1 Introduction
It is nowadays of prime interest to connect research in chemistry and environmental protection. So a number of procedures is now recommended for Green Chemistry [1] involving: either new eco-friendly reagents and catalysts, selected medium such as water, supercritical fluids, ionic liquids or solvent-free reactions, non-classical modes of activation such as ultrasounds or microwaves.
Since the pioneering works of Gedye [2] and Giguere et al. [3], who advocated the use of domestic ovens and solvents for their experiments, more than 1600 publications have appeared to date, testifying to the exceptional interest of microwave (MW) irradiation, which is illustrated by the impressive progression of publications in the field (Fig. 1 ).
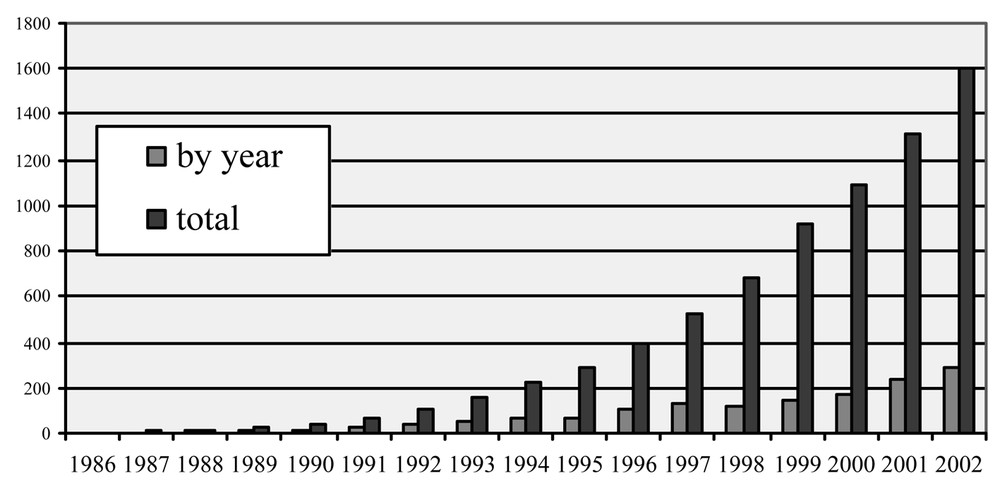
Number of publications dealing with MW in organic synthesis in 1986–2002.
The scope of applications is very extended and concerns a wide spectrum of organic synthesis procedures including, for instance, heterocyclic, organometallic, radio, photo and combinatorial chemistries [4].
Most of these publications [4–9] describe important accelerations for a wide range of organic reactions, especially when carried out under solvent-free conditions. The combination of solvent-free conditions and MW irradiation leads to large reductions in reaction times, enhancements in conversions, sometimes in selectivity, with several advantages of the eco-friendly approach.
The most common benefits described are:
- • very rapid reactions, frequently a few minutes, brought about by high and homogeneous temperatures and combined with pressure effects (if conducted in closed vessels);
- • higher degree of purity achieved due to short residence time at high temperatures;
- • yields often better, obtained within shorter times and with purer products.
2 Microwave exposure and consequences [4]
Microwaves consist in an electromagnetic wave within the range 1 cm–1m in the electromagnetic spectrum. The most usual frequency devoted to industrial, medicinal, domestic or any scientific purposes is imposed by international legislation to 2450 MHz, i.e. to a wave length of 12.2 cm. According to the Planck law, the energy involved in MW–materials interactions is 0.3 cal mol–1 and therefore is largely non-sufficient for molecule excitation and leads to negligible energetic contribution. It thus results in essentially material–wave interactions of electromagnetic nature, consequently with a specificity for polar systems.
It is also noticeable that, in order to have a good compatibility with wavelength, the penetration depth of MW into materials is in the order of magnitude of the decimeter. This fact imposes industrial equipments able to work in continuously.
2.1 Microwave heating
Heating of products submitted to microwave exposure can only result from material–wave interactions. It is brought about by the transformation into heat of a part of the energy contained in the electromagnetic wave.
Polar molecules display the property that they can be oriented along an electric field (dipolar polarization phenomenon). In the absence of this phenomenon, dipoles are orientated at random and molecules submitted to Brownian movement only. In the presence of a continuous electric current, all the dipoles are lined up together in the same direction. If submitted to an alternating current, the electric field is inversed at each alternation with a subsequent tendency for dipoles to move together to follow the field. Such a characteristic induces stirring and friction of molecules, which dissipates as internal homogeneous heating (Fig. 2 ).

