1 Introduction
Supported sulfided transition metals have a great importance because they are used as hydrotreatment catalysts. Generally, the industrial catalysts derive from a mixture of sulfides of one of the elements of group VIB (Mo or W) and one of group VIII (Co or Ni), and they often contain phosphorus to improve their catalytic activity. Beneficial effects of phosphorus on the performances of hydrotreatment catalysts have been reviewed by Iwamoto and Grimblot [1]. Alumina is the conventional support due to its excellent mechanical properties and its ability to disperse the active sulfided phase. Due to the necessity to develop hydrotreating catalysts with enhanced properties, other supports have also been studied such as silica, titania, or zirconia [2]. Apatites, with structural formula Ca10(PO4)6(OH,F)2, which form the mineral compound of bones and teeth, are handled as a solid, harmless to the environment and are stable materials. Some recent papers report their use as new support materials in various catalytic reactions as oxidation [3], water gas shift conversion [4], Knoevenagel condensation [5].
Only few works are devoted to the study of such phosphorus materials as a carrier for sulfided catalysts. In recent papers, it has been reported that NiMo sulfide catalysts supported on hydroxyapatite and various AlPO4-enriched apatite were found to be more active catalysts for the selective conversion of dimethyldisulfide (DMDS) into methanethiol than an industrial NiMoP/Al2O3 catalyst [6,7]. Moreover, within a series of NiMo/apatite and industrial NiMo/alumina sulfided catalysts, we have shown [8] that the reaction rates for DMDS hydrogenolysis linearly correlated with those determined for hydrodulfurization (HDS) of dibenzothiophene, hydrodenitrogenation of quinoline, and hydrogenation of methylnaphtalene. It was, thus, deduced that the nature of the catalytic Ni–Mo–S sites is the same in apatite- and alumina-based catalysts, in particular for hydrogenolysis of C–S, C–N and S–S bonds and for hydrogenation of aromatic rings. Since hydrodulfurization of dibenzothiophène carried out under high hydrogen pressure was reported to correlate real feed HDS [9], dimethyldisulfide appears to be a good model molecule to evaluate the catalytic properties of HDS catalysts.
We have shown that the activity was improved when a calcium-deficient apatite (Ca/P atomic ratio < 1.67) was used because superficial HPO42− species or surface defects facilitated the dispersion of the NiMo oxidic precursors and then that of the sulfided catalytic phase. The apatitic supports have been previously synthesized using research-grade phosphate salts. The present work intends to investigate phosphate-based materials prepared from industrial phosphoric acid of various purities (29, 54, and 60 wt.% P2O5) that was provided by the Moroccan company OCP (Office Chérifien des Phosphates). The apatitic solids were used as a support of the NiMo sulfided phase. Catalyst performances have been evaluated for the hydrogenolysis reaction of dimethyldisulfide and compared to that of an NiMoP/Al2O3 commercial catalyst (Axens, HR348).
2 Experimental
2.1 Characterization techniques
The specific surface area of the catalysts was measured by N2 physisorption using the BET method on a Quantasorb-junior apparatus. A sample mass of 100 mg was degassed at 423 K for 45 min before measurements.
Powder X-ray diffraction (XRD) patterns were recorded on a Huber diffractometer (Model G670) using the Cu Kα radiation. The patterns were collected over the 2θ range from 10° to 80°.
Transmission IR spectra were recorded from 200 cm−1 to 4000 cm−1 at 293 K on a Nicolet 460 spectrophotometer. Samples were prepared by mixing 1 mg of powdered solid with 150 mg of dried KBr.
TGA analyses were performed using a Thermal Analysis instrument (Model SDT 2960) on 20 mg of sample. Mass losses were recorded under synthetic air, with a heating rate of 4 K min−1 from 293 K to 1073 K.
Catalytic measurements were performed in a fixed-bed quartz reactor operated at atmospheric pressure under a flow (0.08 mol h−1) of dimethyldisulfide (2.9 kPa) in hydrogen [6]. The DMDS conversion was studied at 453 K. DMDS and the product of reaction CH3SH were analysed with an on-stream gas chromatograph (Varian 3700) equipped with an FID detector. The catalyst activity was expressed as the first-order rate constant (L h−1 g−1) determined at conversion lower than 12% [6].
