1 Introduction
Molecular aggregation in structured non-aqueous solvents has received little attention compared to the number of investigations in water. The unique properties of water (polarity, protic, and highly structured) have led to the widespread use of aqueous systems. Hydrophobic interactions and internal pressure effects have been used to stabilize these systems [1].
However, many organic reactants are not easily solubilized in water. Moreover, dielectric constants in microenvironments, with their localized interfacial properties, are lower than in water and are closer to those found in salt solutions or in methanol.
These considerations have led to a requirement for non-aqueous solvents which will enhance solubility and reactivity in such non-homogeneous media.
2 Specifications for a water substitute
Two approaches can be employed, one based on theoretical considerations of the physicochemical properties of water, and the other based empirically on the results of experiments.
2.1 Physicochemical properties
The hydrophobic interactions observed in aqueous systems are in fact a particular example of solvophobic interactions in general. The ability of some ionic amphiphilic molecules to form micelles in non-aqueous media indicate that a water replacement would have the following properties:
- i) high polarity: the dielectric constants must be sufficiently high to dissociate ions of amphiphilic molecules and hinder self-associations;
- ii) high solvating power: this, in combination with the high dielectric constant, favors dipole interactions over associations between opposite charge ions;
- iii) highly structured: the notion of structure is based on the strength of intermolecular bonds and the geometry of the molecules. Both chemical (hydrogen bonds) and non-chemical (polar and non-polar) interactions must be taken into account.
The structured nature of a solvent can be evaluated from two main parameters:
- - the internal pressure Pi,
- - the density of the cohesion energy Dce.
Initially, solvents forming hydrogen bonds were chosen, although this is not an absolute requirement if aprotic molecules could be found of sufficient polarity (dipole) to structure the medium.
Tables 1 and 2 show physicochemical parameters for various potential solvents.
Physicochemical characteristics of various solvents
Solvents | Dcea J cm−3 | Pib J cm−3 | ɛ/ɛoc | μDd | ETe kcal mol−1 |
Water | 2302 | 150.6 | 78.5 | 1.8 | 63 |
Glycerol | 1142.4 | 46 | 2.6 | ||
Formamide | 1574.9 | 554.4 | 109.5 | 3.4 | 56.6 |
Ethylène glycol | 892.0 | 502.1 | 37.7 | 2.0 | 56.3 |
Methanol | 873.6 | 288.3 | 32.6 | 1.65 | 55.5 |
N-Methyl formamide | 641.6 | 182.4 | 3.8 | 54.1 | |
N-Methyl acetamide | 532.5 | 175.7 | 4.3 | 52.0 |
a .
b Internal pressure.
c Dielectric constant.
d Charge dipole moment.
e Kosower's parameter.
Surface tension of various solvents
Solvents | γ mN m−1 (T °C) | Solvents | γ mN m−1 (T °C) |
Water | 70 (20) | Methanol | 22.55 (20) |
Glycerol | 63.4 (20) | ||
Formamide | 58.5 (20) | N-Methyl formamide | 37.96 (30) |
Ethylene glycol | 48.9 (20) | N-Methyl acetamide | 33.67 (30) |
46.3 (50) Et3 NH+ NO−3 |
Examination of these parameters suggested that formamide would be suitably structured.
2.2 Empirical observations
These are based on the presence of molecular association in different solvents. Sinanoglu and Abdulnur [2] established the following order of solvophobicity:
Water > glycerol, formamide > > ethylene glycol > butan-1-ol, methanol, propan-1-ol, ethanol > t-butanol. |
This order is also found for the stability of DNA double helices in the same solvents [3]. It can be seen that formamide (and glycerol) stands out from the other non-aqueous solvents. This has also been confirmed by X-ray diffraction, showing the highly structured nature of liquid formamide [4] (Scheme 1).

Structure of formamide (Ref. [2]).
3 Formamide, a water substitute
We therefore carried out a comparison between water and formamide in various situations:
- 1. In a structural investigation of molecular associations (micellizations, formation of microemulsions, liquid crystals…)
- 2. In various reactions in phase transfer catalysis [5] or in microemulsions.
3.1 Self-associations in formamide
Several studies on micellization in this solvent have been carried out [6] although for the SDS/formamide system, Almgren et al. [7] report that they have been unable to detect SDS micelles in formamide at 25 °C.
