1 Introduction
Phytosterol esters are currently gaining importance because of their recent recognition and application in food and nutraceutical industries due to their hypocholesterolemic effect favoring the reduction level of both serum LDL cholesterol and triglycerides [1,2], as well as in other areas such as cosmetics (as emulsifiers, anti-inflammatory and antioxidant compounds) [3]. Vegetable oils are the major source of phytosterols, which are found in their insaponifiable fraction. Phytosterols are plant-specific phytochemicals that are essential components of cell membranes. They are structurally related to cholesterol, although they differ in the complexity of their side chain, which is attached to the steroid ring. Over 40 phytosterols have been identified and among them the most common are β-sitosterol, campesterol and stigmasterol. Free sterols are poorly soluble in most foods (fats and oils), leading to problems with formulations. To overcome this drawback and improve their solubility and compatibility for incorporation in foods, they are usually esterified with fatty acids1,2,3,4. However, this esterification reaction requires the use of acid catalysts, which may favor dehydration of sterols to the side-product stigmastadienes. Strong mineral or organic bases (NaOH, KOH, NaOEt…) are usually used in transesterification reactions, but the difficulty to separate such homogeneous catalysts from the reaction medium, make them not well suited for an environmentally friendly catalytic process. Synthesis of phytosterol esters using biocatalysts in the presence of organic solvents and molecular sieves or other drying agents has been reported in various studies [4–8]. Plant steryl esters were also efficiently prepared from sterols via lipase-catalyzed esterification with fatty acids and by transesterification with fatty acid methyl esters or triacylglycerols [9]. Enzymatic transesterification reaction was recently successfully carried out in a packed bed continuous flow reactor with better environmental control [10].
With the objective of reducing waste formation and extraction steps, Pouilloux et al. [11] have shown that heterogeneous basic catalysts could be advantageously used in the transesterification of sitosterols with fatty methyl esters in the absence of solvent. The best phytosterol ester yield (above 75%) was obtained over magnesium or zinc oxides. However, acid sites, also present on MgO in high amounts along with the strong basic sites, favor the dehydration side-reaction (formation of stigmastadienes).
The aim of the present work was to study the selective synthesis of phytosterol esters from natural sterols and methyl esters (Fig. 1) by privileging a chemical process in the absence of any solvent using basic catalysts that are reusable, active, and more selective than the currently used homogeneous or heterogeneous catalysts. Among all the basic solids tested, we have found that less conventional catalysts such as lanthanum oxides proved highly efficient in terms of reaction yield and selectivity [12]. Basic solid materials are indeed of great importance in heterogeneous catalysis if the nature, quantity, distribution and strength of the basic sites can be monitored so as to yield active centers well adapted to a particular catalytic reaction.
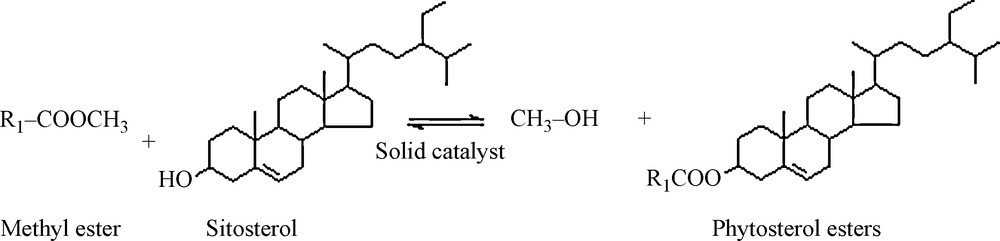
Reaction scheme for phytosterol esters selective synthesis.
Here, we wish to present our preliminary results devoted to the selection of a suitable Mg-containing catalyst (by comparison with La-based samples) and on the analysis of the influence of the physicochemical properties of such catalyst on its catalytic performances. It should be also mentioned that due to the far higher atomic weight of lanthanum than that of magnesium (MWLa/MWMg ratio = 5.8), it is consequently easier to generate a higher number of well dispersed magnesium active sites over a support, for a same weight content. Special attention was also dedicated to the stability in reaction and reusability of the alumina supported MgO catalyst.
2 Experimental
2.1 Catalyst preparation
The γ-alumina support (labeled as Al2O3-SG) was prepared by a sol-gel process following a published procedure [13], that we have modified concerning the hydrolysis and peptization steps. In our case, 1 mol of aluminum tri-sec-butoxide was hydrolyzed with 100 mol of water at 60 °C under stirring for 1 hour, followed by a peptization step with 0.1 mol of HCl (12 mol/L aqueous solution). The temperature of the solution was then increased to 80 °C while stirring during 1 hour until gel formation. The resulting gel was dried in an oven at 120 °C for 12 hours. The white powder was ground and calcined in air flow (10 L/h/g) during 5 hours at 500 °C (heating rate of 1 °C/min), so as to yield the transitional alumina, Al2O3-SG.