2.2 Support preparation
The supports were prepared by the conventional aqueous precipitation method [10]. A volume of 250 cm3 of calcium nitrate solution Ca(NO3)2·4H2O (0.3 mol L−1) was added dropwise to 1000 cm3 of a phosphate boiling solution (0.083 mol L−1) with a Ca/P atomic ratio equal to 0.9, in order to form calcium-deficient apatite. The phosphate solution was a solution of diammonium hydrogenophosphate (NH4)2HPO4. Three other supports were prepared using industrial phosphoric acid with different purities (29%, 54% or 60 wt.% P2O5). In all cases, the pH of the solution was maintained between 9 and 10 during the overall precipitation process by addition of a solution of ammonium hydroxide. The temperature was fixed at 369 K. The suspension was continuously stirred. After total addition of the calcium nitrate solution, the suspension was matured during 30 min. After filtration, the white precipitate was washed with distilled water, dried at 353 K during 12 h and then calcined at 673 K for 4 h. The following calcined apatite was either denoted AH when it was prepared from an (NH4)2HPO4 solution or AHP-p (p being the weight percentage of P2O5 in the industrial acid) when prepared from industrial phosphoric acid. Chemical analysis was performed by the “Service central d'analyses du CNRS” (Vernaison, France).
2.3 Catalyst preparation
Bimetallic catalysts NiMo/AH and NiMo/AHP-p were prepared by successive incipient wetness impregnation, with an ammonium heptamolybdate solution, then with a nickel nitrate solution, after intermediate calcination at 673 K. The Mo content was fixed at 3.5 Mo nm−2, and the Ni/(Ni + Mo) atomic ratio was adjusted around 0.44 because the catalytic activity was found to be maximum with this composition [6]; that lead to bimetallic catalysts with a metal oxide content close to 6.3 wt.% MoO3 and 2.5 wt.% NiO.
The oxidic NiMo samples were sulfided under a flow of H2–H2S–CH4 (60 vol.%–15%–15%) at T = 633 K.
3 Results and discussion
Chemical composition results are reported in Table 1. These results reveal the presence of fluorine in every support prepared from industrial phosphoric acid. Indeed, among the numerous impurities in the industrial phosphoric acid, such as calcium, magnesium, iron, sulphur and aluminium, fluorine was found to be one of the most important. Its presence came from the manufacturing process of phosphoric acid; H3PO4 is obtained by the action of sulphuric acid on a phosphate mineral ore, which contains fluorine in a fluoroapatite solid. The wet treatment by sulphuric acid transforms this solid into H3PO4 together with phosphogypsum CaSO4·2H2O and HF acid. In the prepared supports AHP-p, the amount of fluorine varies from 1.03 to 2.94 wt.% (Table 1). In these AHP-p materials, the Ca/P atomic ratios are found to be lower than the stoichiometric ratio 1.67, which is in line with the presence of a Ca-deficient apatite structure as observed with sample AH [5–7]. The specific surface area of the supports has been measured and the values reported in Table 1 range from 78 m2 g−1 to 95 m2 g−1, showing that these apatitic materials would be suitable supports with a sufficient surface area. The increase in specific surface area for samples AHP-54 and AHP-29 could be explained by the presence of aluminium ions (provided by the industrial phosphoric acid) in these materials. Indeed, we have shown [6,8] that Al-enriched apatite materials exhibited a higher specific surface area than the non-enriched one.
Chemical composition results and specific surface area of apatite supports.
Support | Ca (wt.%) | P (wt.%) | F (wt.%) | Ca/P (at./at.) | Specific surface area (m2 g−1) |
AH | 36.47 | 18.09 | – | 1.56 | 81 |
AHP-60 | 38.39 | 18.75 | 1.32 | 1.58 | 78 |
AHP-54 | 32.88 | 17.66 | 1.03 | 1.44 | 95 |
AHP-29 | 33.41 | 16.66 | 2.94 | 1.55 | 92 |
XRD patterns of the supports are presented in Fig. 1. Diffraction patterns confirm the presence of an apatite phase Ca10(PO4)6(OH,F)2 in every support. The increase in phosphoric acid purity leads to an increase in apatite crystallinity, as noticed by the narrowing of the diffraction lines observed for AHP-60 and AH synthesized with phosphoric acid 60% and diammonium hydrogenophosphate, respectively. In the XRD patterns of AHP-54 and AHP-29, the broad line at 2θ = 32° could indicate the formation of fluoroapatite Ca10(PO4)6F2 [10,11], whereas AH and AHP-60 XRD patterns, which are found to be very similar, are characterized by two separate diffraction lines at 2θ = 31.78° and 2θ = 32.12° in agreement with the formation of hydroxyapatite Ca10(PO4)6(OH)2 [11]. Thus, it seems that the low amount of fluorine does not prevent the formation of hydroxyapatite in the AHP-60 solid, contrary to the case of the AHP-54 and AHP-29 solids.

