1 Introduction
Reactions have been studied in organized molecular media since the 1970s. There is currently much research in this area and the scientific literature on the topic is vast. For example, organic synthesis has been investigated extensively as reflected in monographs and review articles [1].
In recent years, microemulsions have been shown to be useful as media for a wide range of chemical reactions [2], such as bioorganic synthesis [3], preparation of nanoparticles [4], polymerisation [5], organic synthesis [6], metal-ligand complex formation [7], and enzyme processes [8]. Because the topic is now a mature field of study, it is virtually impossible to cover the entire area of reactivity in organized molecular systems. So this review will necessarily be selective and will be mainly focused on the personal work of the authors.
Compared with polar aprotic solvents, such as DMSO, DMF etc. microemulsions are more environmentally benign. Environmentally benign reactions have now become the target of synthetic organic chemists: the development of the concept of green chemistry and its twelve principles acts as a guideline for chemists. It is for this reason that the main objective of this review is to demonstrate that organized molecular systems are very efficient for the application of some of the twelve green chemistry principles, essentially: energy efficiency, safer solvents, atom and molecular economy, less hazardous chemical synthesis, use of renewable feedstocks, catalysis and biocatalysis, enzyme models, and reduced derivatives.
A secondary objective is to emphasize the role of some experimental tools useful for developing research in OMS: generalization of surfactant concepts, utilization of interfacial properties, proximity effects, molecular economy etc.
Organic chemistry has now developed so far that it is time to ask organic chemists about the future of their discipline, and reactivity in organized assemblies is one of the answers to this question [9]. A variety of synthetic reactions have been performed in organized molecular systems (OMS) formed by aggregations of individual molecules [10]. These organized media belong to the new discipline of supramolecular chemistry [11] which may be split into two levels of enquiry:
- • level 1: host-guest interactions;
- • level 2: study of molecular aggregates.
These both depend on low-energy molecular interactions sharing the following properties: molecular recognition, transport from one phase to another, and catalysis. Organized media, which are employed to facilitate chemical processes, mainly belong to the second level. These media are prepared by dissolving amphiphilic molecules in water or organic solvents. They then self-associate into colloidal entities adopting different forms (micelles, microemulsions, vesicles, etc.). The prime role of water has to be emphasized: defined structures arise spontaneously in water owing to non-covalent forces among the component molecules [12]. They excel as solvents with the further benefit of transparency, which confers particular value in photochemical applications [13]. The interest of such systems lies in their utilization as media for conducting reactions, where the selective dissolution of reactants, the stabilization of species and the bringing together of surfactants all favour catalytic processes. A higher degree of control of chemical reactivity is generally observed, and further progress in the physicochemical comprehension of such systems will undoubtedly help to match structure to reactivity.
2 Choice of system for conducting reactions
In general, organized systems are prepared from hydrophobic solutes, which highlights the important role of water. The solutes belong to four categories:
- • surfactants, versatile amphiphilic molecules;
- • planar cyclic or heterocyclic compounds which organize themselves in stacks;
- • non-planar condensed ring compounds such as sterols, which aid the formation of aggregates with a limited number of components;
- • hydrosoluble polymers, which tend to self-associate or organize themselves.
Apart from the systems formed by stacking, which are mainly employed to mimic interactions of purine and pyrimidine bases, media prepared from any of the other solutes can be employed in studies of chemical reactivity. We will discuss only the use of OMS formed with surfactants here.
Surfactants can promote the formation of well-organized monolayers at the various interfaces and aid the formation of aggregates in solution, producing a suspension of microscopic objects. This gives rise to heterogeneous phases within a liquid [14]. In water, spherical or deformed micelles tend to be obtained. According to the concentration and temperature, cylinders and then cubic or hexagonal liquid crystals and, finally, lamellae may be observed (Fig. 1). In non-polar solvents, a different type of aggregate is observed, consisting of reverse micelles which may contain small amounts of water. A number of solvents may be employed instead of water [15]. The degree of organization, however, depends on the cohesion properties (structure) of the liquid (Table 1).