3.1.1 Micellization
It is well known that micellization only takes place above the Krafft temperature for ionic surfactants. We showed that the Krafft temperature itself depends both on the nature of the surfactant and the medium: it was the reason why Almgren was not able to have micellization at 25 °C. Table 3 shows Krafft temperatures and cmc in water and formamide for two ionic surfactants [8].
Krafft temperature and cmc in water and formamide for two ionic surfactants
Surfactant | Formamide | Water | ||
Krafft temperature (°C) | cmc (mol l−1) | Krafft temperature (°C) | cmc (mol l−1) | |
CTAB | 43 | 9 × 10−2 (50 °C) | 26 | 1.03 × 10−3 (45 °C) |
SDS | 55 | 2.2 × 10−2 (60 °C) | 16 | 9.7 × 10−3 (45 °C) |
Micelles of ionic surfactants in formamide can only be formed above the Krafft temperature (higher in formamide than in water). Under these conditions, the cmc values are also higher in formamide than in water (for both ionic and non-ionic surfactants). Formamide would seem to confer the properties of a low melting anhydrous salt similar to triethylammonium nitrate (Fig. 1):
- i) X-ray scattering was used to analyse the structures of aggregates of surfactants in various formamide-containing media.
CTAB micelles were detected in formamide solutions. A spherical model was proposed at values close to the cmc, smaller micelles were observed in formamide than in water. They remained spherical up to at least 2.5 times the cmc and they were observed over the temperature range 40–70 °C [9]. The absolute scattering intensities were measured on 2.88% (close to the cmc), 5%, 10% solutions (by weight): from 5% to 10% the aggregation number increases from 7 to 24
- ii) Molecular motions in the system CTAB/FA have been studied by proton, N-14 and C-13 nuclear magnetic longitudinal relaxation at different frequencies, with the reference to the well known system CTAB/D20. These measurements confirmed the presence of spherical micelles at 60 °C. From all the parameters the following structure and dynamic picture of micelles in formamide emerges:
- - micelles are spherical,
- - the micelle interior is more organized in formamide than in water, whereas significantly less structure is found at the micelle interface which is presumably perturbed by solvent penetration [10].
- iii) The phase diagram of CTAB in formamide was determined by observation with a polarizing microscope [11]. Two mesomorphic phases, a hexagonal and a lamellar, were observed which closely resembled these found in CTAB/water system.
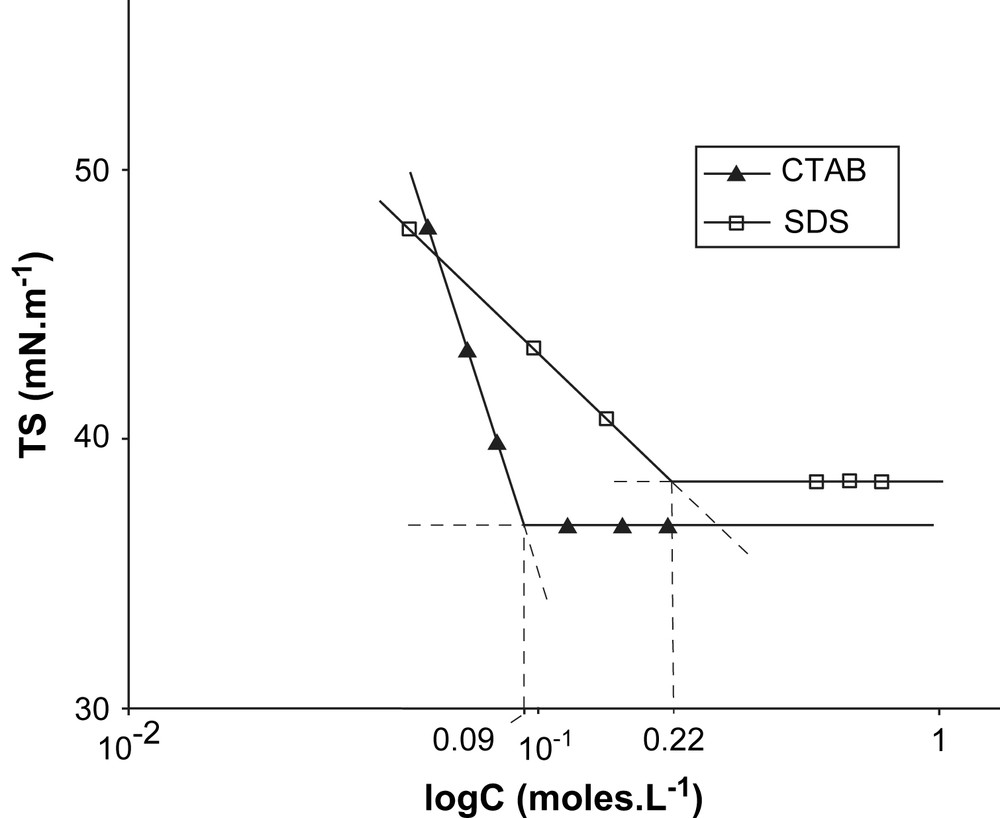
Measurement of cmc of CTAB and SDS solutions in formamide.
