1 Introduction
The use of ionic liquids in polymerization processes makes it possible to enhance the activity of catalysts, to increase selectivity, and to simplify the separation of pure products with the repeated use of the ionic liquid and the catalyst. In the test processes [1], the yields of target products from low-molecular-weight oligomers to high-molecular-weight polymers can be optimized by varying reaction conditions (reaction time, temperature, and catalyst structure).
The polymers synthesized in ionic liquids can be easily separated and isolated in a maximally pure state. It was found that the isolated polymers contained an insignificant amount of ionic liquids, and it is not necessary to wash them with water. The probability of further transformations (i.e., isomerization) of the polymers is low because the process is simplified. Ionic liquids do not decompose upon treatment with water, and they can be used repeatedly. This reduces operational costs and is an important advantage of ionic liquids in terms of green chemistry.
2 Radiation-chemical synthesis of phosphorus-containing polymers with the participation of ionic liquids
Red phosphorus modifications are of considerable interest because these inorganic polymers can be widely used [2–4]. It is important to prepare red phosphorus under milder conditions because of the specific properties of white phosphorus (high reactivity, fire and explosion hazards, and toxicity). One of the most interesting techniques for initiating the synthesis of red phosphorus is a radiation-chemical technique. Its advantages and disadvantages can be determined in comparison with traditional thermal methods. However, it is evident that only radiation-induced initiation makes it possible to perform the polymerization of white phosphorus in nonaqueous media to prepare red phosphorus modified with solvent fragments. The formation of red phosphorus in various systems under the action of ionizing radiation has been studied [4–6].
Depending on the composition of the reaction mixture and the reaction conditions, the phosphorus content of the resulting samples can be as high as 96 wt %, the concentration of solvent fragments can vary to 40 wt %, and the self-ignition temperature can be 373–593 K. Based on the experimental data, a conclusion was made that the irradiation products were red phosphorus, a polymer phosphorus modification.
Phosphorus-containing polymers modified with solvent fragments can be synthesized by performing the polymerization of elemental phosphorus in various solvents and initiating systems.
Red phosphorus prepared by the γ-irradiation of a solution of white phosphorus in benzene (henceforth, it is referred to as a phosphorus-containing polymer (PCP)) exhibited properties comparable to those of commercial red phosphorus samples.
The radiation-initiated polymerization of elemental phosphorus in the presence of the following imidazolium and pyrrolidinium ionic liquids was studied [6]: 1-butyl-3-methylimidazolium triflate [BuMeIm]OTf, tetrafluoroborate [BuMeIm]BF4, and hexafluorophosphate [BuMeIm]PF6; 1-butyl-3-methylpyrrolidinium bistriflamide [BuMePyr]NTf2; and 1-hexyl-3-methylimidazolium tris(pentafluoroethyl)trifluorophosphate [HeMeIm](C2F5)3PF3. It was found that the rate of radiation-initiated polymerization of white phosphorus increased upon the addition of an ionic liquid (Table 1).
G-values (radiation-chemical yields) of PCPs (molecule P4)/(100 eV) in the presence of ionic liquids in a DMSO–benzene mixture (1:1) (T = 298 K, D• = 0.59 Gy/s) [6].
Ionic liquid | [BuMeIm] OTf | [BuMeIm] BF4 | [BuMeIm] PF6 | [EtMeIm] NTf2 | [BuMePyr] NTf2 | [НeMeIm] (C2F5)3PF3 | Without ionic liquid |
[P4]0/[IL] | 0.075 | 0.072 | 0.069 | 0.215 | 0.081 | 0.134 | – |
G-value of PCP | 455 ± 46 | 47 ± 5 | 69 ± 7 | 60 ± 6 | 31 ± 3 | 32 ± 3 | 8 ± 1 |
The structure and concentration of an ionic liquid in the system were found to affect considerably the rate of polymerization. The introduction of an ionic liquid into the P4–benzene–dimethyl sulfoxide (DMSO) system resulted in a increase in the rate of reaction and an increase in the conversion of P4; the formation of a PCP was a second-order reaction with respect to elemental phosphorus because, hypothetically, the formation of a complex between the ionic liquid and P4 was a rate-limiting step of the reaction [6].
The aim of this work was to study the effect of phosphonium ionic liquids on the radiation-initiated polymerization of elemental phosphorus, in comparison with imidazolium and pyrrolidinium ionic liquids.
3 Experimental
In this study, ionic liquid [Et3OcP]NTf2–triethyloctylphosphonium bis(trifluoromethylsulfonyl)imide [(C8H17)(C2H5)3P]+[N(SO2CF3)2]−(99.9% purity; Nippon Chemical, Japan) was used.