Microwave heating.
From this heat dissipation inside the materials, the result is a final repartition in temperature much more homogeneous when compared to classical heating. Microwave heating is therefore an original procedure bringing the following advantages: speed, no inertia, heat affects the product only, ease of use, quick energy transfer in the whole mass without any superficial overheating.
2.2 Specific effects of microwaves (non-purely thermal)
The materials–wave interactions produce heating of the reaction medium by polar molecules (solvents, reagents or complexes, solid supports). To these purely thermal effects can be added specific effects due to MW irradiation (Fig. 3 ).

Microwave effects on organic reactions.
To determine these effects, an accurate comparison is required between MW and conventional heating (Δ), all other conditions being identical (time, temperature, pressure, similar profile of temperature rise). If the results obtained are different, the origin of these specific effects could be due to:
- • better homogeneity and heating speed;
- • intervention of hot spots with high localized microscopic temperatures;
- • variations in activation parameters ΔG≠ = ΔH≠ – T ΔS≠.
Due to previous organization of the polar system under microwaves (dipolar polarization), activation parameters can be modified. This was experimentally proved by Lewis [10] during imidization of polyamic acid (Eq. (1) ) either by conventional heating or under microwave activation, when the activation energy is largely reduced from 105 to 57 kJ mol–1.

Imidization of a polyamic acid.
3 Organic synthesis under microwaves [4,9]
The applications that can implement such a technique are derived from two main types of reactions: thermal reactions, which need high temperatures for long reaction times and where MW will bring acceleration of the processes, lower decomposition of products and consequently enhanced yields, and equilibrated reactions, with displacement by vaporization of light molecules.
3.1 Limitations
The boiling points of solvents are reached rapidly, often inducing safety problems (e.g., explosions). To solve these problems, the operation has to be carried out in closed vessels (generally made of Teflon, a material transparent to MW and resistant up to 250 °C and 80 psi) and using only small amounts of products (roughly 1/10 of the total volume). This of course constitutes a serious limitation (e.g., reduction in MW efficiency as the penetration depth is far below λ, scaling up, etc.). Another main limitation is the absence of measurement and control of temperature. However, these limitations can be overcame by the following two approaches:
- • (1) the use of solvent-free techniques,
- • (2) operation with an adequate reactor allowing homogeneity of electric field and temperature provided by a wave-guide (monomode systems such as Prolablo Synthewave and then CEM Discover) or a wave diffuser (multimode reactors as Milestone Ethos MR system).
There is also need for an accurate measurement and monitoring of temperature through the reaction by modulation of emitted power with an optical fibre.
4 The specific microwave effects [9]
4.1 According to reaction medium
The most suitable situations to observe some MW effects necessarily involve non-polar solvents (as transparent to MW and consequently allowing specific MW interactions with reaction partners). Polar solvents have to be avoided as able to mask specific MW effects on reactants, due to their ability to absorb the waves.
When a non-polar solvent is involved, it is clear that the possibility of intervention of specific (non-purely thermal) effects is evidently dependent on the reaction. It was thus shown that the MW effect is absent during Diels–Alder or ene-reactions [11,12], whereas it is very high for aryl diazepines synthesis [13,14].
By far, the best-adapted situation to take advantage of MW activation is connected to solvent-free reactions. It allows the optimal MW–materials interactions as there is no limitation due to solvent absorption. They can concern three kinds of conditions (see below § 5). They reveal very efficient coupling between solvent-free methods and MW activation as a promising and fruitful procedure for Green Chemistry.