Micellar solutions and liquid crystals.
Cohesive solvents.
Solvent | ɛ/ɛ° | μ (D) | γ (mN.m−1) at 20 °C | DCE (dyne.cm−2)a |
Water | 78 | 1.8 | 70 | 27.5 |
Glycerol | 43 | - | 63 | 17.8 |
Formamide | 109 | 3.4 | 58 | 15.0 |
Ethylene Glycol | 38 | 2.2 | 48 | 12.0 |
N-methyl formamide | 182 | 3.8 | 35 | 8.3 |
a Density of cohesive energy (DCE) = ΔUvap./Molecular volume ≈ γ/(V1/3).
These substitutes may confer benefits from the modification of properties such as polarity and solubility. We have also shown that a protic character is not necessary if the solvent is structured enough (high cohesive energy) and if it has high polarity. 3-methylsydnone is a good example of such liquids. With this mesoionic molecule, we have been able to form all types of aggregates [16] (Table 2).
Aprotic structured solvents.
Solvent | ɛ (T°C) | μ (D) | D.C.E. (dyne.cm−2) | γ (mN.m−1) |
3-methylsydnone | 144 (40 °C) | 7.3 | 14.0 | 57 (40 °C) |
Formamide | 109.5 (25 °C) | 3.4 | 15.0 | 58 (20 °C) |
Water | 80 (25 °C) | 1.8 | 27.5 | 70 (20 °C) |
Owing the low concentration of surfactant necessary to obtain micelles, the quantity of solute in the colloidal medium is very low. Synthesis for preparative purposes necessitates larger quantities, and a microemulsion is the medium of choice. Addition of hydrocarbon and alcohol to micellar solutions, generally consisting of water and surfactant, can give rise to a variety of microheterogeneous phases. Most of these media, albeit of different structures, are encompassed by the term microemulsion [17]. Microemulsions also include three-component systems: with or without cosurfactant (Table 3).
Characterization of emulsions and microemulsions.
Properties | Emulsions | Microemulsions |
Composition | Water, surfactant, oil | Water, surfactant, oil, cosurfactant |
Aspect | Turbid, milky | Clear |
Formation | With energy | Spontaneous |
Aggregate size | D ≈ 1 μm | D ≤ 500 Å |
Behaviour over time | Decantation | Stable |
In fact, the most useful system is that of four-component microemulsions: surfactant, cosurfactant (alcohol, short chain amine, etc.) water and hydrocarbon. The domain of existence of a microemulsion is defined by the spontaneous and abrupt transition from a cloudy emulsion to a transparent microemulsion. Numerous authors have described the phase diagrams of microemulsions (Fig. 2) as they are of particular interest for the tertiary recovery of oil [18]. Similar diagrams are obtained when water is remplaced by formamide.
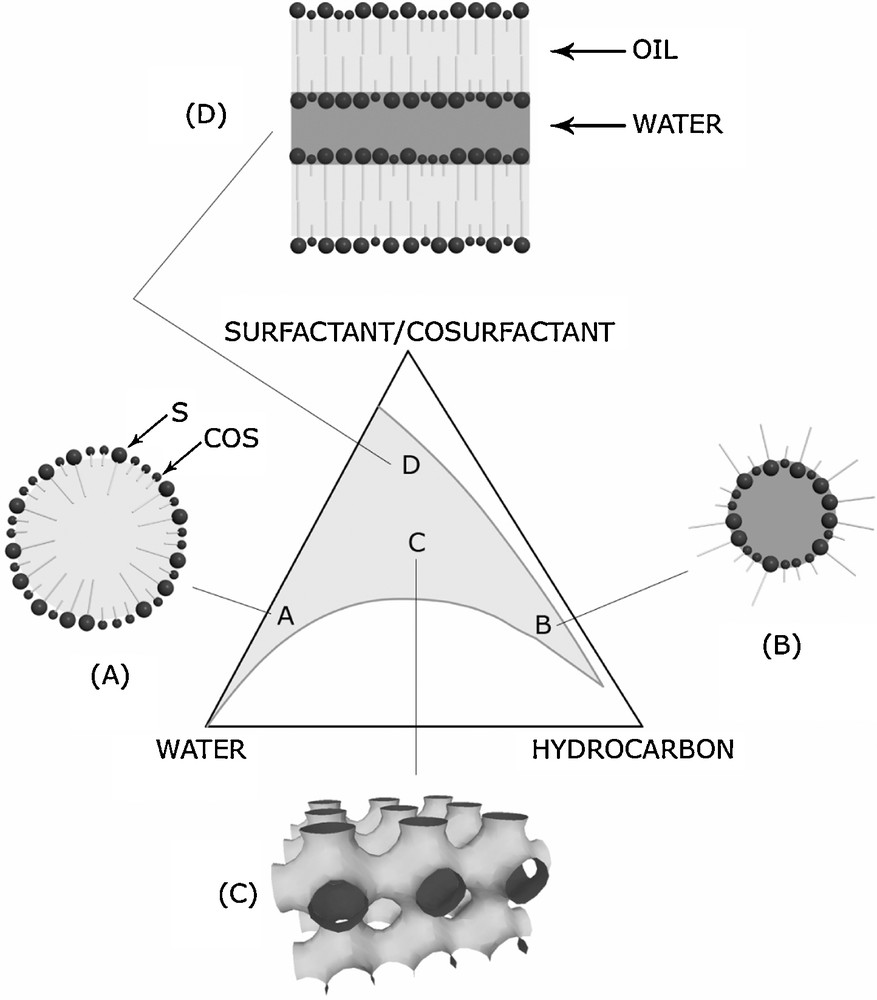
Structures of microemulsions.
The principal characteristics of microemulsions are as follows:
- • in the oil-rich zone: water in oil microemulsions (reverse micelles: W/O or L1) in which the dispersed phase consists of approximately reverse spherical micelles (Fig. 2B);
- • in the water-rich zone: oil in water microemulsions (direct micelles: O/W or L2) in which the dispersed phase consists of direct micelles having diameters ranging from 100 to 500 Å (Fig. 2A);
- • in the phase inversion zone: bicontinuous phase. In the zone of phase inversion, the microemulsions are said to have a bicontinuous structure with channels of hydrocarbon and channels of water (or formamide) separated by interfacial layers (Fig. 2C);
- • in the surfactant-rich zone: liquid crystal structures (Fig. 2D).