White phosphorus was purified in accordance with a modified procedure [7]. The solvents were purified using the standard procedures [8].
PCPs were synthesized using the procedure described below. 390 ml of benzene and 7.95 g of the elemental (white) phosphorus were placed into the retort (1000 ml) and stirred at ∼320 K until the white phosphorus was completely dissolved. Then 13 ml of the IL was added, while stirring, and after that 52 ml of propan-2-ol. The mixture was cooled in a water bath to room temperature (the solution became turbid), and filtered. The resulting solution was placed into the hermetically sealed reactor and irradiated using the irradiation installation MRCh-γ-100 (isotope 60Co, radiation absorbed dose rate D• = 0.30 Gy/s, irradiation time 15.5 hours, radiation absorbed dose D = 16.7 kGy).
The irradiated mixture presented itself as a yellowish liquid containing a fine dispersion of an orange precipitate. The precipitate was filtered off using a double-layered filter paper box, and the latter was placed into the Soxhlet apparatus, where the precipitate was washed out with 200 ml of benzene for 18 hours. After that, the washed-out phosphorus-containing polymer was dried in the desiccator under vacuum (water-jet pump) until the constant mass value was obtained.
The reaction products were characterized by elemental analysis, XPS data, IR spectroscopy (Bruker Tensor 27 M instrument), X-ray diffraction analysis (DRON-3 M diffractometer), scanning electron microscopy (SEM) and transmission electron microscopy (TEM) and NMR (31P, 1H)-spectroscopy. The micrographs were taken using Tesla BS 340 scanning electron microscope.
4 Results and discussion
The PCP prepared by the polymerization of white phosphorus in the propan-2-ol–benzene–phosphonium ionic liquid system (Table 2) was a finely dispersed orange powder. It was insoluble in accessible polar and nonpolar solvents; this is characteristic of previously described phosphorus-containing polymers and commercial red phosphorus [9,10].
Physicochemical properties of PCP samples.
Reaction system | Polymerization | Color | G (P4 units/100 eV) | Phosphorus content (%) | XRD data |
Benzene–propan-2-ol + ionic liquid ([EOP]NTf2) | In solution | Yellowish orange | 6 | 56 | X-ray amorphous |
The shape and morphology of PCP particles were studied on a Tesla BS 340 scanning electron microscope. A phosphorus-containing polymer prepared upon the irradiation of elemental phosphorus in benzene was used as a reference sample. Fig. 1 shows the micrograph of the PCP sample.
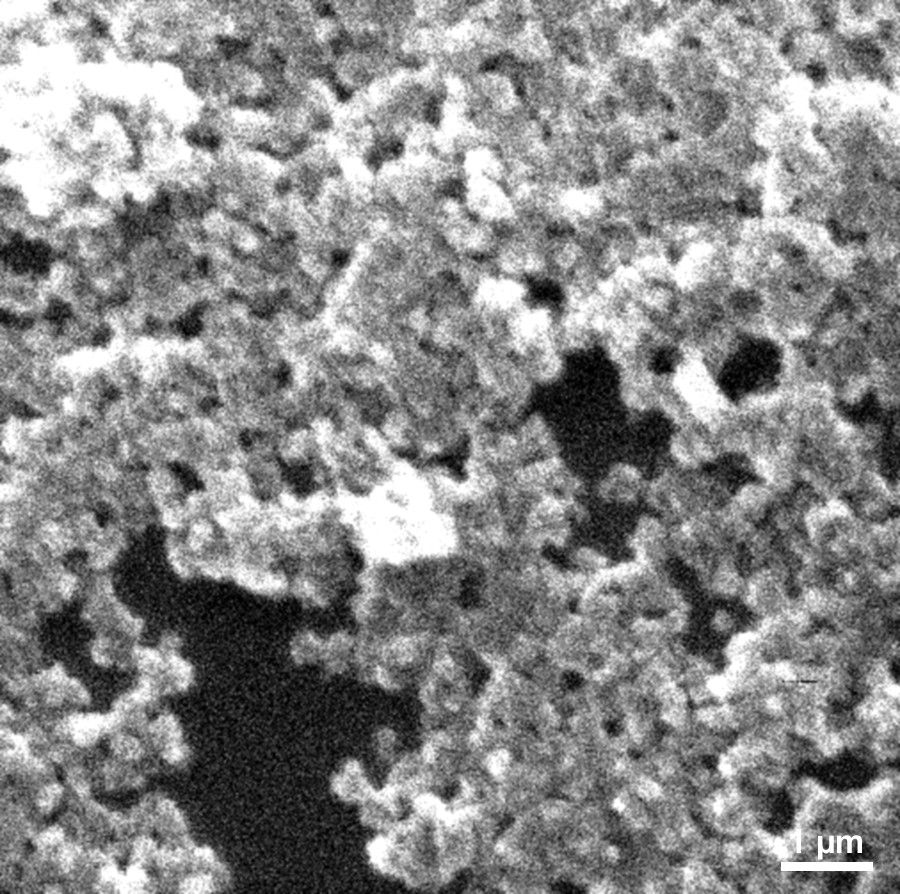
Micrograph of the PCP product prepared by the irradiation of a solution of white phosphorus in a benzene–propan-2-ol binary solvent with an ionic liquid added. Т = 298 K, D• = 0.59 Gy/s, D = 24.7 kGy, [Р4]0 = 0.013 М, and [ionic liquid]0 = 0.16 М.