The interpretation of MW effects in terms of dipole–dipole interactions is identical to explain solvent effects (Hughes–Ingold theory) [15]. So, aprotic dipolar solvents should be avoided and replaced by MW activation using a non-polar solvent or, even better, no solvent. A striking example lies in the regioselective phenacylation of 1,2,4 triazole (Fig. 4 ), which can lead to alkylation in position 1 (N1), 2 (N2) or both (N1,4) [16].
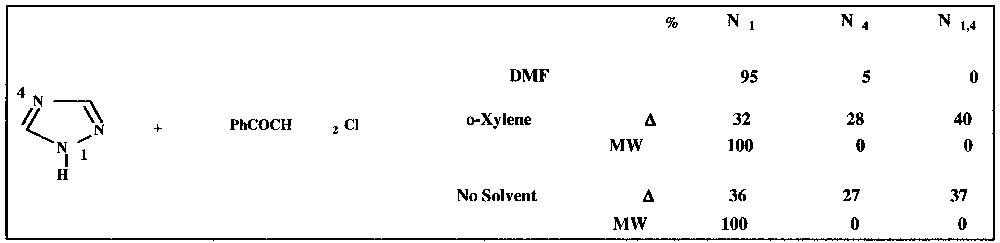
Regioselective phenacylation of 1,2,4-triazole.
It is clear that the same behaviour is obtained under solvent-free conditions plus MW irradiation as when using a polar aprotic solvent such as DMF.
4.2 According to reaction mechanism
Microwave effects result from material–wave interactions and, due to the dipolar polarization phenomenon, the greater the polarity of a molecule (such as the solvent), the more pronounced the microwave effect when the rise in temperature is considered. In terms of reactivity and kinetics, the specific effect has therefore to be considered according to the reaction mechanism and particularly with regard to how the polarity of the system is altered during the progress of the reaction.
Specific microwave effects can be expected for polar mechanisms, when the polarity is increased during the reaction from the ground state towards the transition state. The outcome is essentially dependent on the medium and the reaction mechanism. If stabilization of the transition state (TS) is more effective than that of the ground state (GS), this results in an enhancement of reactivity by a decrease in the activation energy (Fig. 5 ), due to electrostatic interactions (of dipole–dipole types) of polar molecules with the electric field.
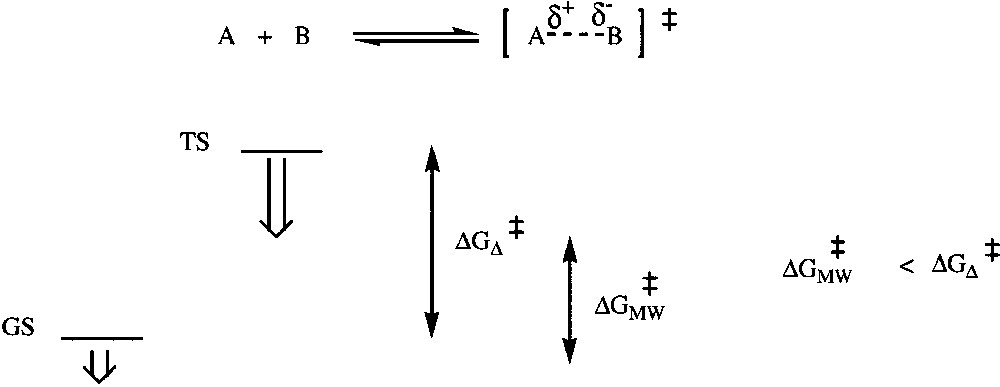
Relative stabilizations of TS (more polar) and GS in a polar mechanism.
MW-materials interactions are increased with the polarity of the system. Therefore, positive MW specific effects could be expected if the polarity of the system is increased during the reaction progress, i.e. if the polarity of the transition state (TS) if greater than for the ground state (GS). The most adapted cases concern thus essentially reactions between neutral molecules via a dipolar TS or anionic reactions involving tight ion pairs in GS that become loose ones in TS (Fig. 6 ).

Microwave effects according to reaction mechanism.
4.3 According to transition state position along the reaction coordinates
The position of the transition state along the reaction coordinates in relation to the well-known Hammond postulate has also to be considered.
If a reaction only necessitates a small activation energy, ΔG≠, the TS looks like the GS (it is depicted as a ‘reactant-like transition state’). Consequently, the polarity is only slightly modified between the GS and TS during the course of the reaction and only weak specific microwave effects can be foreseen under these conditions.
By way of contrast, a more difficult reaction implies higher activation energy. The TS therefore occurs later along the reaction path and, consequently, the influence of polarity effects may be significantly improved. It may be assumed that a microwave effect should be more pronounced when the TS occurs later along the reaction coordinate (depicted more as a ‘product-like transition state’) and is therefore more prone to develop an increased polarity.
5 Some striking examples of solvent-free organic synthesis under microwaves
It has been shown that solvent-free conditions are especially adapted to microwave activation as reactions can be run safely under atmospheric pressure in the presence of significant amounts of products.
In this section, typical and recent examples of the coupling of solvent-free reactions with microwave activation will be described. Comparisons with conventional heating methods realized either in an oil or sand bath previously heated to the same temperature observed in microwave experiments will be given when available.
5.1 Reactions on solid supports [6,17]
Mineral oxides are often very poor conductors of heat, but behave as very efficient microwave adsorbents, this resulting in turn in a very rapid and homogeneous heating. Consequently, they display very strong specific microwave effects, with significant improvements in temperature homogeneity and heating rates, enabling faster reactions and less degradation of final products when compared to classical heating.
Some typical examples are given in Figs. 7 and 8 .