Microemulsions are media of choice for both mechanistic studies and preparative reactions since the droplets are larger than in a simple micellar solution, due to the high concentration of amphiphiles. They thus possess higher dissolving power. The problem of elimination of the surfactant can be overcome by optimizing the phase behaviour and by the transition to a polyphasic system for product separation. The high degree of dispersion makes microemulsions transparent, which confers a particular advantage for photochemical reactions: they are fluid and stable over time and a direct micellar system (O/W) can be transformed into a reverse micellar system (W/O). This may favour consumption of a large amount of one of the constituents (if it is also one of the reactants) while remaining in the macroscopically homogeneous domain.
3 Properties of organized liquid media
3.1 Compartmentalization [19]
Liquid organized media can both solubilize and localize reactants and products. Substances dissolved in these solutions will be distributed throughout the continuous phase as well as in the objects (Fig. 3).
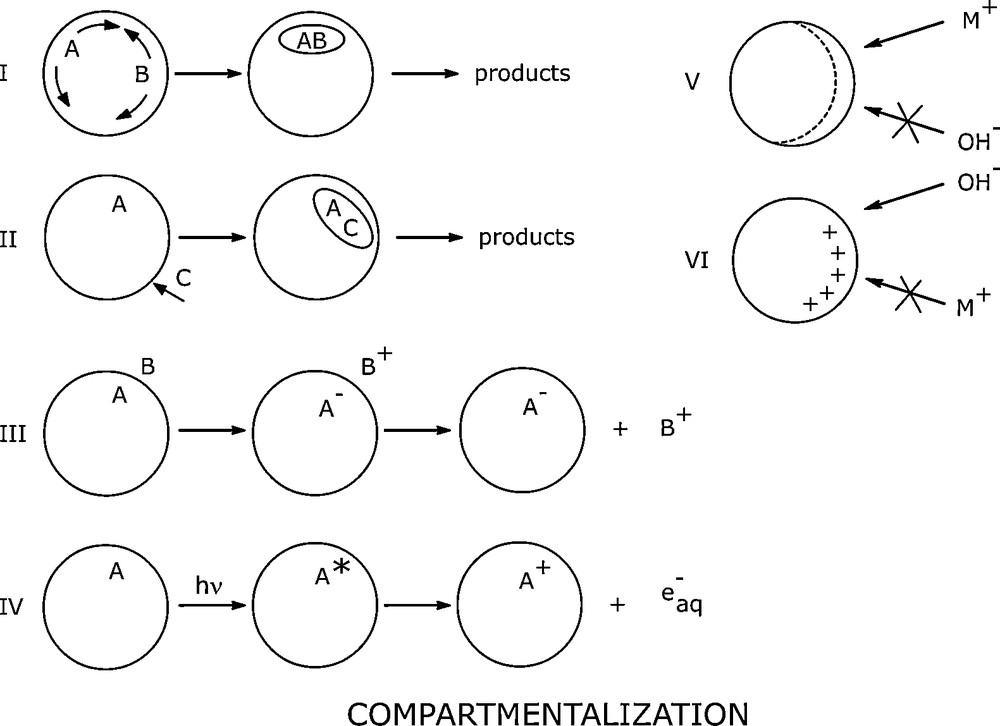
Compartmentalization.
This partitioning of reactants and products can be exploited to: locally modify concentration or maintain concentration gradients at the reaction site, prevent the approach of unwanted reactants, and favour charge separation. Another property is the ordering of molecules, which makes them react, or induces them to react, in a selective manner. The localization at interfaces and compartmentalization accompanied by a change of site after transformation will affect equilibria and can bring about changes in ionization and redox potentials (Table 4). Rapid and selective reactions of preparative amounts of substrate can be carried out in such media, which are also very suitable for mechanistic studies.
Molecular order and properties.
Molecular order |
Crystal > Monolayer > Micelle microemulsions > Polymer solutions > Molecular solutions |
Properties of organized liquid media |
Solubilization, localization, organization of the reactants |
Alteration of the concentration at the microscopic level and maintenance of reactant concentration gradients |
Modification of ionization potential, oxidation-reduction potentials |
Modification of reaction mechanism and velocity, by stabilization of reactants, intermediates or products |
Separation of the products and/or the charges |
3.2 Interfacial processes
In many cases, the reaction mechanism in OMS is essentially an interfacial reaction. To illustrate this behaviour, we will consider the probe reaction of 2-acetonaphthone oxime O-methyl ether studied by Padwa and Albrecht [20] (Fig. 4).
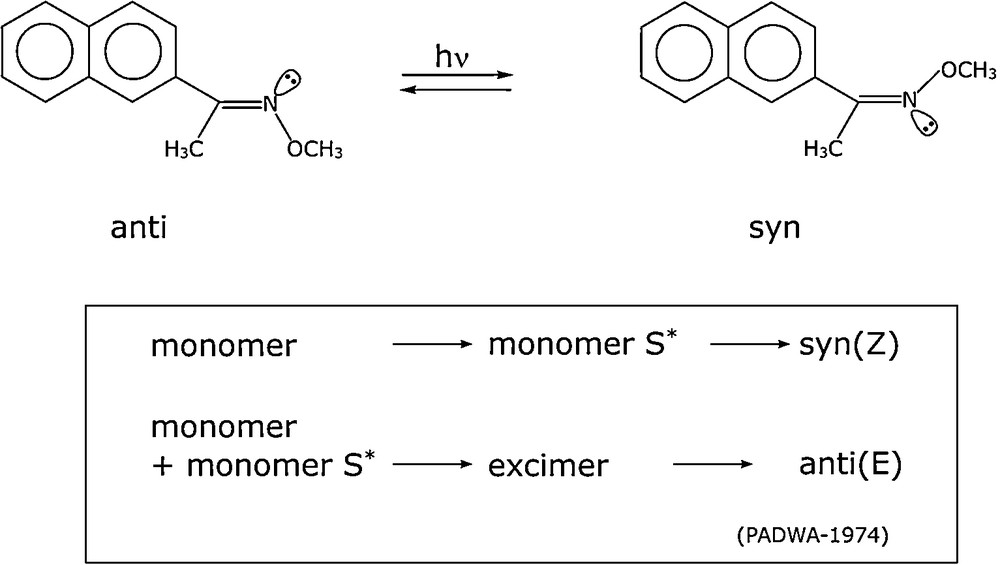
Photoisomerization of 2-acetonaphthone oxime O-methyl ether.
Irradiation led to a photo stationary state, the composition of which was dependent on:
- • temperature: high temperature diminished the fraction of the anti form;
- • solvent: higher polarity increased the fraction of the syn form;
- • concentration: high oxime ether concentration enhanced the fraction of the anti isomer in the photostationary state.
These investigations of Padwa on photoisomerization in benzene and pentane were extended by examining the effect of concentration in different solvents [21] including cyclohexane and more polar solvents such as acetonitrile and various alcohols. A linear change with concentration effect was more marked in protic than in aprotic solvents (Fig. 5).