As found by X-ray diffraction, PCP products formed both in pure benzene and in benzene–propan-2-ol are X-ray amorphous.
As found by morphological analysis and electron microscopy, particles with an average size of 0.25 μm were formed in the radiation polymerization process performed in a polar benzene–propan-2-ol solvent. These particles were much smaller than those formed in pure benzene (11 μm). This phenomenon can be due to the fact that a change from benzene to a more polar solvent is accompanied by a decrease in the solubility of the polymer and, finally, the polymer is released at its lower concentration in solution.
The similarity of the physicochemical properties (Table 3) of products formed in the polymerization of elemental phosphorus in various media suggests a common product formation mechanism.
Physicochemical properties of phosphorus polymer species prepared by the irradiation of a solution of white phosphorus in benzene and the product prepared in benzene–propan-2-ol with a phosphonium ionic liquid added.
Physicochemical properties | White phosphorus | Sample prepared in benzene solution | Sample prepared in the benzene–propan-2-ol–ionic liquid system |
Color | White | From light yellow to dark red | From light orange to yellow |
Solubility in water | 10−4 g/l | Insoluble | Insoluble |
Solubility in organic solvents | Soluble | Insoluble | Insoluble |
Interaction with nonoxidizing acids | Does not react | Does not react | Does not react |
Interaction with nitric acid | Reacts on heating | Reacts on heating | Reacts on heating |
Inflammability | Spontaneously flammable in air | Spontaneously inflammable; combustible with the release of heavy white smoke | Spontaneously inflammable; combustible with the release of heavy white smoke |
According to elemental analysis data, the synthesized PCP mainly consisted of phosphorus (56%); it also contained carbon (6%), hydrogen (3%), and oxygen (∼35%). The presence of carbon and hydrogen in the sample suggests the chemical incorporation of benzene fragments into the polymer structure of phosphorus (probably, because of chain termination by organic radicals generated by γ-radiation).
According to XPS data, the surface composition of the PCP particles was as follows: С, 68%; O, 22%; and P, 11%. Note that the carbon content was comparatively high. This can be due to the sorption of solvent molecules on the surface of PCP particles (Figs. 2–3).
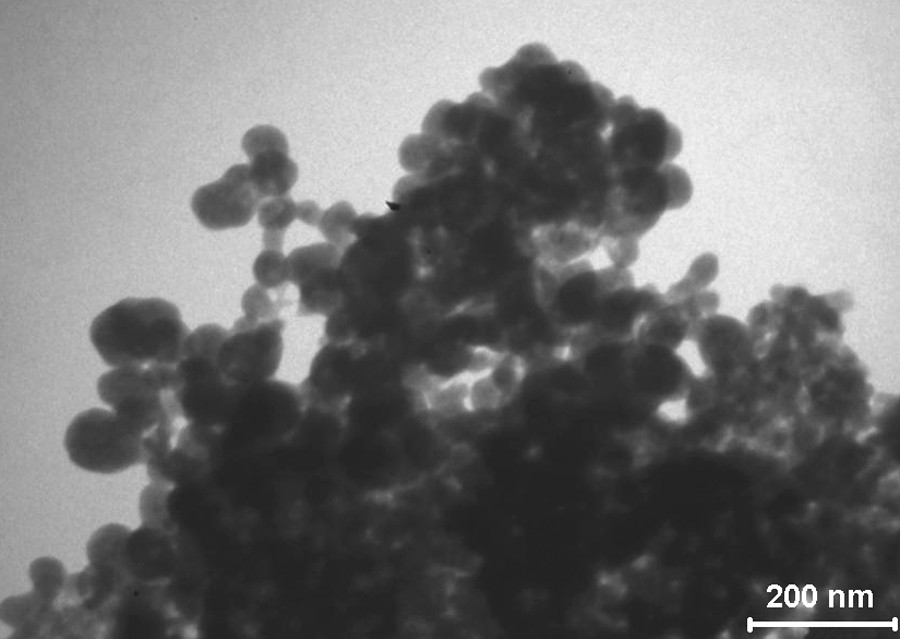
Micrographs of product samples prepared by the irradiation of a solution of white phosphorus in benzene. Т = 298 K, D• = 0.59 Gy/s, D = 24.7 kGy, and [Р4]0 = 0.013 М.
