1 Introduction
Considerable interest has been devoted to the development of boron and silicon containing inorganic polymers as they posses certain technological advantages as compared to polymers containing boron or silicon exclusively [1,2]. In particular, the SiBCN system has been intensively studied in the past [3–6], while only few papers reported on the SiBCO system [7–9]. For technical reasons the SiBCO system could be produced far cheaper than the SiBCN counterpart. The former material can be obtained by starting from easily accessible poly(organosiloxanes) while the latter one has to be produced from expensive poly(organo-silazanes). Therefore, the knowledge of the SiBCO properties in comparison to that of SiBCN ceramics is of fundamental interest. The first step in this direction is, however, the development of suitable synthetic strategies that allow the production of appropriate preceramic polymers.
Carbon-free borosilicate glasses were prepared by using the sol–gel method based on hydrolysis and polycondensation reactions of metal alkoxides as reported by several authors [7, 9–11]. Despite the numerous studies devoted to SiCO glasses only few papers have been published related to the synthesis of multicomponent silicon oxycarbide glasses [7–9]. In the present study we investigated a novel synthetic approach to prepare preceramic polymers on the basis of poly(organoborosiloxanes), which yield SiBCO ceramics by the polymer-to-ceramic transformation process. The main advantage of such an approach is the formation of polymers with well-defined molecular units controlling the polymer structure. Moreover, the polymer structure determines the solid-state structure and phases of the final ceramic product derived therefrom [12].
2 Results and discussion
The hydroboration reaction of cyclic vinylsiloxanes with borane dimethylsulphide in dry argon atmosphere leads to polymeric SiBCO precursors (Eq. (1)). In particular, the reaction of 1,3,5,7-tetramethyl-1,3,5,7-tetravinylcyclotetrasiloxane with borane dimethylsulphide was performed in n-hexane or toluene as solvent at room temperature. While precipitation of the resulting polymer is observed during the reaction in n-hexane, the product remains soluble in toluene. The different solubility behaviour of the products is an important feature, in particular with respect to application.
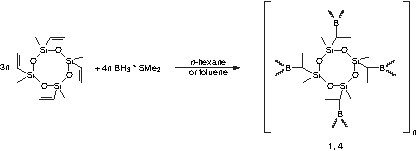
The obtained polymers are colourless powders sensitive against water and air. Variation of the stoichiometric ratio of the educts by increasing the amount of borane leads to polymers with functional boron-hydrogen groups (Eqs. (2) and (3)). These reactive groups can be utilized for further cross-linking reactions.
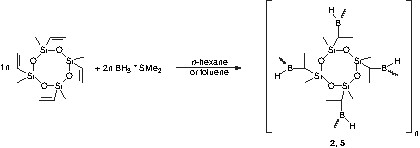
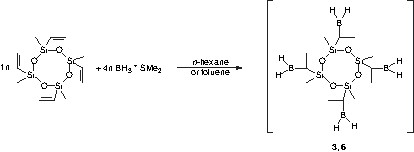
The polyborosiloxanes as synthesized in different molar siloxane/borane ratios are summarized in Table 1. Elemental analysis data of the synthesized polymers 1–6 are listed in Table 2. The results are in agreement with the values calculated with respect to the products expected according to reaction Eqs. (1) and (2). In contrast, polymers 3 and 6 obtained according to the reaction of Eq. (3) reveal systematic discrepancies when comparing the calculated and the found values for the empirical formula. These differences are related to the fact that polymers 3 and 6 cannot be obtained with the ideal molecular structure. Due to further cross-linking via the formation of intermolecular B–C linkages the found composition deviates from that of the ideal structure.
