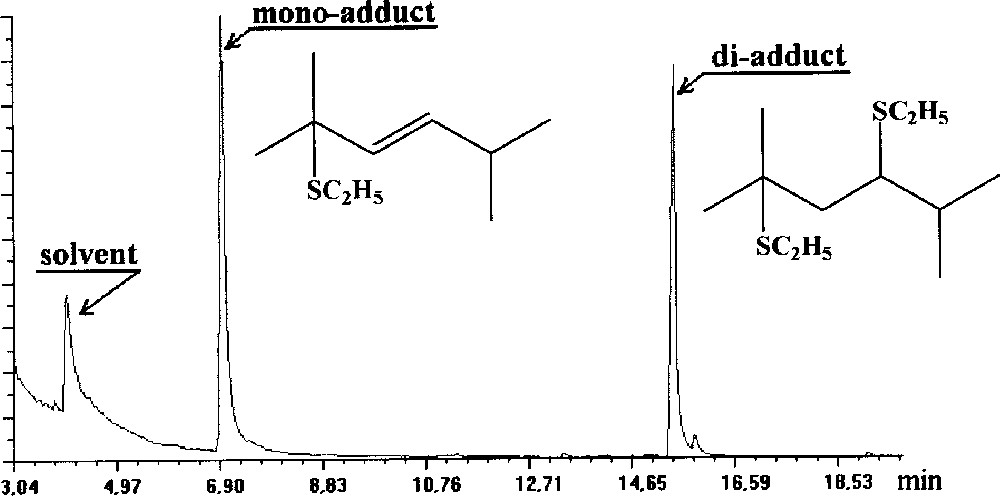
Chromatogram of the mixture obtained from the ethanethiol derivatisation of 2-5 dimethyl-2,4-hexadiene.

Mass spectrum of the ethanethiol derivative di-adduct of 2-5 dimethyl-2,4-hexadiene.
The fragment ions observed in the EI spectrum of the mono-adduct (Fig. 3) allow to determine a molecular structure corresponding to 4-ethylthio-2,5-dimethyl-hexadecene-2 and confirm that this product results from the simple addition of CH3CH2SH. This mono-adduct is not detected again if the reaction time between the ethanethiol and the alkadiene is two times larger.

Mass spectrum of the mono-adduct obtained from the derivatisation of 2-5 dimethyl-2,4-hexadiene.
The other EI spectra are presented in Fig. 4. In each case the mass spectra of the di-adducts allow the location of the double bonds thanks to the molecular ion, the cleavage between the original ethylenic carbons and the systematic loss of CH3CH2SH from the fragment ions resulting from this cleavage.
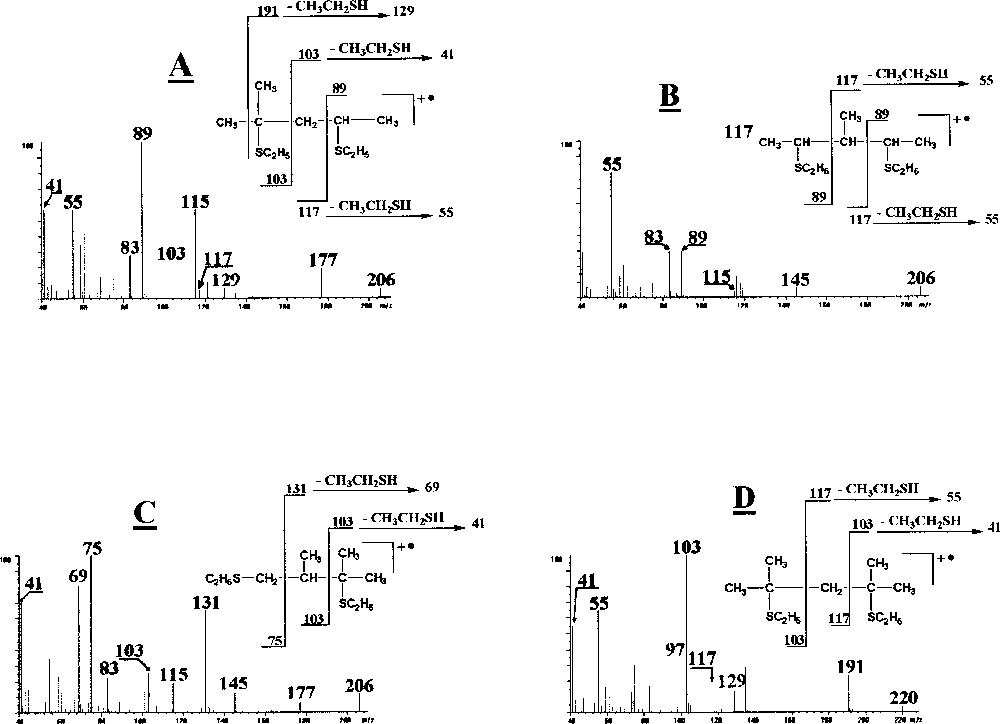
Mass spectra of the ethanethiol derivatives of 2 methyl-1,3-pentadiene (A), 3 methyl-1,3-pentadiene (B), 2,3 diméthyl-1,3-butadiene (C) and 2,4 dimethyl-1,3-pentadiene (D).