XRD patterns of AHP-29, AHP-54, AHP-60 and AH supports.
IR spectra, displayed in Fig. 2a, confirm the formation of an apatite phase whatever the support, with the observation of fundamental vibrational mode of PO4 groups at 574 cm−1, 609 cm−1, 966 cm−1 and 1020–1120 cm−1 [12,13]. The presence of adsorbed water could also be detected from IR spectra in the range 3300–3600 cm−1 (Fig. 2b) and about 1637 cm−1. In both samples AH and AHP-60, the bands at 633 cm−1 and 3572 cm−1, shown in Fig. 2a and b, are attributed to structural OH groups in a hydroxyapatite phase Ca10(PO4)6(OH)2 in agreement with the results reported in the literature [14,15]. Such OH groups are not detected in sample AHP-29 indicating that this sample is not a hydroxyapatite but rather a fluoroapatite as already deduced from XRD analysis. The IR spectrum of AHP-54 shows a very weak band at 3572 cm−1 showing the formation of a low amount of hydroxyapatite in this support mixed with fluoroapatite. Furthermore, a careful examination of Fig. 2a allows one to notice a band at 875 cm−1 for AH and AHP-60 that is assigned to HPO42− groups of an Ca-deficient apatite [16,17], with a general formula Ca10−x(HPO4)x(PO4)6−x(OH)2−x, where x represents cationic vacancies. From chemical compositions, the number of vacancies is determined to be 0.5 vacancy per 10 calcium ions in both AH and AHP-60 supports; subsequent surface defects are thus very likely on those Ca-deficient apatites. In the spectra of AHP-29 and AHP-54 solids, the band at 875 cm−1 was not visible, indicating a very low amount or the absence of HPO42− groups in these solids. Since a Ca/P atomic ratio below 1.67 (Table 1) points to the presence of a Ca-deficient apatite phase, it seems that the formation of a non-stoichiometric fluoroapatite cannot be excluded in both AHP-29 and AHP-54 supports.
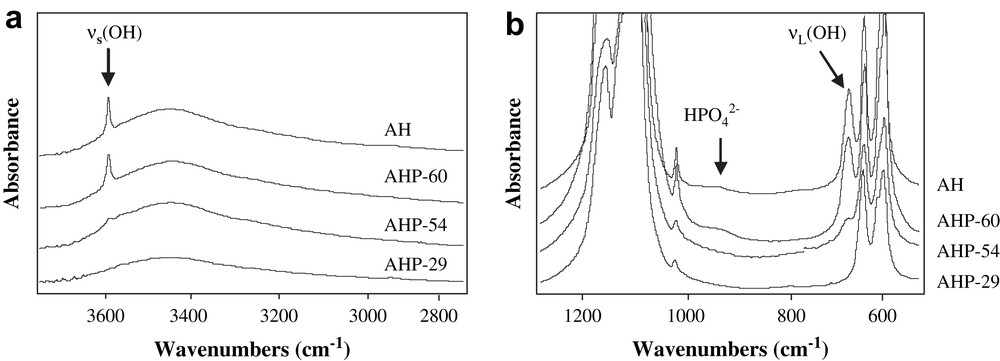
IR spectra of AHP-29, AHP-54, AHP-60 and AH apatite supports; (a) 760–1220 cm−1, (b) 2800–3800 cm−1.