In order to compare the effects of formamide on the existence of intermediate phases, a small-angle X-ray scattering set up was used to observe the diffraction peak corresponding to the high values of the phase parameters and their transformation. The X-ray findings added extra details to the CTAB/FA diagram which was initially established by optical microscopy [12]. The following sequence of intermediate phases was identified as well as in CTAB/water system, than in CTAB/FA:
There are:
- i) a difference of 25–30 °C between the formation of the liquid crystal phase;
- ii) a more extended domain of stability of hexagonal phase in water than in formamide.
These results show that mesophases of identical symmetry was observed in FA and water with closely similar lattice parameters. Thus these solvents have similar capability to form lyotropic mesophases.
The micellization of SDS in formamide has been evidenced by measurement of surface tension above 55 °C [8] and verified by Raman spectroscopy. As with CTAB the cmc is much higher in formamide than in water (Table 3).
The formation of micelles of SDS has been demonstrated beyond doubt by small angle neutron scattering (SANS) [13] the spherical micelles formed are smaller than those observed in water: a value of 15–27 molecules with considerable dispersion being observed experimentally. The study demonstrated a progressive self-association of molecules: the growth of micelles is not compatible with a pseudophase model and the mechanism of self-association is in line with the model of multiple equilibrium. Such self-association occurs after spontaneous micellization and contributes to the increase of the micelles size.
The lyotropic liquid crystals formed by SDS in formamide were investigated by using small-angle X-ray diffraction and optical microscopy [14] (Fig. 2). It was interesting to compare with the behavior of the SDS/water system, in this case complex phases were observed:
Hexagonal (p6m) ↔ centered rectangular (cmc) ↔ rhomhoedral ↔ centered tetragonal ↔ lamellar |
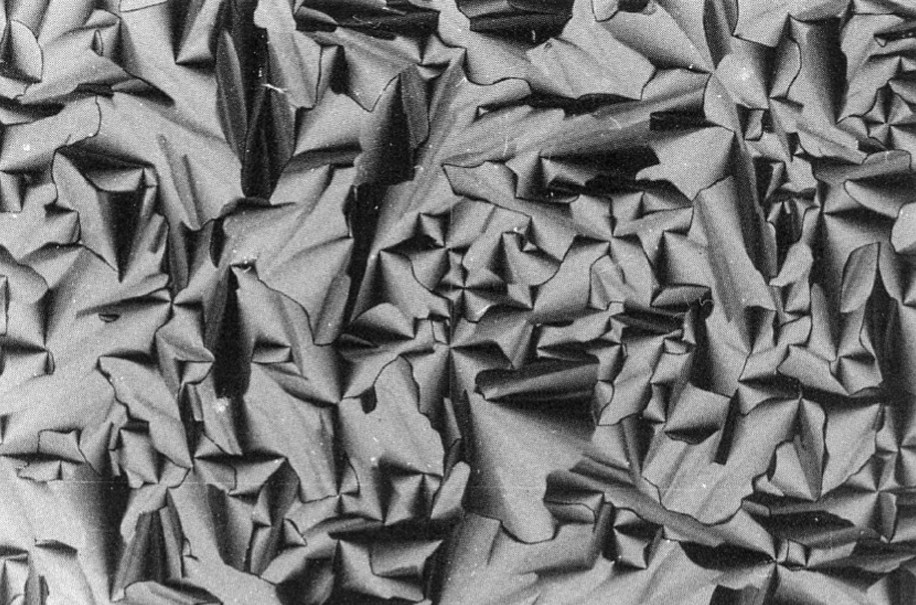
Lyotropic liquid crystals formed by SDS in formamide.