Polymers 1–6 synthesized with different siloxane/borane ratios and in different solvents
Polymer | Molar ratio | Solvent |
siloxane:borane | ||
1 | 3:4 | n-hexane |
2 | 1:2 | n-hexane |
3 | 1:4 | n-hexane |
4 | 3:4 | toluene |
5 | 1:2 | toluene |
6 | 1:4 | toluene |
Elemental analysis of polymers 1–6
Polymer | Molecular formula | Molecular formula |
(calculated) | (found) | |
1 | Si3B1C9H21O3 | Si2.8B1C9.8H23.6O2.9 |
2 | Si2B1C6H15O2 | Si2.4B1C7.7H18.8O2.5 |
3 | Si1B1C3H9O1 | Si1.7B1C5.7H15O1.6 |
4 | Si3B1C9H21O3 | Si2.8B1C9.4H20.3O3 |
5 | Si2B1C6H15O2 | Si2.5B1C8.5H19O2.9 |
6 | Si1B1C3H9O1 | Si2.1B1C7H15.6O2.4 |
FT–IR and Raman spectra of the products 1–6 indicate that the hydroboration reaction was complete as shown for compounds 1–3 in Fig. 1. No absorption bands around 1600 and beyond 3000 cm–1, which could be assigned to residual vinyl groups [13–15], are found.
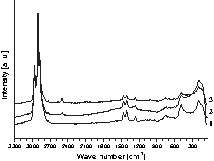
Raman spectra of the synthesized polymers 1–3.
The presence of B–H bonds could be clearly identified by Raman spectroscopy for compounds 2 and 3 as well as for 5 and 6 (see Eqs. (2) and (3)). In contrast to polymer 1, an additional vibration mode is found for polymers 2 and 3 at 2500 cm–1, as can be seen from Fig. 1. This absorption band agrees well with that of B–H bonds, as described in the literature [16].
The intensity of the B–H Raman bands increases with increasing amount of B–H bonds in the polymer. The spectroscopic investigation of polymer 4 proofs that no B–H bonds are present, which is in line with Eq. (1). Further characteristic bands are found between 100 and 1500 cm–1 as well as between 2800 and 3000 cm–1. The former absorptions are assigned to Si–O–Si, Si–O, and Si–C while latter vibrations are due to aliphatic C–H bonds.
The thermal behaviour of the reaction products was studied in detail with polymer 4 between room temperature and 1100°C by thermal gravimetric analysis (TGA) in combination with in situ mass spectrometry. The results are presented in Figs. 2 and 3.
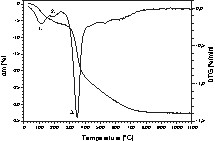
Thermal gravimetric analysis (TGA + DTG) of polymer 4.
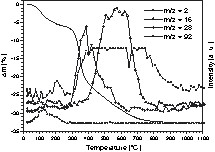
Thermal gravimetric analysis with in situ mass spectrometry of polymer 4; m/z = 2 [H2]+, m/z = 16 [CH4]+, m/z = 28 [C2H4]+, m/z = 92 (toluene). The signal m/z = 2 shows an overflow due to the used sensitivity of the intensity range.
Accordingly, polymer 4 synthesized in toluene shows three weight loss steps. The first step between room temperature and ~ 150 °C is due to the loss of residual toluene (~ 2 wt%) as analysed by in situ mass spectrometry (see peak m/z = 92 in Fig. 3). Steps two and three are related to the evolution of hydrogen (m/z = 2) and methane (m/z = 16) beyond 200 and 400 °C, respectively. In addition, the signal m/z = 28 is found between 250 and 650 °C and is identified as C2H4. Considering that the signals m/z = 27 and 26 (not shown in Fig. 3) were also detected in the same temperature range as that of m/z = 2 and 28 proofs the formation of volatile C2H4 as gaseous by-product. Moreover, the absence of the peak m/z = 44 related to CO2 indicates that signal m/z = 28 cannot be related to the evaporation of CO. The thermal decomposition is completed at around 800 °C.
The loss of C2H4 and CH4 during polymer decomposition significantly reduces the amount of carbon in the final ceramic product obtained at 1100 °C. The overall carbon content is an important feature of the material since it controls the electrical behaviour of the SiBCO ceramic. Polymer 1 synthesized in n-hexane instead of toluene as solvent reveals an identical thermal behaviour.
The precursor-to-ceramic conversion process was carried out in an inert argon atmosphere. The structural changes between the polymer and the inorganic solid-state were analysed by Raman spectroscopy. The spectra show bands located at 1350 and 1600 cm–1, which are characteristic for the two main bands of amorphous or graphite-like sp2-carbon and diamond-like sp3 entities. This finding clearly indicates that amorphous or graphite-like carbon has been separated from the SiBCO matrix.