Photoisomerization of 2-acetonaphthone oxime O-methyl ether in different solvents.
The percentage of the syn isomer was consistent with a lower polarity of the excimer compared to the excited monomer (singlet). The influence of protic solvents was not mediated by this process as methanol and acetonitrile have very similar polarities, although the excimer fluorescence emission was more readily observed in methanol than in the other solvents. The E → Z photoisomerization was then investigated in various microemulsion zones of the phase diagram using different surfactants and cosurfactants [22]. We discuss the results obtained with water/benzene/SDS/butanol (1/2) below, and describe the behaviour of the compound over the whole phase diagram (Fig. 6):
- • in the benzene-rich area (W/O with benzene as continuous phase), the change was linear with concentration, like that observed in non-polar solvents such as pure benzene. The oxime ether was localized in the bulk benzene phase and the presence of micelles did not affect the photoisomerization reaction;
- • in contrast, in the other areas, there was a discontinuous change with concentration. The plot of the proportion of Z isomer against concentration exhibited a discontinuity represented by two lines. The results were consistent with a localization of the oxime ether at the interface of benzene-rich micelles as this compound is insoluble in both water and mixtures of water and butanol.

Photoisomerization of 2-acetonaphthone oxime O-methyl ether in various microemulsion zones.
At low concentration, the compound lies at the interface, the reaction medium is protic and the effect of concentration resembles that observed in methanol.
With a rise in concentration, the excess oxime passes into the micelles and thus into benzene. The effect observed is the result of two processes:
- • photoisomerization in protic medium at the interface (at constant concentration);
- • photoisomerization in oil at high oxime ether concentration.
Thus the proportion of Z at the photostationary equilibrium was not greatly affected by the total concentration of oxime ether. For this reaction, processes at the interface were significant and, by studying the photoreactivity of cinnamic acid derivatives in microemulsion, it was possible to show how important the interface local concentration was.
4 Photodimerization of cinnamic acid derivatives
The photochemical behaviour of cinnamic acid and its derivatives is greatly affected by the medium structure. Thus the photodimerization of cinnamic acid in the crystalline state is one of the oldest and best known reactions but, in solution, the photoreaction gives no photodimers, only photoisomerization (Scheme 1).
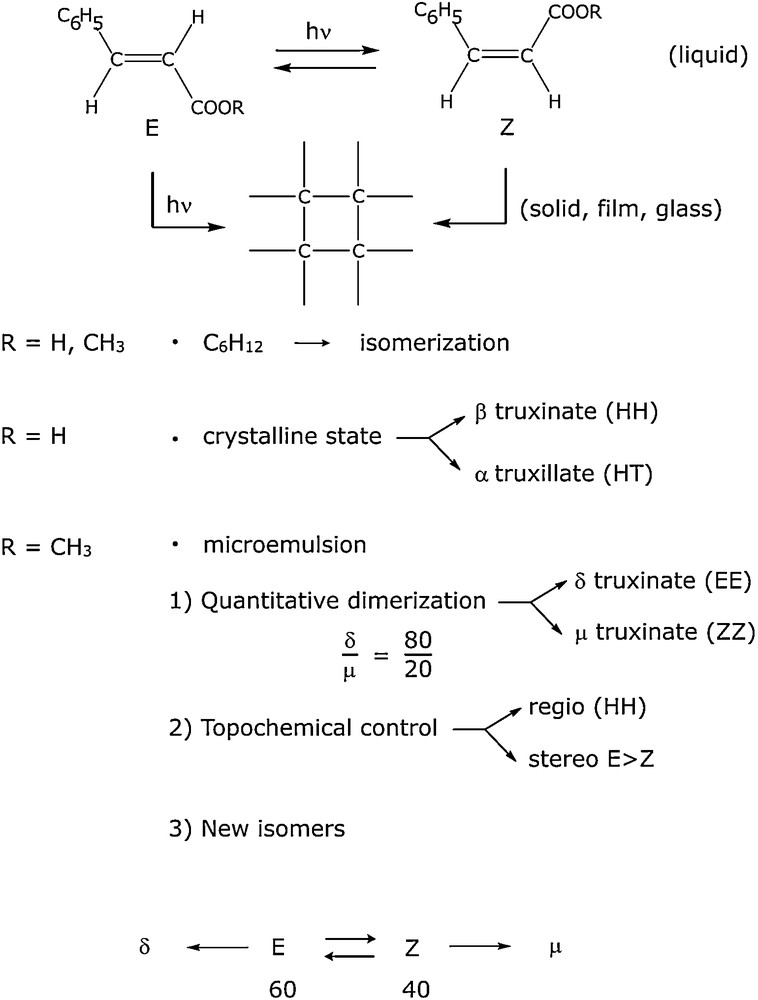
Photodimerization of cinnamic compounds.
We therefore investigated the photoreactions of cinnamic acid and methylcinnamate in microemulsions: water/cyclohexane/SDS/butan-1-ol (1/2). Because of their low solubility in water and cyclohexane, cinnamic derivatives are localized at the interface where the local concentration is high. By irradiation at 300 nm we observed the total formation of dimers with a regioselectivity depending on the photostationary isomerization rate [23]. Such results show that it is possible, thanks to the high local interfacial concentration, to obtain dimerization reactions in the liquid state which were previously only seen in the solid state.
5 Interfacial processes and selective extraction of products
A study of concentration effects in isophorone (3,3,5-trimethylcyclohex-2-en-1-one) photodimerization confirmed the role of the interfacial localization and also highlighted an interesting phenomenon of selective extraction of products from the bulk solvent to the interface.
5.1 Photodimerization of isophorone
This can give rise to three main products, as observed in conventional solvents (Fig. 7).