The rhomhoedral structure turns into a cubic phase Im3n.
The conventional sequence of ordered lyotropic phases predicted by Charvolin and Sadoc [15] has been observed on the binary system of SDS in formamide which exhibited the following sequence:
Hexagonal ↔ cubic (Ia3d) ↔ lamellar |
The complexity of the aqueous system compared to the simpler SDS/formamide system is possibly due to the fact that SDS is stable in formamide but not in water in which the following reactions ((I) and (II)) are always existing:
C12H25OSO3− + H2O ⇆ C12H25OSO3H + OH− (I) |
C12H25OSO3− + H2O → C12H25OH + HSO4− (II) |
4 Micellization in formamide of water-insoluble amphiphilic compounds
Two types of water-insoluble amphiphilic compounds have been studied [16]:
- 1) ionic surfactants represented by long chain phosphonium salts prepared by heating triphenyl phosphine with various alkyl, or fluoroalkyl, iodides at 95 °C in the absence of solvent:
Yields: 25–92%.Ph3P + ICH2CH2R → Ph3P+–CH2CH2R, I− Table 4 shows that micellization occurs in FA with these phosphonium salts.
- 2) Mesoionic surfactants represented by long chain alkylazides prepared by the method described by Reeves and Bahr [17] involving a liquid/liquid phase transfer reaction in water or formamide:
R = n-C5H11, n-C8H17, n-C10H21, n-C6F13, n-C8F17X = Br, I.
cmc of phosphonium salts in formamide at 64 °C, determined from measurement of surface tension
R | cmc (mol dm−3) |
C6F13 | 0.156 |
C8F17 | 0.029 |
C10F21 | 0.011 |
C6H13 | 1.030 |
C8H17 | 0.800 |
C10H21 | 0.383 |
Measurement of the surface tension of R–C2H4–N3 in formamide at 40 °C demonstrated the surfactant properties and the existence of micellization of these long chain azides in this medium (Table 5).
cmc of mesoionic azide compounds in formamide at 40 °C determined from measurement of surface tension
R | cmc (mol dm−3) |
C5H11 | 0.125 |
C8H17 | 2.3 × 10−2 |
C10H21 | 6 × 10−3 |
The long chain fluoroalkylazides are not soluble in FA illustrating the hydrophobicity of perfluorinated groups. These compounds thus have no surfactant action in this solvent in contrast to the long chain phosphonium salts.
We have been able to extend these results to zwitterionic amphiphiles: N-alkylbetaïnes, β-alanine betaïnes and γ-butyrobetaïnes [18]; all betaïnes micellize in formamide (Table 6)
Values of cmc for the betaïnes CH3(CH2)nN+(CH3)2–(CH2)m–CO2−, at 60 °C by measurement of surface tension
Water | Formamide | |||||
n | Series 1 m = 1 | Series 2 m = 2 | Series 3 m = 3 | Series 1 m = 1 | Series 2 m = 2 | Series 3 m = 3 |
11 | 1.4 × 10−3 | 7 × 10−1 | ||||
13 | 1.7 × 10−4 | 6.9 × 10−4 | ||||
14 | 7.8 × 10−5 | 4.2 × 10−4 | 6.2 × 10−2 | 5.3 × 10−2 | ||
15 | 3.8 × 10−5 | 8.3 × 10−5 | 1.6 × 10−4 | 2.9 × 10−2 | 5 × 10−2 | 2.1 × 10−2 |
17 | 8.6 × 10−6 | 2.2 × 10−5 | 4 × 10−5 | 6 × 10−3 | 7.9 × 10−3 | 6 × 10−3 |
Owing the properties of betaïne giving micelles in water and formamide it was an excellent example to compare their aggregative behavior. Self-association of these amphiphiles in non-aqueous solvents was accounted for in terms of interactions between the polar heads of the molecules, solvophobic interactions and solvation.