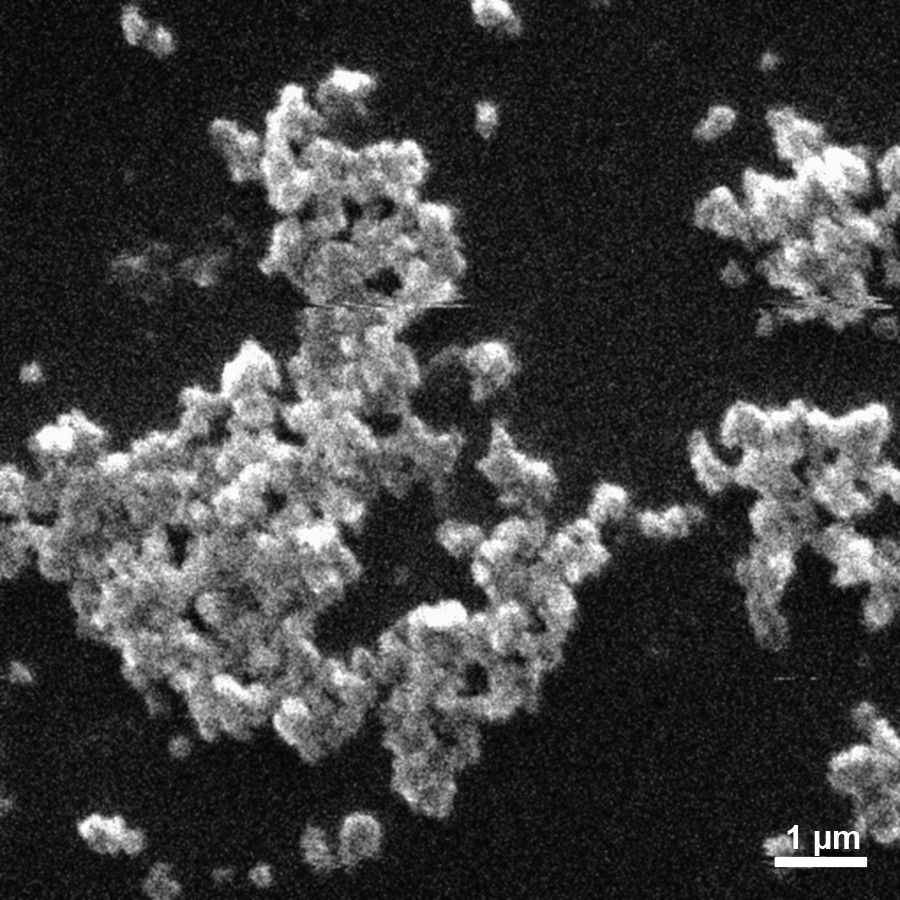
Micrographs of product samples prepared by the irradiation of a solution of white phosphorus in benzene–propan-2-ol with a phosphonium ionic liquid added. Т = 298 K, D• = 0.59 Gy/s, D = 24.7 kGy, [Р4]0 = 0.013 М, and [ionic liquid]0 = 0.16 М.
Scanning electron microscopy (SEM) and transmission electron microscopy (TEM) data for the PCP indicate that nanosized samples were prepared from solution. They contained spherical or elliptical particles of size 10–140 nm; in this case, 45% of the nanopartices were characterized by a size of 30–50 nm.
As estimated from the empirical formula of the polymer based on the amount of terminal solvent fragment groups, the degree of polymerization in solution was n = 5–6 ((Р4)5–6).
The IR spectra of the PCP exhibited absorption bands due to P–P stretching vibrations (490–520 cm−1) [11,12]. Absorption bands at 1400 and 1010 cm−1 suggest the chemical binding of organic fragments (benzene) to the phosphorus atoms of the resulting polymer [13].