Photodimerization of isophorone.
In micellar media or water/cyclohexane/SDS (sodium n-dodecyl sulfate/butan-1-ol) microemulsions, the reaction can be oriented towards exclusive formation of the HH anti isomer (yields 95%) [24]. A study of the concentration effects of isophorone in W/O microemulsion (e.g. microemulsion 12), with cyclohexane as the bulk phase showed the interfacial localization of the ketone, not very soluble in water but whose reaction gives dimer with the same stereochemistry as in protic solvent and the continuous extraction to the interface. In microemulsion 12, in the reverse micelle part of the diagram (Fig. 7), it was possible to add 50% (vol/vol) of isophorone and still retain a transparent stable monophasic system. This led us to consider that isophorone could replace the hydrocarbon as a constituent of the microemulsion. This work also pointed out another feature: microemulsification is a general phenomenon and micro emulsions can be prepared with a polar component as “the oil”.
In this case, isophorone is both a constituent of the microemulsion and a reactant at the same time. This was the first example of our molecular economy principle [25].
All these observations can be explained by the following facts (Fig. 8):
- • at the interface, the HH dimerization rate is higher than the HT dimerization rate: (vHH)i >> (vHT)i;
- • in cyclohexane, the opposite phenomenon occurs: (v’HH)C6H12 << (v’HT)C6H12;
- • at the interface, the rate of HH isomer formation is much higher than the rate of HT formation in cyclohexane: (vHH)i >> (v’HT)C6H12;
- • the photodimer produced at the interface does not remain in the micelle; it is replaced by new isophorone molecules. Consequently, isophorone is continuously extracted from the cyclohexanic phase. This has been demonstrated by FTIR spectroscopy: the carbonyl group can be used as a probe by taking advantage of the hydrogen-bonding effects of the water or butanol on the carbonyl vibrational frequency. Frequency and intensity variations can be used to supply information on the localization of the ketone [26].
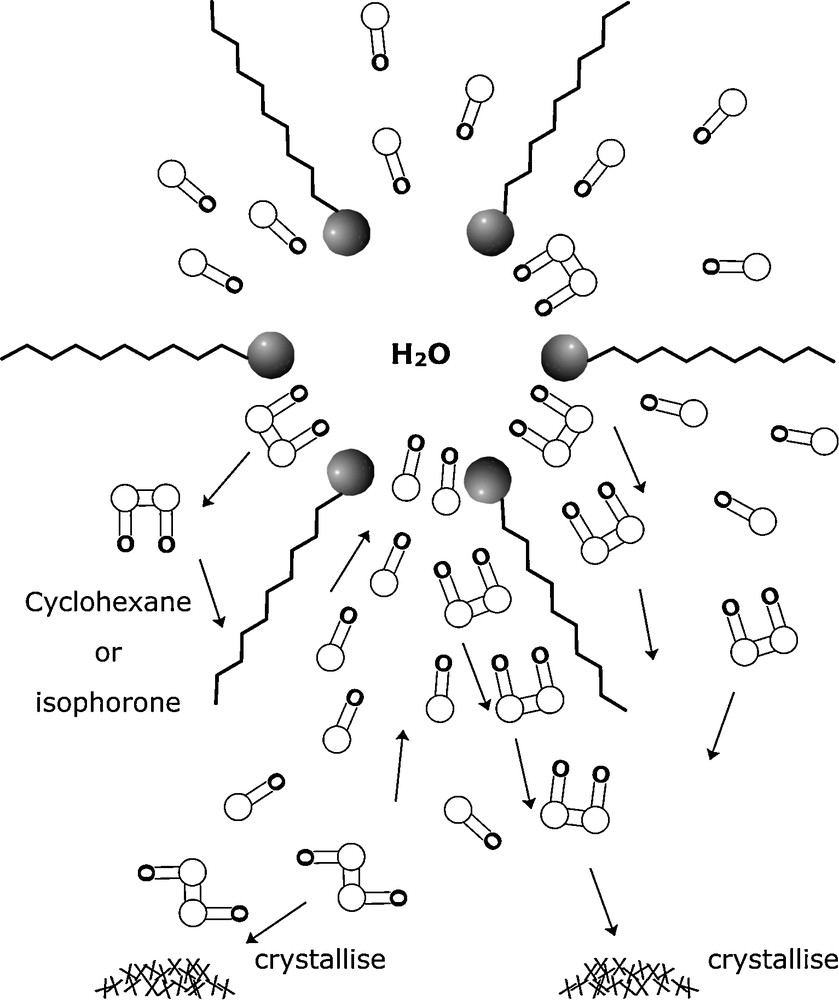
Selective extraction of products.
6 Aggregation formation without surfactant
We were interested in norbornene polymerization by a metathetic reaction with RuCl3 as catalyst. In conventional solvents (butanol), this catalyst is very selective and gives a 95% trans polymer. To study this reaction, we used mixture of formamide, norbornene, butanol and RuCl3 only (formamide and norbornene being non-miscible) [27]:
- • at low formamide concentration, we postulated that the medium was made up of small aggregates of formamide dispersed in a continuous phase, a mixture of butan-1-ol and norbornene. The reaction took place in the continuous phase and the stereoselectivity was the same as in pure butanol (5% cis) (Table 5);
- • when the formamide/butanol ratio was greater than 1.2, we observed an abrupt change of stereoselectivity (43% cis). Here we postulated the formation of droplets of norbornene in a continuous phase mixture of butanol and formamide.
Change of stereoselectivity in the metathetic polymerization of norbornene.
HCONH2/Butanol | Cis polymer (%) | Yield (%) |
0 | 5 | 85 |
0.2 | 5 | 82 |
1.2 | 5 | 74 |
1.5 | 43 | 63 |
3.4 | 42 | 40 |
The reaction took place at the interface of the droplets, the catalyst being in the continuous phase with the formamide in the coordination sphere of the metal. To verify our hypothesis, we carried out the polymerization in formamide microemulsion. The constituents of the microemulsions were: formamide/norbornene/SDS/butan-1-ol. The phase diagram of this system, with a constant formamide/SDS ratio of 2 is shown in Fig. 9. The results of the polymerization in microemulsions 1 (rich in formamide) and 2 (rich in norbornene) are also indicated in the same figure. These results confirm our interpretation and show that it is possible to obtain dispersed systems, analogous to OMS, without surfactants.
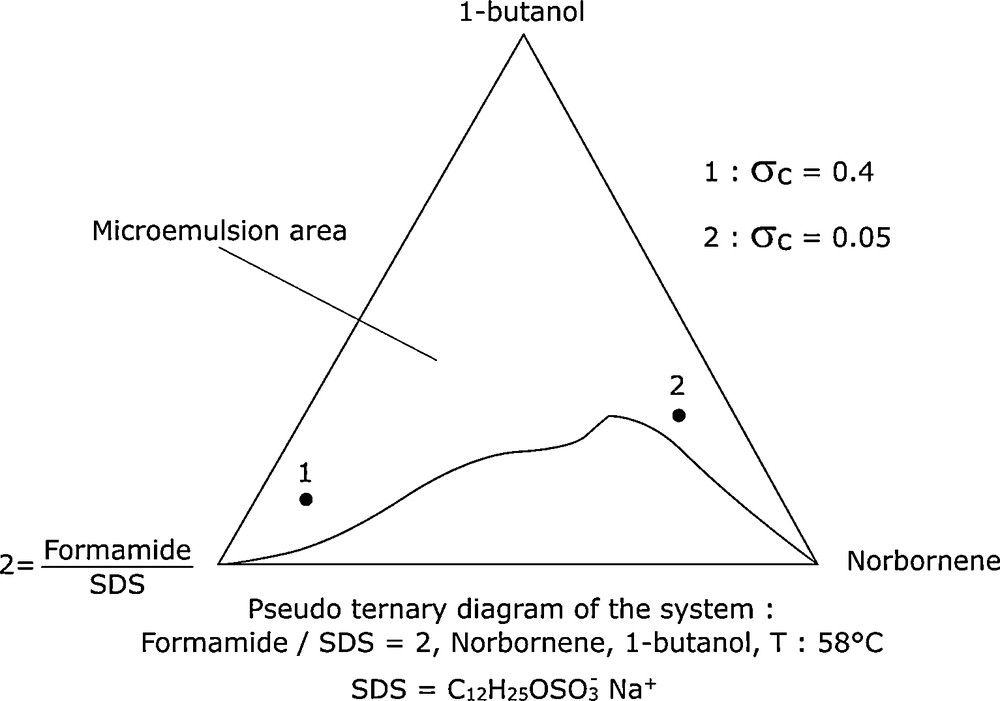
Norbornene metathetic polymerization in microemulsions.
7 Green chemistry and reactivity in organized molecular systems
Media are among the most important factors to be considered when attempting to perform organic synthesis in a soft and non-polluting environment satisfying the conditions of sustainable development. OMS can be used very efficiently for applying the twelve green chemistry principles (Paul Anastas and John Warner have put forward 12 principles that give chemists a road map for implementing green chemistry): replacing organic solvents, performing reactions in water, catalysis and biocatalysis, economizing molecules (and, of course, atoms) and working with low-energy conditions, are all possible for reactions in OMS.
7.1 Design for energy efficiency
Formamide microemulsions have been used as reaction media for the Wacker process, the catalytic oxidation of alkenes. This important industrial process has been in widespread use for the last 50 years [28]. It involves the transformation of a terminal olefin, RCH = CH2, into a ketone, RCOMe, by water, using a palladium (II) salt as catalyst. PdII is then regenerated either by CuII/O2 or a quinone.
R – CH = CH2 + PdII + H2O → RCOMe + Pd°C + 2H+
Formamide is a good solvent for salts with large ions [29]. We found the solubility of palladium chloride in formamide to be 50 gL−1 (only 0.7 gL−1 in water) and therefore decided to employ it in the Wacker process. We investigated the Wacker process in homogeneous media using a standard formamide microemulsion, hex-1-ene as olefin, with benzoquinone to regenerate PdII. The phase diagram is shown in Fig. 10.