For a given homologous series of surfactants the cmc can be expressed in terms of the following relationship:
In formamide, as in water, there is a linear relationship between log (cmc) and the length of the respective incremental contribution a and b of the polar heads and CH2 groups to micellization. The values of these parameters an listed in Table 7
Values of parameters (a) and (b) representing the contribution of polar head and CH2 groups, respectively, to micellization in water and formamide
Surfactants | Water | Formamide | ||
a | b | a | b | |
Betaïnes | 0.9 | −0.32 | 3.58 | −0.33 |
Alkylazides | 0.9 | −0.25 |
It can be seen that parameter b was essentially the same in both solvents: this shows that solvophobic interactions in formamide are similar to those in water. In contrast, the parameter a is different.
Since the solvophobic interactions are identical, the interaction between the polar heads of the surfactants would tend to have the greatest influence on micellization. The high value of a in formamide can be accounted for by interaction stemming essentially from solvation. In water, micellization involves mainly the formation of hydrogen bonds with water, whereas dipole/dipole interactions predominate in formamide: these interactions are more difficult to disrupt than hydrogen bonds. Since the formation of micellar aggregates depends on the nature of the surfactant, the nature of the solvent and largely on the interaction between the two, production of micelles hinges on the optimal choice of the surfactant/solvent pair.
5 Formamide microemulsions
Microemulsions have attracted considerable interest in view of their potential uses, especially as a medium for chemical reactions [19], in photochemistry [20], for enhanced oil recovery, storage of solar energy, drug delivery, etc.
Macroscopically microemulsions are transparent dispersions of two immiscible fluids (water and oil) which are formed spontaneously by admixing certain amphiphilic molecules.
A typical system contains four substituents: water, oil (hydrocarbon, fluorocarbon…) surfactant (ionic, zwitterionic or non-ionic) and cosurfactant (short chain alcohols, amines and also oximes).
In all cases, water would seem to be required.
It occurred to us that it should be possible to prepare microemulsion with formamide instead of water. Thus we have reported some four component systems where the oil was cyclohexane, the structured solvent was formamide, and the cosurfactant was butan-1-ol [21].
Two surfactants were studied:
- - cetyltrimethylammonium bromide (CTAB)
- - cetyltributylphosphonium bromide (CTbPB).
For CTAB, we found similar monophasic areas to those obtained with water (Fig. 3)
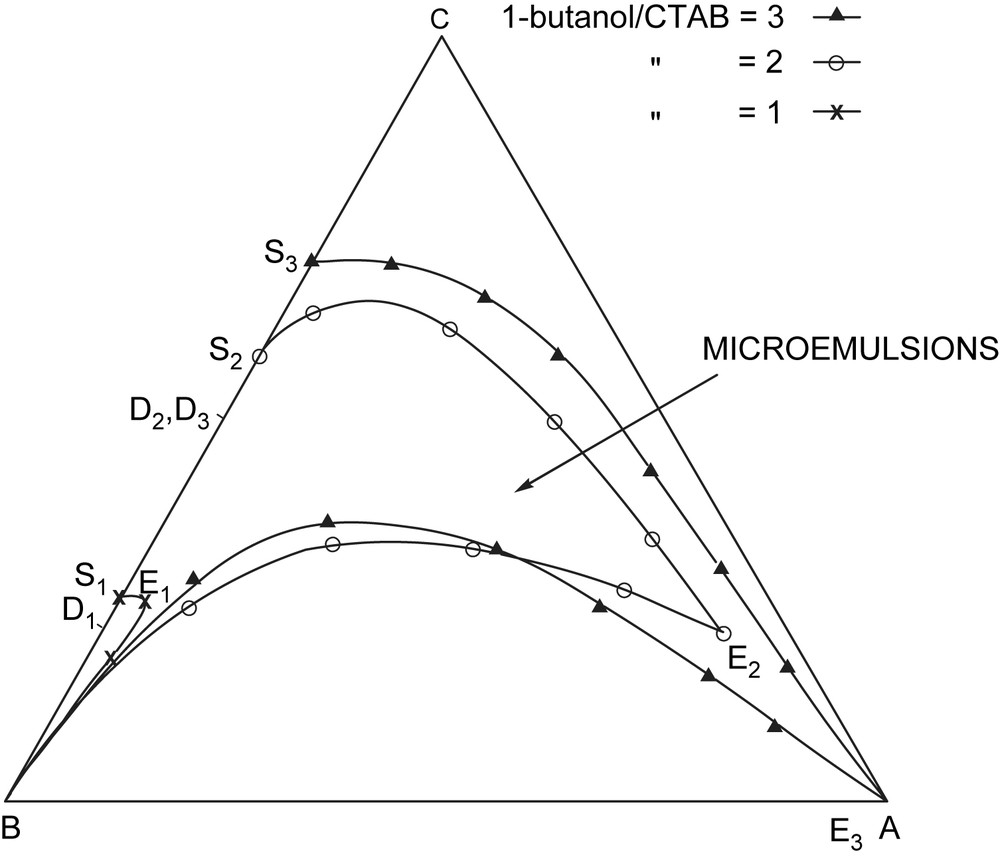
Microemulsions with formamide instead of water. A: cyclohexane; B: formamide; C: 1-butanol/CTAB.