The occurrence of oxygen in all of the synthesized PCP samples was due to the partial oxidation of the surface layers of polymeric phosphorus or the incorporation of oxygen-containing organic radicals, which initiated the polymerization process, into the polymer structure. This manifested itself in the appearance of absorption bands due to phosphorus-oxygen bonds in the IR spectra (Table 4): 1640 cm−1 (HP=O, HOP=O), 1160 cm−1 (ν P = O), and 1010 cm−1 (δ POC) [11–13]. The bands at 1010 and 1160 cm−1 due to the POC and Р = О bonds, respectively, exhibited the highest intensities.
Characteristic absorption bands in the IR spectra of PCP samples and their attribution [12,14,15].
Absorption band ν (cm−1) | Intensity | Attribution |
1640 | Medium | P(O)–OH, P(O)–H |
1400 | Weak | Р–C, Р–CPh |
1160 | Strong | Р=О |
1010 | Strong | (P)–O–C |
790–810 | Weak | Р–Н, Р–СН2 |
680–690 | Weak | Р–О–P |
490–520 | Medium | Р–P |
Table 5 summarizes the XPS spectra of the PCP samples. The XPS data support and supplement the results of instrumental physical studies to indicate the occurrence of P–P and P–C6H5 bonds and large amounts of oxygen-containing groups as the constituents of the PCP samples (Table 5).
XPS data for the PCP.
XPS line | Energy (eV) | Attribution according to the NIST XPS Database |
P 2p3/2 | 130.3 ± 0.3 | P–P C6H5–P |
P 2p3/2 | 132.8 ± 0.4 | O=P–C6H5 |
O 1s | 532.8 ± 0.2 | (O)P–H |
The NMR (31P) spectra of PCPs exhibited the presence of five types of phosphorus nuclei (Figs. 4–5; Table 6):
- • Singlet at δ∼0 ppm, that might be attributed to P–Ph group [16,17];
- • Doublet at δ≈7 ppm with JPH = 700–720 Hz, which might be attributed to P–H group;
- • Triplet at δ∼16 ppm with JPH = 550–590 Hz, which might be attributed to O=P–H or HO–P–H groups;
- • Wide multiplet (∼1500 Hz) with the centre at ∼30 ppm, which corresponds to P–P polymeric group;
- • Wide singlet at δ≈−450 (−460) ppm, attributed to the absorption of the P–P group in the white phosphorus [2,12,17].
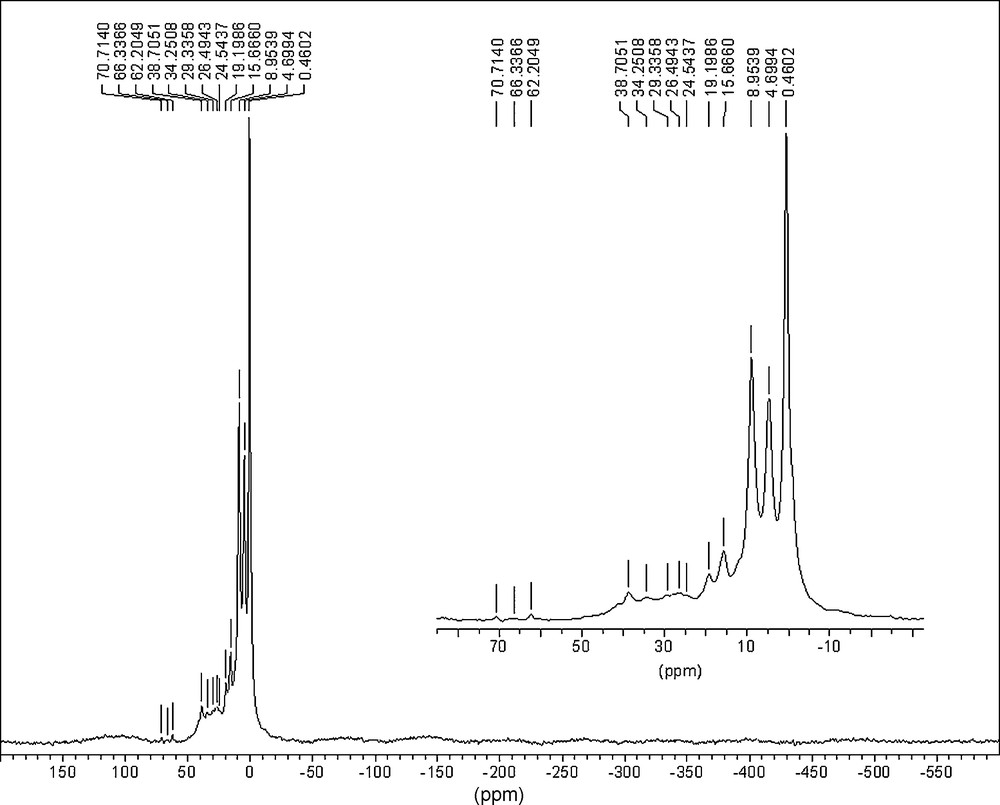
NMR (31P) spectrum of the PCP sample prepared by the gamma-irradiation of the solution of white phosphorus in the mixed solvent benzene–propan-2-ol–[EOP]NTf2 (D• = 0.30 Gy/s, D = 16.7 kGy).