The crystallization behaviour and phase separation induced by annealing of the sample beyond 1100 °C were determined by means of X-ray powder diffraction. These investigations show that the ceramics are X-ray amorphous up to 1200 °C and phase separation is found to be pronounced at T > 1300 °C (Fig. 4). As the crystalline phase, β-SiC is formed exclusively. While in boron-free SiOC ceramics the formation of cristobalite is found at higher temperatures [17], crystalline silica phases could not be detected in the SiBCO materials annealed between 1200 and 1500 °C.

X-ray powder diffractograms of SiBCO ceramic derived from polymer 4 subsequently heat-treated for 5 h in Ar at different temperatures.
Compositional changes during annealing were detected by elemental bulk analysis. As can be seen from Table 3, the carbon and oxygen contents remain nearly constant up to 1300 °C. Furthermore, it is evident from Table 4 that the silicon-to-boron ratio remains almost constant during annealing of the SiBCO ceramic up to 1300 °C. At temperatures exceeding 1300 °C, a significant drop of the amount of oxygen from around 20 wt% to 0.3–1.8 wt% is analysed, and an increase in the silicon-to-boron as well as silicon-to-carbon ratio is found. Thus, the mass loss between 1300 and 1500 °C indicates decomposition of the SiBCO matrix caused by the evaporation of volatile B–O and C–O species. Consequently, the material formed at 1500 °C has to be considered as polycrystalline β-SiC embedded in an amorphous residual SiBC(O) matrix.
Elemental analysis of the ceramics 1–6 heat-treated up to 1500 °C.
Polymer | Si [wt.%] | B [wt.%] | C [wt.%] | O [wt.%] | Si [wt.%] | B [wt.%] | C [wt.%] | O [wt.%] | Si [wt.%] | B [wt.%] | C [wt.%] | O [wt.%] |
1100 °C | 1300 °C | 1500 °C | ||||||||||
1 | 39.60 | 4.41 | 30.45 | 25.60 | 40.20 | 4.34 | 31.26 | 22.44 | 64.25 | 4.66 | 27.94 | 0.61 |
2 | 38.45 | 5.75 | 31.25 | 24.85 | 35.65 | 4.92 | 25.85 | 23.98 | 62.70 | 6.21 | 28.41 | 0.45 |
3 | 37.70 | 6.92 | 32.05 | 24.10 | 38.95 | 6.79 | 30.89 | 19.16 | 61.50 | 7.33 | 27.77 | 0.32 |
4 | 38.20 | 4.70 | 32.68 | 22.41 | 40.65 | 4.04 | 30.28 | 17.35 | 63.75 | 4.45 | 28.24 | 1.33 |
5 | 37.80 | 5.39 | 32.78 | 22.40 | 48.50 | 4.43 | 30.70 | 9.62 | 60.90 | 6.48 | 28.86 | 1.76 |
6 | 37.75 | 6.40 | 32.92 | 21.46 | 39.35 | 5.62 | 27.51 | 20.69 | 60.15 | 8.50 | 28.31 | 1.17 |
Comparison of the molecular formula of the polymers 1–6 and the ceramics derived therefrom
Molecular formula | ||||
Polymer | Ceramic / 1100 °C | Ceramic / 1300 °C | Ceramic / 1500 °C | |
1 | Si2.8B1C9.8H23.6O2.9 | Si3.5B1C6.2O3.9 | Si3.6B1C6.5O3.5 | Si5.3B1C5.4O0.1 |
2 | Si2.4B1C7.7H18.8O2.5 | Si2.6B1C4.9O2.9 | Si2.8B1C4.7O3.3 | Si3.9B1C4.1O0.1 |
3 | Si1.7B1C5.7H15O1.6 | Si2.1B1C4.2O2.4 | Si2.2B1C4.1O1.9 | Si3.2B1C3.4O0.1 |
4 | Si2.8B1C9.4H20.3O3 | Si3.1B1C6.3O3.2 | Si3.9B1C6.8O2.9 | Si5.5B1C5.7O0.2 |
5 | Si2.5B1C8.5H19O2.9 | Si2.7B1C5.5O2.8 | Si4.2B1C6.2O1.5 | Si3.6B1C4O0.2 |
6 | Si2.1B1C7H15.6O2.4 | Si2.3B1C4.6O2.3 | Si2.7B1C4.4O2.5 | Si2.7B1C3O0.1 |
3 Conclusions
New polyborosiloxane precursors have been synthesized by the hydroboration reaction of 1,3,5,7-tetramethyl-1,3,5,7-tetravinylcyclotetrasiloxane with borane dimethylsulphide using different molar ratios and solvents. The associated SiBCO ceramics were obtained by pyrolysis of these polymers in inert Ar atmosphere at 1100 °C.