Phase diagram of the Wacker catalytic microemulsions system: HCONH2, C9H19C6H4 (OCH2CH2)8OH (2:1 w/w); propan-2-ol; hex-1-ene - water (1,56:1 w/w).
Benzoquinone and hex-1-ene were then introduced into the microemulsions (M and N). In order to compare our results with other published studies, we investigated the oxidation of hex-1-ene in the heterogeneous medium, water-DMF [30]. The reaction carried out in DMF-water started after an induction period of around 100 minutes. In the microemulsions M and N, the main feature was the absence of an induction period. It should also be noted that the overall reaction was faster than in the water-DMF mixture, particularly in the olefin-rich microemulsion N which gave a three-fold increase in reaction velocity with respect to the traditional medium.
7.2 Safer solvents
Another mode of polymerization of norbornene is Ziegler-Natta type polymerization catalyzed by palladium chloride. With such a process, latex and microlatex are obtained (Scheme 2).

Polymerization of norbornene.
First, we demonstrated the feasibility of this reaction in water and in micellar solution of sodium n-dodecyl sulphate (Fig. 11). In contrast to metathesis, we obtained a saturated polymer in which the norbornane group was conserved (Scheme 2, reaction I) [31]. In aqueous emulsion we obtained stable latex with small particles of homogeneous size (d ≈ 10 nm) and atactic structure.
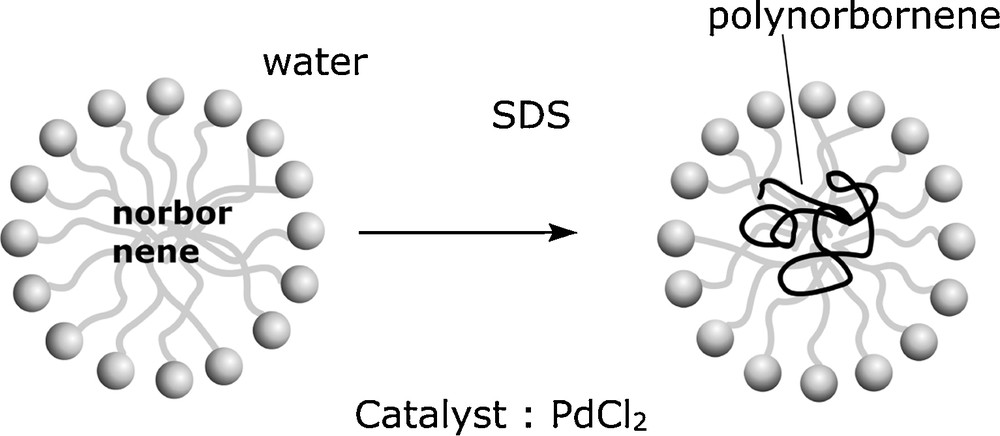
Polymerization of norbornene in aqueous emulsion.
The reaction was extended to the vinyl polymerization of functionalized norbornene: N-methyl norbornylgluconamide and N-methyl norbornyllactobionamide (Scheme 3) [32].
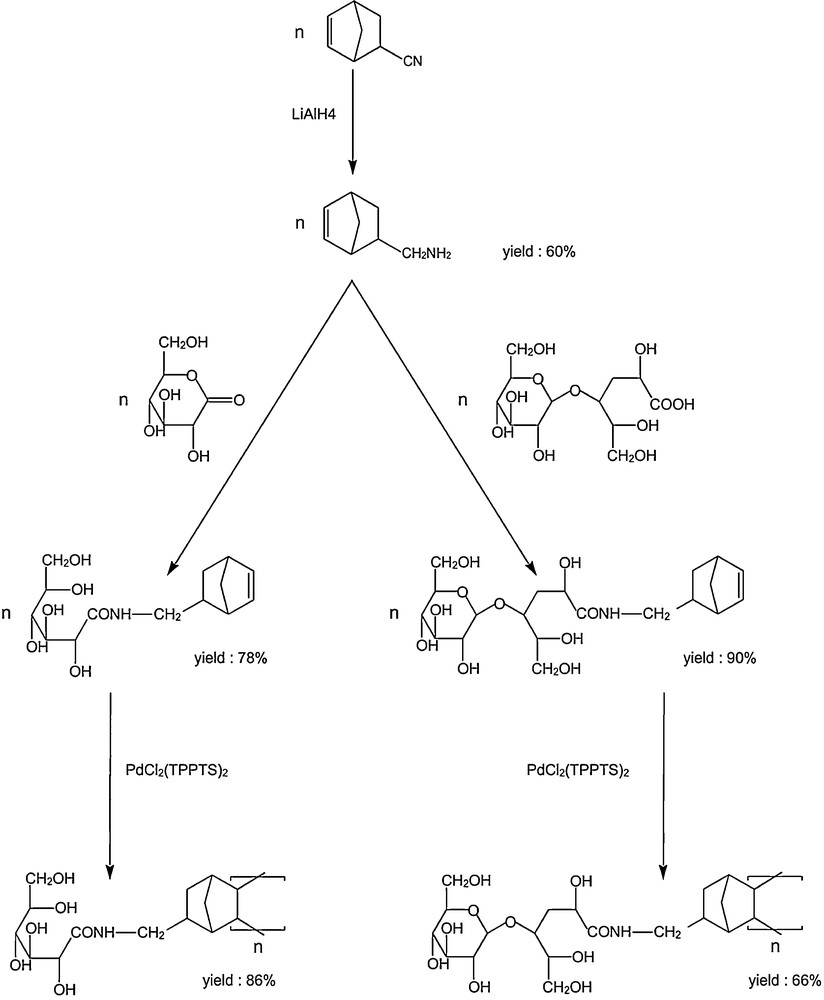
Polymerization of sugar-substituted norbornenes.
Vinyl polymerization of these derivatives produced a high yield of neoglycopolymers bearing the norbornene moiety. The water solubility of these sugar-based norbornene derivatives and their amphiphilic character enabled vinyl polymerization in a homogeneous aqueous phase, without additional surfactant. These amphiphilic polymers were highly soluble in water, forming stable particles consisting of aggregates of oligomers.
7.3 Atom economy or molecular economy
To respect the principle that synthetic methods should be designed to maximize the incorporation of all materials used in the process into the final products, we studied two synthetic reactions.
7.3.1 Effect of micelles on the preparation of compounds with large rings
Lactonization and lactamization giving lactones and lactames with medium and large rings is entropically unfavourable and dimerization or polymer processes cannot be adapted, even with high dilution techniques. Therefore the carboxylic groups of ω-hydroxy acids and ω-amino acids need to be activated in order to prepare the corresponding lactones and lactames. For example, Mukaiyama described the use of 2-chloro-1-methylpyridinium iodide (C16PyCl, I) as a carboxyl activating agent [33]. We tried to use the looping of the substrate at the interface of a reverse micelle (effect similar to a “2D template” effect) for lactonization and lactamization, by using modified Mukaiyama reactant: N-hexadecyl-2-chloropyridinium iodide (Fig. 12).

Macrocyclization using modified Mukaiyama reactant.
Note that we used N-hexadecyl-2-chloropyridinium iodide both as a constituent of the micelles and as a reagent. This is another application of the “molecular economy principle”. The organization of this agent in reverse micelles formed in dichloroethane facilitates the cyclization giving rise to a micellar effect. Lactamization of 12-aminododecanoic acid was carried out in that medium and the yield obtained with C16PyCl, I was much higher than those previously reported (Fig. 13) [34].

Lactamization of 12-aminododecanoic acid.
7.3.2 Reaction in the bicontinuous phase of a microemulsion: amidation of olefin
Amidation of olefin C8F17-CH = CH2 was performed by γ radiolysis in a formamide microemulsion containing the olefin as oil, a potassium salt of perfluorinated carboxylic acid as surfactant and an perfluorinated alcohol as cosurfactant [35]. The olefin and the formamide were thus both constituents of the microemulsions and reactants. We refer to this as the principle of “molecular economy” (Fig. 14).
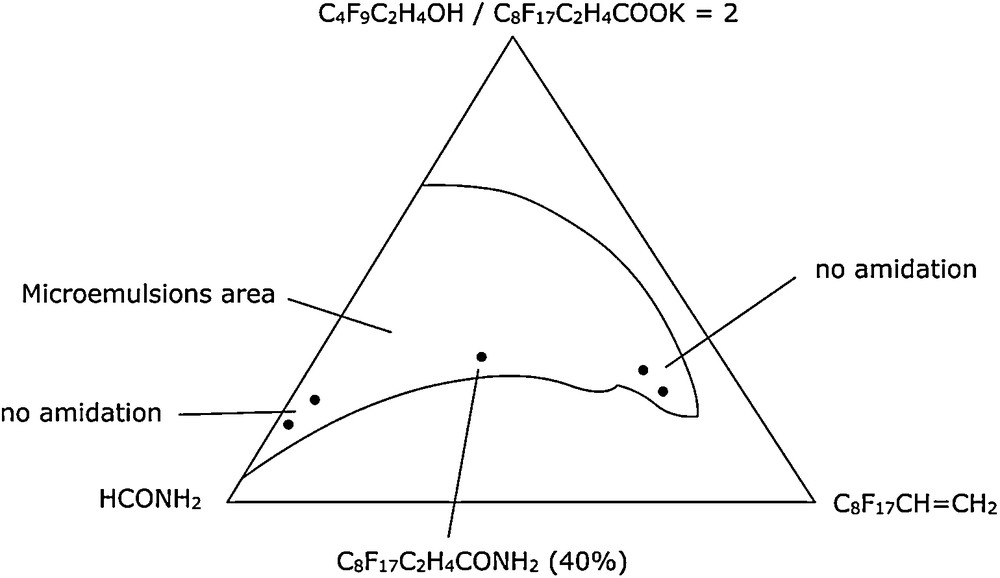
Amidation of mixed olefin in microemulsion.
The amidation reaction only took place in microemulsion with a bicontinuous structure. Here the constituents (also reactants) diffused together and suitable contact between formamide and the olefin could occur, thus enabling reaction. Photoamidation by formamide of substituted olefin RF-CH = CH-RH was carried out in the same conditions and in a homogeneous medium: tert-butyl alcohol. In tert-butyl alcohol, amidation occurred preferentially at the α-position on the alkyl chain whereas RH, in the microemulsions, the opposite regioselectivity was observed and the yields were enhanced (Scheme 4) [36].

Mechanism of olefin amidation.
7.4 Less hazardous chemical syntheses
7.4.1 Chemical decontamination of toxic compounds (warfare agents and pesticides)
This type of problem is increasing in importance. In this study we report the oxidation of mustard gas analogues in microemulsion media [37]. The success of chemical decontamination in a micellar solution was found to depend largely on the lipophilicity of the model compounds (evaluated by the log P parameter) and their corresponding sulphoxides. The reactivity and selectivity of the reaction were related to the localization of the different substrates in the microheterogeneous medium. Methoxyphenyl methyl sulphide, thioanisole and the half mustard 2-chloroethyl phenyl sulphide appeared to be suitable compounds to model the degradation of yperite (Scheme 5). So, we used these compounds as simulants in the oxidation reaction in microemulsions, where they acted as the oil of the system (“molecular-economy principle”) [38].