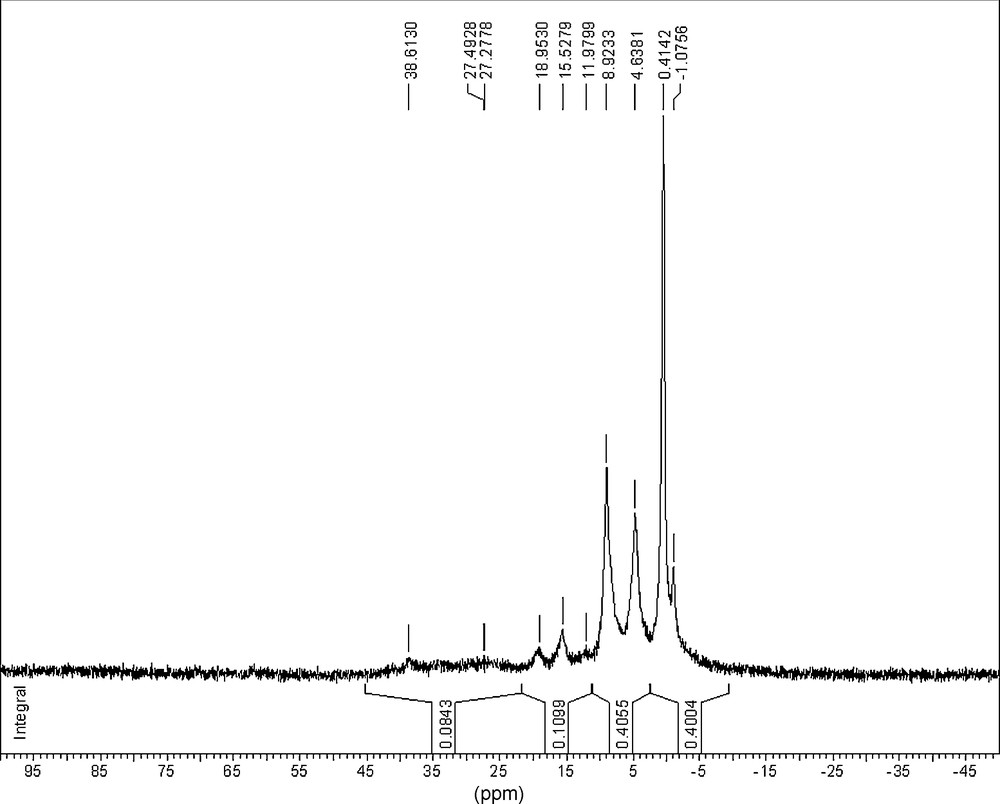
NMR (31P) spectrum (with the integrals of the signals) of the PCP sample prepared by the gamma-irradiation of the solution of white phosphorus in the mixed solvent benzene–propan-2-ol–[EOP]NTf2 (D• = 0.30 Gy/s, D = 16.7 kGy).
Characteristic signals in the NMR (31P) spectra of PCP samples and their attribution [2,12,17].
Chemical Shift, ppm | Multiplicity | JP-X (Hz) | Integral, arb. units | Attribution |
−1.1 ± 0.1 | Singlet | – | ||
0.4 ± 0.1 | Singlet | 0.400 | P–CPh | |
4.6 ± 0.1 8.9 ± 0.1 | Doublet | 700 ± 5 | 0.406 | P–H |
12.0 ± 0.1 15.5 ± 0.1 19.0 ± 0.1 | Triplet | 585 ± 5 | 0.110 | P–H(O) |
∼24–34 | Wide multiplet | 0.084 | P–Р | |
38.6 ± 0.1 | Singlet | P+–Alk |
The signals in the region 62–81 ppm were attributed to the side rotation lines of the signals in the region 0–20 ppm, described above (they are shifted from the main signals as far as 10 kHz and are characterized with the same multiplicity and spin-spin coupling constant).
The 1H NMR spectra of PCPs exhibited the presence of several types of protons (Fig. 6, Table 7):
- • the singlet (7.7 ppm) attributed to CPh–H;
- • the signal at 5.1 ppm, that might be attributed to P–H;
- • the singlet (4.8 ppm), attributed to the proton of the phosphinic group P(O)–H.