Fig. 5 shows the EI mass spectrum of the adduct formed from a particular alkadiene, the 2,4-dimethyl 2,3-pentadiene (allene), after addition of ethanethiol across the double bonds; the fragment ions at m/z 220, 117 and 103 allow once more to locate the double bonds. Nevertheless, the EI mass spectrum is identical to the mass spectrum of the ethanethiol derivative of the 2,4-dimethyl-1,3-pentadiene (Fig. 4), which implies that the location of the double bonds is ambiguous in this case.
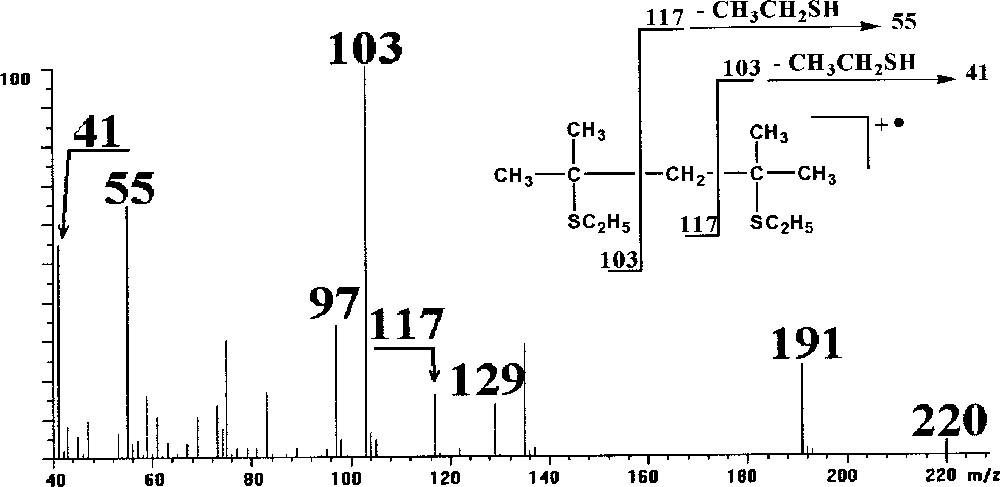
Mass spectrum of the ethanethiol derivatives of the 2,4-dimethyl 2,3-pentadiene.
Fig. 6 recapitulate the systematic fragmentations observed from the compounds obtained after the reaction between the conjugated alkadienes and ethanethiol, allowing the location of the double bonds. It is important to note that the ethanethiol derivatives are not obtained with the classical alkadienes (with one or several ethylene groups between the double bonds) and consequently, it is not possible to use definitively this method instead of the DMDS; the two methods are complementary.
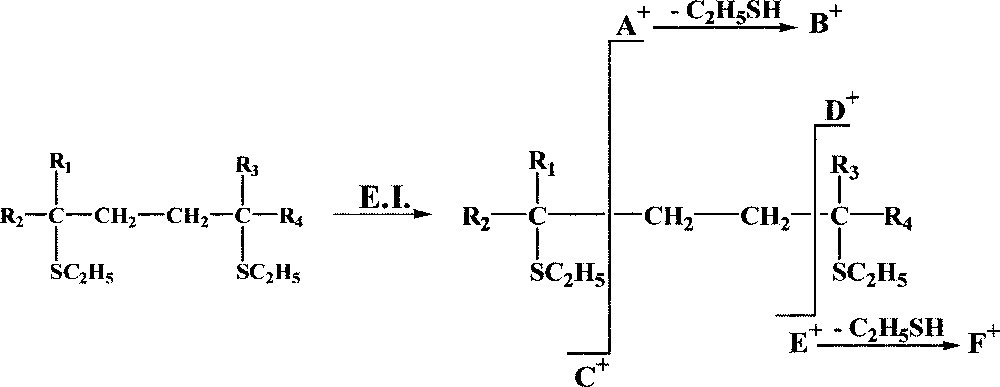
Fragmentations observed from the ethanethiol derivatives, allowing the location of the double bonds.
The detection limits for this method is about 50 pmol l–1 and, consequently, can be probably used for the studying of a complex environmental mixture.
4 Conclusion
The use of DMDS to derivatise the conjugated substituted alkadienes to locate the double bonds after EI is not effective, whereas the use of ethanethiol allowed the location of the double bonds for all the substituted alkadienes studied here. Nevertheless, the two methods are complementary, because the ethanethiol derivatives are not obtained with the classical alkadienes.