Some typical examples of MW-assisted reactions on solid supports.
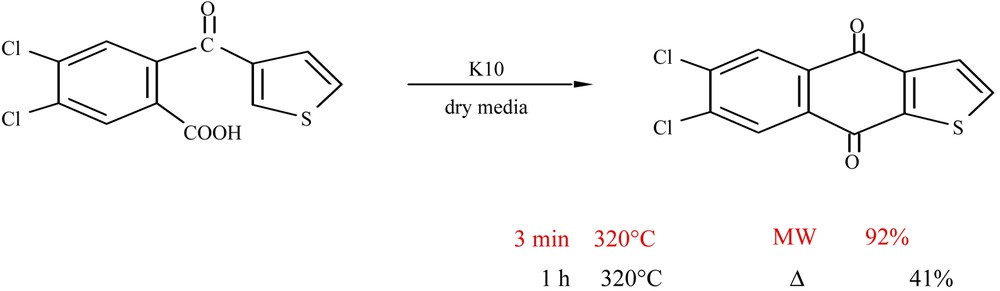
Synthesis of heterocyclic quinones using clay under MW [18].
5.2 Phase-transfer catalysis [19]
Due to ion-pair exchange, there is formation of loose ion pairs Nu–, NR4+ which are very reactive, lipophilic and polar species. They are consequently highly sensitive to MW exposure.
Some typical examples of carboxylate alkylations with long chain halides are shown in Fig. 9 .

Some typical examples of solvent-free PTC under microwaves.
A striking evidence for MW effect according to the difficulty of the reaction lies in the case of saponification of hindered aromatic esters [20]. The reaction with octyl mesitoate only occurs under MW activation in agreement with a very late transition state along the reaction coordinates (Fig. 10 ).