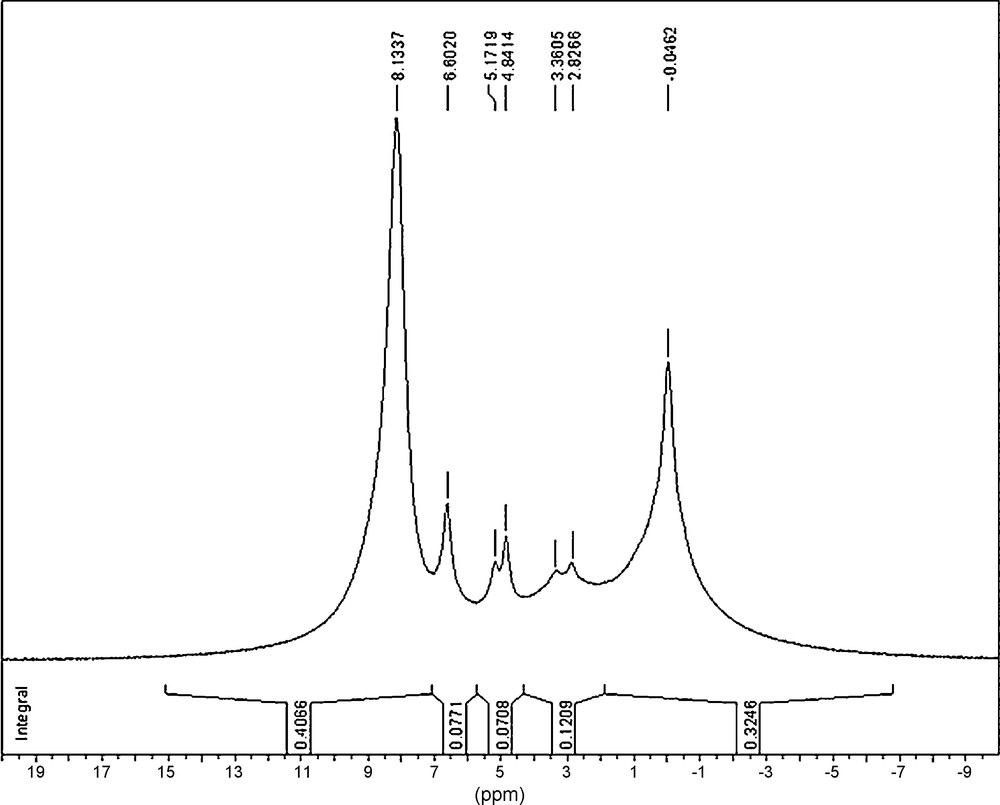
NMR (1H) spectrum (with the integrals of the signals) of the PCP sample prepared by the gamma-irradiation of the solution of white phosphorus in the mixed solvent benzene–propan-2-ol–[EOP]NTf2 (D• = 0.30 Gy/s, D = 16.7 kGy).
Characteristic signals in the NMR (1H) spectrum of PCP sample prepared by the gamma-irradiation of the solution of white phosphorus in the mixed solvent benzene-propan-2-ol-[(C8H17)(C2H5)3P]+[N(SO2CF3)2]− (D• = 0.30 Gy/s, D = 16.7 kGy) and their attribution [2,12,17].
Chemical shift (ppm) | Multiplicity | Integral, arb. units | Attribution |
−0.1 ± 0.1 | Singlet | 0.325 | (P)–H |
2.8 ± 0.1 | Doublet | 0.121 | (P)–H |
3.4 ± 0.1 | |||
4.8 ± 0.1 | Singlet | 0.071 | P(O)–H |
5.2 ± 0.1 | P–H | ||
6.6 ± 0.1 | Singlet | 0.077 | (P)–H |
7.7 ± 0.1 | Singlet | 0.407 | CPh–H |
The other signals were difficult to attribute, but one might suppose them to belong to protons bonded to phosphorus in different groups, presented in the polymeric phosphorus structure [18–21] (Table 7).
Thus, we determined the elemental composition of the synthesized PCPs, supported their polymeric character, and identified the main functional groups bound to phosphorus atoms.
5 Reactivity and industrial applicability of PCPs
Polymer phosphorus species can replace white phosphorus in organophosphorus synthesis reactions to compete with commercial red phosphorus. The synthesized samples were tested at A. Favorsky Institute of Chemistry, Russian Academy of Sciences, Siberian Branch (Irkutsk).