Electric conductivity measurements along the phase boundary (extrapolation of the linear portion of the dilution curve) showed there was a discontinuity in electrical conductivity. This discontinuity indicated a percolation phenomenon for a volume fraction of formamide ∅ = 0.02 (Fig. 4)
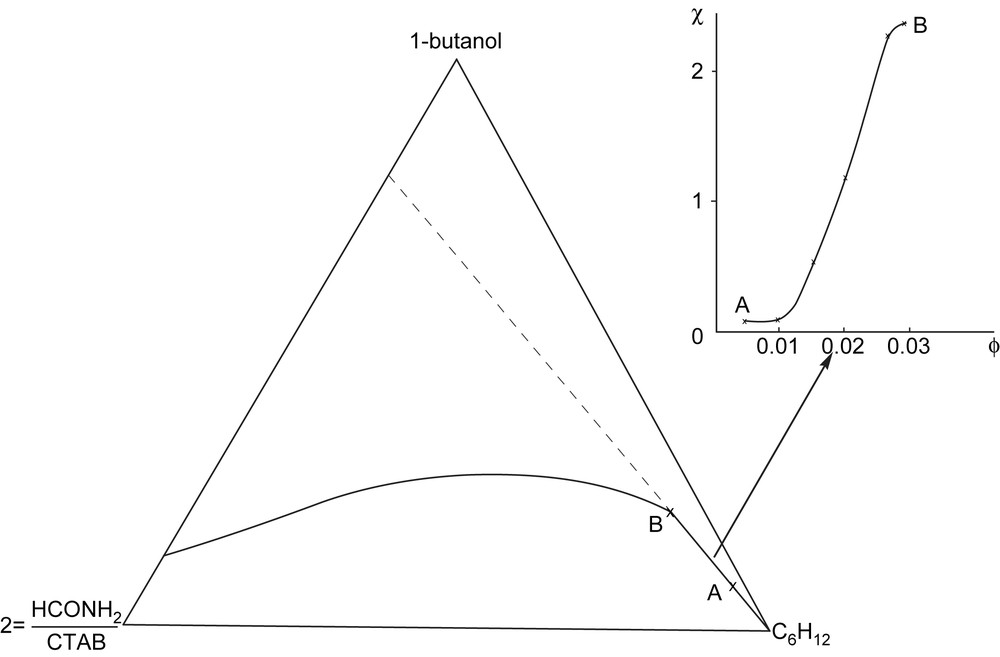
Electric conductivity measurements along the phase boundary.
It is noteworthy that no microemulsion was obtained with CTbPB and water. In formamide with this surfactant we have been able to obtain large monophasic areas in the pseudoternary diagram of (butan-1-ol/CTbPB = 3,2,1, formamide, cyclohexane).
X-ray scattering was used to analyse the structures of microemulsions of the system (formamide/CTAB = 2, isooctane, butan-1-ol) in the isooctane-rich zone: the pseudoternary phase diagram looks like the one precedently described with cyclohexane. Nevertheless this analysis has shown that the microemulsions obtained in formamide are quite different to those observed in water-in-oil microemulsions. Dispersed formamide filaments were observed, rather than the globular particles seen in aqueous microemulsions [22].
It was also possible to prepare large continuous monophasic areas of perfluorinated microemulsions by using formamide in place of water [23]. The systems studied consisted of quaternary mixtures of formamide:
- - potassium 2,2,3,3-tetrahydroperfluoro undecanoate as surfactant,
- - cosurfactant: 1,1,2,2-tetrahydroperfluorohexanol,
- - and perfluorinated oils: perfluorodecaline; 1,1,2-trihydroperfluoro-1-decene (C8F17CHCH2) and 1,1,1,2,2-pentahydroperfluoro decane (C8F17C2H5).