Solvent-free esters saponification.
5.3 Neat reactions without support or catalyst
This type of reactions is illustrated here, as a selected example, by the possibility to realize easily with quantitative yields Leuckart reductive amination of ketones under solvent-free MW radiation [21]. This constitutes an evidence for specific MW activation for a difficult reaction (late transition state) involving a polar mechanism (addition of neutral amino compound on carbonyl moiety via a dipolar transition state) (Fig. 11 ).
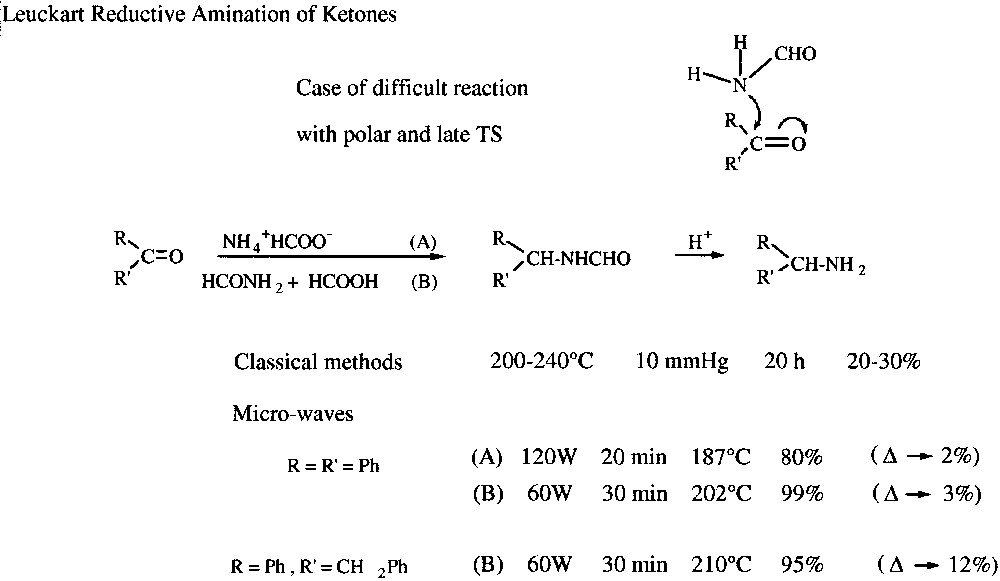
Leuckart reductive amination of ketones under microwaves.
5.4 Some specific examples using biomass derivatives
Within the frame of non-alimentary valorisation of agricultural products, several procedures were foreseen in order to propose new developments for renewable agricultural resources.
5.4.1 Cetalisation of l-galactono 1,4-lactone, a by-product of sugar industry [22]
l-galactono 1,4-lactone is derived from sugar beet root. When reacting with long-chain aldehydes, it leads to long-chain acetals possessing liquid crystal properties. It was possible to improve seriously this reaction in yields from 25 to 89% under Green Chemistry conditions using clay (K10 montmorillonite) with a solvent-free procedure under MW irradiation and within 10 minutes instead of one day (Fig. 12 ).
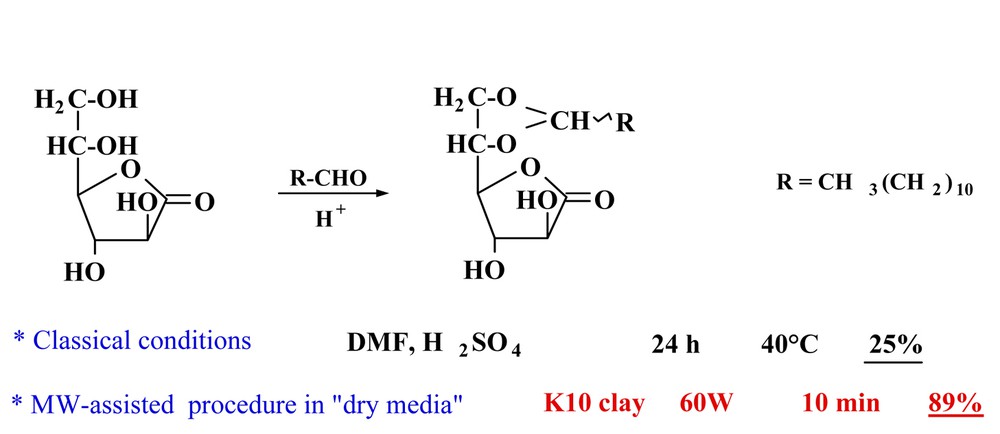
Cetalisation of l-galactono 1,4-lactone under MW green procedure.
5.4.2 Etherification of isosorbide [23]
Among d-anhydrohexitols, isosorbide is one of the most important products of starch industry. It can be transformed in diethers using a solvent-free PTC procedure. Within very short reaction times (5–30 min.), yields are noticeably increased from 10–45% under conventional heating up to 98% under MW activation (Fig. 13 ).

Dietherification of isosorbide under solvent-free PTC conditions
5.4.3 Polyetherification of isosorbide [24]
As an extension of the above study, the synthesis of polyethers was realized by reacting dihalides or dimesylates under similar conditions. Small amounts of toluene were necessary to ensure a good control of temperature and as a diluent for viscous polymers (Fig. 14 ).

Polyetherification of isosorbide under solvent-free PTC conditions.
In addition to the reaction rate increase, MW effects on polymer structures (as determined by MALDI–TOFMS) were observed. Polymers with higher molecular weights with ethylenic groups as chain terminations were thus obtained, unlike to conventional heating, where shorter hydroxylated compounds were achieved.
5.4.4 Glycosylation of monosaccharides [25]
A three-step solvent-free MW-assisted synthesis of decyl d-glucopyranoside with 1-decanol was established for d-glucose and extended to d-galactose, d-mannose with 70% average overall yield for the three steps (peracetylation, glucosylation and saponification). Rate enhancements and reduction in reaction times were observed for the two last steps when carried out under MW irradiation in similar conditions (Fig. 15 ).

A three-step solvent-free MW-assisted procedure for glucose glycosylation.
6 Conclusions
When coupled to microwave irradiation, solvent-free techniques revealed furthermore to be of special efficiency as clean and economic procedures. Serious improvements and simplifications over conventional methods originate from their rapidity, their enhancements in yields and purities of products.
Non-purely thermal specific MW effects can be involved essentially when non-polar solvents or, better, solvent-free conditions, are concerned with polar mechanisms (more polar transition states when compared to their ground states), and for difficult reactions that necessitate high activation energy (late transition state according to the Hammond postulate). In all cases, accurate controls of temperature and microwave emitted power are necessary to ensure reproducible and safe reactions.