FT–IR and Raman spectroscopy indicate that the hydroboration of the vinyl groups was complete. With increasing the amount of borane, the Raman spectra reveal characteristic B–H bands at 2500 cm–1 after reaction with the siloxane.
Thermal gravimetric analyses show that the decomposition of the polymers occurs in three weight loss steps, which are due to (i) the loss of solvent followed by (ii) and (iii) the release of H2, CH4 and C2H4. Raman spectroscopy and X-ray powder diffractometry reveal that the obtained ceramics are amorphous up to 1200 °C. Carbon is found in form of an amorphous or graphite-like phase. Enhanced phase separation with formation of crystalline β-silicon carbide starts at temperatures exceeding 1300 °C and is correlated with the decomposition of the SiBCO matrix.
One main feature of the synthesized SiBCO system is that cristobalite formation can be avoided, which is important for technical reasons. Phase transformations associated with crystalline SiO2 (cristobalite) during cooling and heating may lead to matrix or surface cracking deteriorating the mechanical strength and/or oxidation resistance of the material.
4 Experimental procedures
4.1 General comments
All reactions were carried out in a purified argon atmosphere using standard Schlenk techniques (all equipment is provided with side-arms for pumping out air and moisture and introducing inert gas; see also [18]). 1,3,5,7-Tetramethyl-1,3,5,7-tetravinylcyclotetrasiloxane was purchased from ABCR (Karlsruhe, Germany). Borane dimethylsulphide complex was obtained from Aldrich (Milwaukee, USA). All chemicals were used as received without further purification.
4.2 Synthesis of the polymers
The polyborosiloxanes were prepared according to the following synthetic route: In a 1 L Schlenk flask equipped with a magnetic stir bar, 10 ml of 1,3,5,7-tetramethyl-1,3,5,7-tetravinylcyclotetrasiloxane (10 g, 29 mmol) were solved in abs. n-hexane or toluene and cooled to 0 °C. After stirring for 30 min, the borane dimethylsulphide in 50 ml solvent was added slowly. After stirring for further 20 h at room temperature the solvent was removed by distillation under reduced pressure at 40 °C. The products were dried at room temperature and under reduced pressure (2 × 10–2 mbar), and finally isolated as colourless powders. Table 1 shows the used molar ratios and solvents.
4.3 Characterization techniques
The polymeric structure was investigated using Fourier transform infrared (FT–IR) and Raman Spectroscopy. FT–IR spectra were recorded on a Perkin Elmer FT-IR 1750 using a diamond single reflection ATR unit from Specac Inc. (UK), and the Raman spectra were collected on a Bruker FT Raman Modul FRA 106 equipped with a germanium detector (D 418-S) and 1064 nm laser excitation source. Thermal analyses were performed with a Simultaneous Thermal Analyzer STA 429 (Netzsch, Germany). The gases evolved during pyrolysis were detected with a Balzers QMA 400 mass spectrometer coupled to the STA equipment. TGA experiments were performed at a heating rate of 5 °C min–1 under argon. The chemical composition of the polymers as well as the Si and B contents of the ceramics were investigated by Mikrolabor Pascher, Remagen, Germany. The C and O content of the ceramics were measured by hot gas extraction using a NO-Analyzer Leco TC-436 and C-Analyzer Leco C-200, respectively.
4.4 Ceramic conversion process
Pyrolysis experiments of the polymers were carried out in a GERO tube furnace equipped with an internal quartz glass shuttle under continuous argon flow. The samples were heated to 1100 °C, with 100 °C min–1 and held for 5 h at this temperature. Finally, the samples were cooled down to room temperature at 200 °C min–1.
Crystallization of the amorphous SiBCO ceramics was studied by annealing of the samples at temperatures between 1200 and 1500 °C for 5 h in argon. The heat treatment was performed in a resistant heated graphite furnace.