The thermal decomposition of the dried supports is reported in Fig. 3. In addition, the derivative curve corresponding to the thermal decomposition of the sample AH is also shown in Fig. 3, in order to better evidence the temperatures at which weight losses occurred. The results show that the curves corresponding to samples AH and AHP-60 have almost the same profile, with marked weight losses centered around 350 K and 520 K (as shown by the derivative curve) due to physisorbed and constitutive H2O departure, respectively. A lower continuous weight loss was observed for all samples until 770 K where a plateau was reached. An important weight loss was again observed at 1000 K, particularly for samples AH and AHP-60. This phenomenon is less noticed for the sample AHP-54. It is due to the condensation of HPO42− ions [18] through the reaction:
2HPO42− → P2O74− + H2O |

TGA curves of dried supports and derivative of mass loss for the dried AH support.
This reaction is obviously characteristic of a Ca-deficient hydroxyapatite Ca10−x(HPO4)x(PO4)6−x(OH)2−x as already reported in the literature [19]. TGA analysis agrees with the formation of Ca-deficient hydroxyapatite in AH and AHP-60 supports, and to a lesser extent in AHP-54. The condensation of hydrogenophosphate species was not observed in the sample AHP-29, in line with a fluoroapatite structure as deduced from XRD and IR data.
4 Catalytic results
Table 2 shows the activity in DMDS hydrogenolysis of NiMo apatite-supported and industrial NiMoP/Al2O3 catalysts. Among the catalysts, the sulfided NiMo/AH catalyst was found to be the most active. The activity of apatite-supported catalysts is in relation with the purity of the phosphoric acid solution: the activity increases as the purity of the acid used in the support preparation is raised. Indeed, the activity of AHP-60 supported catalyst is almost similar to the activity of the AH-supported one and higher than the activity obtained for the NiMoP/Al2O3 catalyst. Moreover, those two NiMo/apatite catalysts are more active than the industrial NiMoP/Al2O3 catalyst although they have about half of its NiMo content. Such a finding has already been observed in the conversion of dibenzothiophene performed on NiMo sulfide supported on Ca-deficient apatite supports [8]. It thus can be stated that promotion of MoS2 slabs by Ni ions is easier on the apatite support than on alumina. One of the reasons might be the fact that the promoter Ni ions do not diffuse into the apatite structure as it is the case in alumina.
Rate constants for hydrogenolysis reaction of DMDS determined at 453 K over NiMo/apatite catalysts and reference NiMoP/Al2O3 catalyst.
Catalyst | MoO3 (wt.%) | NiO (wt.%) | Rate constant (L h−1 g−1) |
NiMo/AH | 6.6 | 1.9 | 28.3 |
NiMo/AHP-60 | 6.0 | 1.8 | 27.3 |
NiMo/AHP-54 | 5.7 | 2.2 | 18.7 |
NiMo/AHP-29 | 6.3 | 2.4 | 16.4 |
NiMoP/Al2O3 | 14.1 | 2,7 | 23.6 |
Catalytic results are in agreement with support characterization. AH and AHP-60 solids consist of Ca-deficient hydroxyapatite that allows a better dispersion of NiMo entities because of superficial HPO42− and surface defects, as reported by Elazarifi et al. [6,7]. Therefore, the low catalytic activity of NiMo/AHP-29 and NiMo/AHP-54 should be related to the presence of impurities as fluorine in phosphoric acid which prevents to synthesize Ca-deficient hydroxyapatite.
5 Conclusion
NiMo catalysts supported on apatite prepared with industrial phosphoric acid (29%, 54%, 60 wt.% P2O5) have been prepared and their catalytic performances have been measured in DMDS hydrogenolysis. The characterization results showed that the phosphoric acid purity directly affects the nature of the apatite supports and then the activity of the supported catalysts. Apatite prepared with phosphoric acid 60% has similar properties to those of the support prepared from diammonium hydrogenophosphate, showing that this phosphate solution could be replaced by a cheaper industrial product. In this case, synthesized apatites are Ca-deficient hydroxyapatites. It allows one to prepare more efficient NiMo-supported catalysts than an industrial NiMoP/Al2O3. The higher activity is due to the presence of superficial HPO42− species and surface defects which facilitate the dispersion of the NiMo active phase. The apatite-based NiMo catalysts, active in DMDS hydrogenolysis, are thought to be active in HDS reactions because it has been proved that the active sites were similar for both kinds of reactions.
Acknowledgements
The authors are grateful to the French–Moroccan committee CMIFM for financial support through the MA/06/145 Volubilis program, and to the Moroccan company OCP (Office Chérifien des Phosphates) for providing industrial phosphoric acids.