Chemical decontamination of mustard gas.
In order to obtain a pseudoternary phase diagram, we chose a cosurfactant: butanone and a surfactant: cetylpyridinium chloride (CPCl), with a cosurfactant/surfactant weight ratio of 2. The third constituent was the binary solvent system (water-formamide, 50/50, V/V). The resulting pseudoternary phase diagram is presented in Fig. 15.

Microemulsion for decontamination of yperite.
We chose the formulation visualized by point A on the phase diagram. The three model substrates were oxidized in the formulation described above. Magnesium monoperoxy phtalate (MMPP) was used as the oxidizing agent. Our results are summarized in Table 6. The analysis of these results shows that the three model substrates were oxidized quantitatively. The selectivity in favour of sulphoxide was excellent: no more than 6% sulphone was produced, and only 1% sulphone was formed in the oxidation of thioanisole by the binary solvent system microemulsion.
Oxidation of the model substrates in microemulsion medium by MMPPa.
Microemulsion | ||||
Model substrate | Aqueous | Binary systemb | ||
Yield (%) c | Select. c | Yield (%) c | Select.c | |
p-CH3O-C6H4-SCH3 | 100 | 95:5 | 99 | 94:6 |
C6H5-SCH3 | 100 | 96:4 | 100 | 99:1 |
C6H4-SCH2CH2Cl | 98 | 96:4 | 98 | 95:5 |
a All reactions were carried out at 25 °C for 1 h. The molar ratio MMPP: substrate was kept constant at 0.55.
b Water–formamide (50:50 v:v).
c Yields and selectivity (molar ratio sulphoxide:sulphone) were determined by HPLC after calibration using an internal standard. The uncertainty of the results is evaluated at ±2%.
7.4.2 Formulation for decontamination of polymer-thickened agent
Mustard gas and soman (pinacolyl methylphosphonofluoridate) are often formulated with 5 to 10% (by weight) of an organic polymer to enhance their adhesion to surfaces and to prevent solubilization of the toxic in most aqueous decontaminant solutions. The presence of a thickener adds constraints to the design of an efficient decontamination medium. In order to be efficient, the microemulsion must contain one organic solvent able to solubilize both toxic and polymer; we chose benzyl chloride, which dissolves the polymer efficiently [39]. The formulation finally used was: cetylpyridinium chloride as the surfactant, butan-1-ol as the cosurfactant, water as the continuous phase, benzyl chloride as the oil. The pseudoternary phase diagram is presented in Fig. 16.
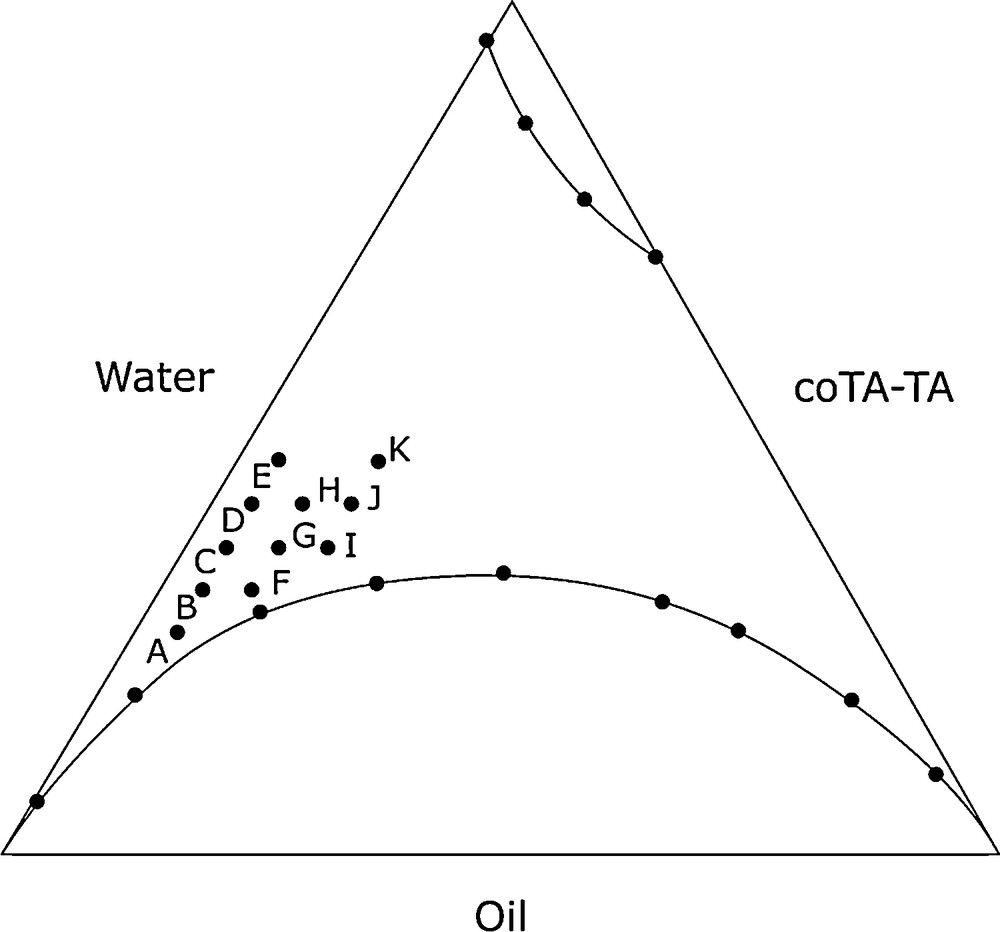
Formulation for decontamination of polymer-thickened agent.
We therefore performed the oxidation tests with MMPP at points E, H, K. The results obtained are listed in Table 7. The formulation was efficient despite of the difficult experimental conditions we imposed: oxidation of 2-chloroethylphenylsulphide occurred with 85% conversion and suitable chemoselectivity.
Decontamination of polymer-thickened agent.
Formulation | Half-mustard | Polymer-thickened | Half-mustard |
E (5% Oil) | Yield %a Select.a | 96 95:5 | 81 80:20 |
H (10% Oil) | Yield %a Select.a | 95 90:10 | 86 84:16 |
K (15% Oil) | Yield %a Select.a | 97 97:3 | 84 87:13 |
a By HPLC analysis.
All these results demonstrate the efficiency and potential of microemulsions for mustard gas destruction/decontamination in essentially aqueous systems.
7.5 Use of renewable feedstocks and design of safer chemicals
A common challenge in surfactant systems is to prepare amphiphilic compounds that perform their desired function with minimum toxicity. Thus non-ionic surfactants can be synthesized by using sugar chemicals as raw materials (which are also renewable feedstocks). Alkypolyglucosides (APG) are, in our opinion, the must important group of “green surfactants”. The Henkel process is one of the most efficient methods for their synthesis (Scheme 6). This process has some drawbacks: low pressure distillation is necessary to extract water and BuOH, yields are low (35%) (the final mixture contains dodecyl alcohol and butyglucoside) and, at the end, the product is yellow. We propose a one-step auto-microemulsification process for the synthesis of alkylglucosides (Scheme 7).