To evaluate the phosphorylating capacity of the synthesized PCPs, their interaction with α-methylstyrene in a superbasic medium (the Trofimov-Gusarova reaction, Fig. 7) was studied in comparison with commercial red phosphorus.
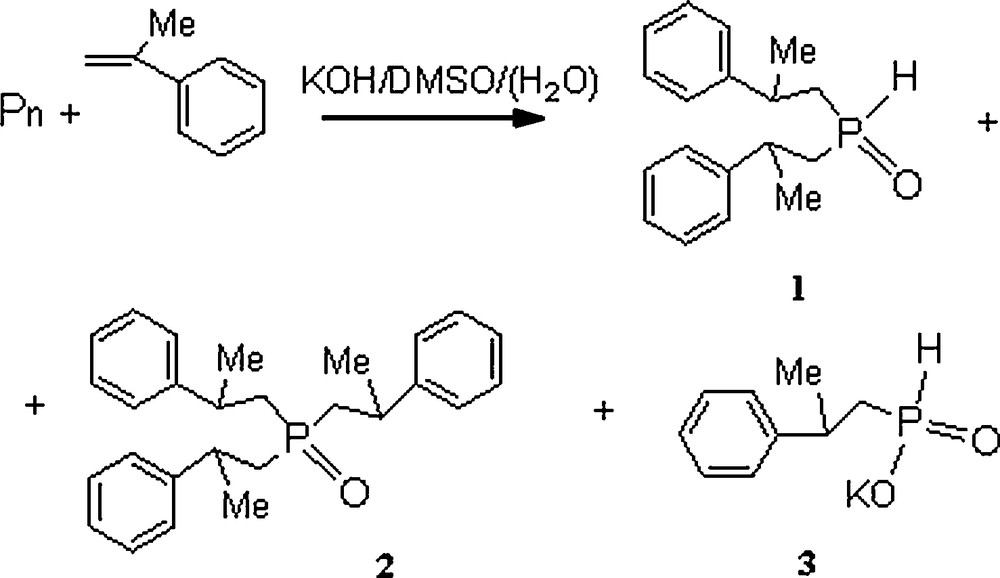
Reaction scheme of PCP with α-methylstyrene in a superbasic medium.
The reaction occurred on heating (393 K, 3 h) the reactants in the KOH–DMSO system with the use of the PCP to form bis(2-phenylpropyl)phosphine oxide 1, tris(2-phenylpropyl)phosphine oxide 2, and potassium (2-phenylpropyl)phosphinite 3 (Table 8). The conversion was 100 or 17% in terms of phosphorus or α-methylstyrene, respectively [22]. Commercial red phosphorus reacted less effectively with α-methylstyrene under analogous conditions to form tertiary phosphine oxide 2 in a yield of only 15% (in this case, the conversion of phosphorus or α-methylstyrene was 82 or 18%, respectively) (Table 8).
Activity of PCPs in organophosphorus synthesis.
Sample | Product yield (%) | ||
Commercial red phosphorus (GOST [State Standard] 8655–75) | PCP | P4 | |
Conversion | 83 | ∼100 | 100 |
Σ (1,2,3) | < 75 | 95 | 97 |
It is believed that the cleavage of red phosphorus by potassium hydroxide is the rate-limiting step because this step occurs in an almost heterogeneous system on the surface of a solid phase (the polymer molecule of red phosphorus is difficult to decompose):
Pn + OH– →P1OH + Pm– (l + m = n). |
Next, an attack at the double bond of α-methylstyrene took place with the consecutive P–P bond cleavage in the polymer molecule of red phosphorus.
Under conditions of this reaction, insignificant amounts of phosphine and potassium hypophosphite were formed by the interaction of elemental phosphorus with aqueous potassium hydroxide [23].
As can be seen in Table 8, the reactivity of the PCP sample in the reaction with α-methylstyrene was comparable with that of P4 and higher than that of commercial red phosphorus.
6 Conclusions
(1) Phosphorus-containing polymers were synthesized by polymerization in solution in the presence of phosphonium ionic liquids and characterized by a number of physicochemical techniques. The effect of phosphonium ionic liquids on the radiation-initiated polymerization of white phosphorus was studied.
(2) The synthesized nanosized PCPs were found to exhibit enhanced reactivity toward organophosphorus synthesis reactions, as compared with that of commercial red phosphorus.
Acknowledgements
This work was supported by the Russian Foundation for Basic Research (project no. 09-03-00493, and partly project no. 08-03-00251).
We are grateful to Dr. Katsuhiko Tsunashima (Organic Chemicals Division, Nippon Chemical Industrial Co., Japan) for providing us with the phosphonium ionic liquids.