Electrical conductivity measurements showed a discontinuity in electrical conductivity along the phase boundary probably due to a percolation phenomenon for a value fraction of formamide ∅ = 0.12.
For perhydrogenated oils we found only ∅ = 0.02. This high value for perfluorinated microemulsions is perhaps due to the fact that perfluoro micelles are smaller than perhydrogenated ones. More micelles are therefore necessary for percolation with a greater quantity of surfactant and formamide. It is also another example of different behavior in water and formamide.
5.1 Vesicles and liposomes formation in formamide
The most widely studied vesicle systems are prepared in water from lecithins (liposomes) or double-chain surfactants (vesicles). It has been reported that phospholipids form bilayers in both glycerol [24] and N-ethylammonium nitrate [25]. We have also been able to observe formation of bilayer systems, vesicles and liposomes in formamide.
Bilayer membranes were obtained after dispersion of dodecylakyldimethylammonium bromide in formamide:
(C12H25)(CnH2n+1)N+ (CH3)2 Br− |
Vesicles were observed in suspension of C12Cn (0.2 M) in formamide for n > 0.9; results were similar to those obtained in water, but at higher concentration (0.2 M vs. 0.1 M) and with longer sonication times than those required in water (30 min vs. 5 min) multilamellar vesicles with a diameter of 1000–5000 Å and a layer thickness of 2 nm, rather smaller than those obtained in water (4 nm) were observed [26]. The vesicles grew rapidly, but polydispersion was observed in the light-scattering studies 24 h after preparation.
Thanks to a probe reaction (decarboxylation of 6-nitrobenzisoxazole) in the dodecylalkyldimethylammonium bromide/formamide system, we have been able to determine the phase transition temperature Tc: around 40 °C for C9C12 and slightly over 41 °C for C10C12. These values are higher than those for similar surfactants in water (28 °C for C14C14).
A bilayer was not formed as the second hydrophobic chain is shorter than C9: in this case, only the micellar phase is formed. These results show that, in order to form a bilayer and hence vesicles, the side chain must be above a certain length: in our series, this was observed at C9.
By using the method normally employed for preparation of liposomes (of the MLV type) in water, we obtained evidence that lecithin (Lipoid 80) will form multilayered liposomes in formamide: the liposomes were found to be stable for more than 2 weeks [27].
The sizes of liposomes were found to be larger in formamide than in water (500 nm vs. 100 nm). A part from this size difference, it should be noted that the layer thickness was 4 nm in formamide and between 7 and 10 nm in water (measured on electron micrographs).
Our findings are in line with those observed for conventional monocatenary surfactants in formamide. In all cases, the structural parameters are smaller in formamide than in water, which can be accounted for by the fact that formamide is less structured than water and induces weaker short range repulsive interactions.
6 Reactivity in organized molecular systems in FA
Various studies have shown the value of aqueous microemulsions as reaction media although their scope has been limited by the lack of solubility of many reagents in water. Formamide microemulsions was particularly useful in such reactions. Among the different organic synthesis we realized, we will indicate:
- - Diels–Alder reaction between methyl acrylate and cyclopentadiene [28]. Using this cycloaddition we were able to explore the monophasic areas of both ionic and non-ionic surfactants solution in formamide. From the values of the endo/exo ratios observed, there is a discontinuity in reaction selectivity indicating the presence of structured phases which would have been impossible to detect by measurement of density;
- - amidation of olefins by gamma irradiation and light-induced addition [29];
- - Wacker process for the catalytic oxidation of alkenes [30];
- - chemical decontamination of nerve gas [31].
7 Conclusion
At the question “Is formamide a water like solvent”, we answer: yes! In the field of organized molecular systems, we have been able to obtain colloidal aggregates having similar shapes and functions than with water. The differences we observe are essentially quantitative and sometimes interesting: it is possible to use new amphiphilic molecules soluble in FA but not in water. From a mechanistical point of view the use of FA will be a new tool for fundamental studies of this so important domain of, not only chemistry, but also physics and biology.
Acknowledgements
X-ray scattering studies have been realized by Professor C. Petipas and Dr. X. Auvray at the University of Rouen. We would like to thank very much our French colleagues for their kind and fruitful collaboration.