Henkel process of alkyl glucoside synthesis.

One-step auto-microemulsification process for the synthesis of alkyl glucosides.
Here, the butylglucoside is synthesized “in situ”; the reaction does not involve distillation as the equilibrium is shifted to the formation of the final product by water becoming entrapped in the reverse micelles. The system works at room temperature and the final product is white [40].
7.6 Catalysis and biocatalysis
Organized molecular systems are very suitable media for enzymatic reactions, enzyme being soluble in the water pool of reverse micelles and hydrophobic substrates being easily solubilized in the organic bulk solvent. We have tested the oxidation of linoleic acid by soya lipoxygenase in reverse micellar solution [41]. We chose bolaamphiphilic sugar compounds as surfactants. With these surfactants, the enzymatic reactions take place very easily and, thanks to the nature of the bolaforms, the specific activity of the lipoxygenase is not altered after 60 h of running (Table 8).
Activity of soya lipoxygenase after 60 h in presence of bolaforms or Tween 20.
Surfactant | Tween 20 | n = 6 | n = 7 | n = 8 | n = 9 | n = 12 |
Specific activity | 573 | 11863 | 13294 | 11863 | 12067 | 12067 |
Relative activity (%) | 4 | 79 | 88 | 79 | 81 | 80 |
Surfactant | Tween 20 | n = 6 | n = 7 | n = 8 | n = 9 | n = 12 |
Specific activity | 573 | 10523 | 15032 | 13529 | 13530 | 15033 |
Relative activity (%) | 4 | 70 | 100 | 90 | 90 | 100 |
7.7 Enzyme model
With OMS, it was also possible to make aggregates having a functional configuration similar to the active site of an enzyme [42]. Thus, a-chymotrypsine possesses three important functional groups in its hydrophobic pocket active site:
- • imidazole ring;
- • carboxylic and hydroxylic groups.
For enzyme mimicking, it is necessary to have an arrangement where at least part of these reactants are associated. So, we used CTAB as surfactant: the core of the micelles was then the hydrophobic pocket and the cationic charge was favorable for a nucleophilic attack of a substrate by a nucleophilic reactant (OH– or H2O). To obtain an imidazole ring at the interface of the micelle, it is possible to use a compound having a hydrophobic tail to entrap the product in the aggregate. We chose the hydrolysis of p-nitrophenylacetate (PNPA) as the test reaction, and to facilitate the transfer of the acetyl group after the hydrolysis (and the regeneration of the system) we dissolved chloral hydrate in the aqueous solution. The scheme summarizes the postulated mechanism of the process (Scheme 8).

Postulated mechanism of the hydrolysis of p. nitrophenylacetate.
Different long chain substituted imidazole compounds were tested and, with the dodecyl ester of urocanic acid, we observed a very good result: the hydrolysis was nearly 5000 times faster than with CTAB alone. Compared with a pure water solution, the kinetics was approximately 40,000 times better. Varying the imidazole/PNPA ratio led to no modification of the activity, showing that the system worked as a catalytic one [43].
7.8 Reduction of derivatives
In many cases we synthesized functionalized molecules avoiding the use of derivatization. These syntheses were often in the field of sugar chemistry. We obtained new amphiphilic dendrimers derived from PAMAM (a commercial dendrimer) [44] and a chiral substrate, D-gluconolactone [45] (Fig. 17). The introduction of chirality into a dendritic structure creates an asymmetric macromolecule having a chiral surface with internal cavities. These dendrimers can act as chiral rigid unimolecular micelles. This provided an opportunity to investigate these chiral dendrimers as potentially useful materials in mediating chiral recognition and enantioselective binding of guest molecules. Furthermore, it is well known that the mobility of micelles avoids the use of chiral surfactants in asymmetric synthesis [45]. The dendritic chiral rigid unimolecular micelles could be used as promoters or catalysts in chiral induction, e.g. in the reduction of ketones.

Glycodendrimers derived from PAMAM and D-gluconolactone.
7.9 Asymmetric reduction of acetophenone by NaBH4 at the interface of amphiphilic chiral supports in water [46]
To investigate the influence of the topology of the chiral surface, the reaction was carried out in the presence of various chiral supports with the same sugar group (gluconamido) and structural modulations from the different generations of dendrimer [G(1)G, G(2)G, G(3)G, G(4)G]. The results for the reduction in the presence of these different chiral supports are summarized in Table 9.
Enantioselective reduction of acetophenone in water in presence of different chiral supports.
Chiral support | Yield (%) | % eea |
80 | 0 | |
30 | 0 | |
G(1)G | 95 | 0 |
G(2)G | 98 | 0 |
G(3)G | 95 | 50(S) |
G(4)G | 92 | 98(S) |
a Determined by GC using a βDex 110 column.
In these homogeneous conditions, only G(4)G gave high enantioselectivity; all the other chiral supports led to poor results.
The enantioselectivities obtained appeared to be directly influenced by the external surface of the structure [47]. Even with substrates well known for giving poor results (especially linear ketones), good enantioselectivities were obtained. It is also important to note that, as the dendrimer was insoluble in methanol, it was very easy to recover it by filtration (Fig. 18).
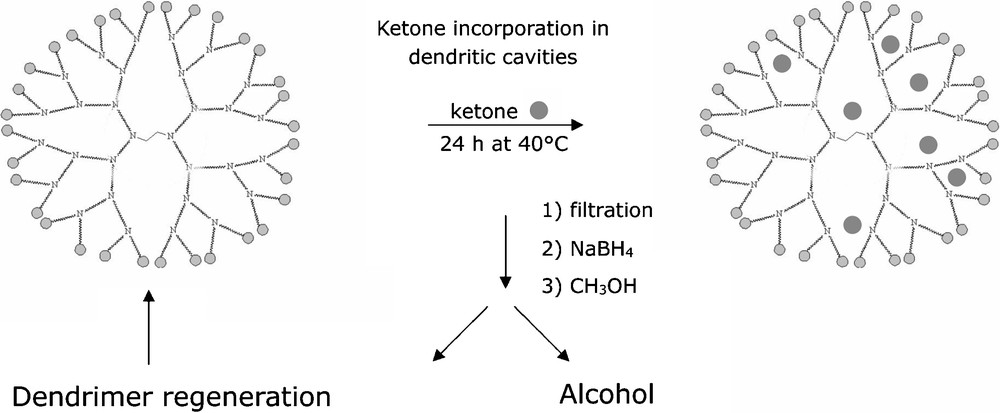
Dendrimer regeneration.
8 Conclusion
It can be seen from the examples described here that OMS show considerable promise for synthetic applications.
Based on the results already obtained, we believe that organic synthesis can be further advanced by improved formulation of the reaction media. Rather than employing conventional homogeneous media, chemists may now be able to design media to favour and optimize a desired reaction. This approach is a valid alternative to phase transfer catalysis, which in fact often proceeds via the same mechanism. From an industrial and practical point of view, microemulsions are easier to stir than biphasic media as they are macroscopically homogeneous.
The development of this strategy hinges largely on the training of chemists, as it requires a through knowledge of synthetic chemistry and the physicochemical properties of colloidal systems. This poses the problem of specialization within a simple discipline.