A reference γ-alumina sample supplied by Rhodia (170 m2/g) was also used for comparison as catalyst support (labeled commercial γ-Al2O3). The magnesium precursor was then inserted at room temperature into the alumina supports through wet impregnation. An alcoholic magnesium acetate solution (50 mL EtOH) was used to prepare the Mg-loaded alumina samples. The Al2O3 support (5 g) was mixed with the Mg acetate solution (0.14 mol/L) and the resulting mixture was stirred for 2 hours. The solvent was slowly evaporated at 50 °C, then the alumina impregnated solids were dried in air for 12 hours at 120 °C, before being activated up to 550 °C under flowing air for 8 hours (heating rate of 1 °C/min), so as to completely decompose the acetate anions and generate the corresponding supported MgO phase. The amount of Mg precursor was adjusted to obtain a final Mg content of 8 wt % after calcination. The resulting alumina-supported MgO samples were labeled as MgO-Al2O3-SG and MgO-γAl2O3.
Commercial lanthanum oxide (5 m2/g) supplied by Rhodia was also used for comparison as basic catalyst.
2.2 Characterization
Powder XRD patterns of the calcined alumina supported MgO materials were recorded on a Bruker D5005 diffractometer with monochromatized Cu Kα radiation (λ = 1.5418 Å) at 40 kV, 30 mA. The diffraction patterns were recorded in the 2θ values range 20–80° with a rate of 0.02°/sec and a step size of 2 seconds. Bulk chemical analysis for Mg and Al was achieved by ICP. The surface area and pore size analysis of the samples were carried out by adsorption-desorption of nitrogen on a Micromeritics ASAP 2010 instrument (−196 °C). Prior to N2 adsorption, the samples were degassed under vacuum at 90 °C for 1 hour, followed by a further heating at 300 °C for 6 hours. The BET equation was used to calculate the surface area from adsorption data obtained at p/p0 between 0.05 and 0.2. The pore diameter was evaluated at the maximum of the pore size distribution determined by the BJH method (adsorption).
TG-DTA study of the decomposition sequence in flowing air of the alumina supported magnesium acetate was performed on a SDT 2926 microbalance (TA instruments) from 20 to 800 °C at a heating rate of 10 °C/min.
TG-MS measurements were carried out with a TGA apparatus SDT Q600 from TA Instruments coupled with a mass spectrometer Prisma QMS 200 (Balzers). Known amounts of samples were disposed in an alumina crucible and heated from 25 to 750 °C with a 10 °C/min temperature slope under a N2 flow rate of 100 mL/min. Mass spectroscopy measurements were performed at m/z 18 (water) and m/z 44 (CO2).
IR spectra were recorded on a Nicolet Nexus spectrometer with a spectral resolution of 4/cm. Samples were pressed as self-supported wafers. Discs of 10 mg/cm2 were prepared and treated directly in the cell. The samples were evacuated up to 240 °C (10 °C/min) for 30 minutes and then brought back to room temperature prior to contact of probe molecules. Pyridine and CO were introduced on the activated samples at room temperature.
2.3 Catalytic experiments
The transesterification of β-sitosterol with methyl dodecanoate was carried out at atmospheric pressure under a nitrogen flow in a Pyrex batch reactor equipped with a mechanical stirrer. The nitrogen flow was used instead of air flow in order to favor the elimination of the co-product (methanol) generated during the transesterification reaction, as well as to avoid parallel oxidation reactions. In accordance with previous data [11], the N2 flow was fixed to 5 L/h. Under such conditions the yield to phytosterol esters reached a maximum, while no removal of methyl dodecanoate was observed during all experiments.
First, 3.5 × 10−2 mol of sterol and 3.5 × 10−2 mol of methyl dodecanoate were heated to 240 °C. Then the catalyst (5 mg mol%) was added to the mixture. The analysis of the reaction products was performed on a GPC equipped with a FID detector and an on-column injector. The percentage of each compound was determined using hexadecane as an internal standard. The conversions of methyl dodecanoate and β-sitosterol were followed as functions of the time on stream of the microsamples withdrawn periodically and evaluated with respect to the initial and final contents of fatty methyl ester and free sterol in the solution.
3 Results and discussion
3.1 Catalysts characterization
The wide-angle X-ray diffractograms of the calcined sol-gel and commercial alumina support samples (Al2O3-SG and commercial Al2O3) showed the characteristic diffraction lines expected for γ-alumina (JCPDS file 050-0741). However, smaller full width at half maximum (FWHM) and higher diffraction peaks intensity were noticed for the commercial γ-Al2O3 compound, suggesting large crystal size variations among both samples. Table 1 summarizes the textural properties of the parent alumina host support and the MgO impregnated samples. The alumina sample prepared through the sol-gel procedure exhibited a specific surface area of 300 m2/g with a pore volume of 0.28 cm3/g and a mean pore size of 3.5 nm, determined by applying the BJH model to the adsorption branch. By contrast, decreased specific surface area (170 m2/g) and larger mean pore diameter (13 nm) was observed for the commercial γ-alumina sample, indicating that larger and more crystallized alumina particles are present, as confirmed by the XRD pattern.
Textural properties of various alumina-supported MgO samples and of their corresponding alumina supports.
Sample | Mg/Al | SBET (m2/g) | Dpore (nm) | Total pore volume (cm3/g) |
Al2O3-SG | 0 | 300 | 3.5 | 0.28 |
MgO-Al2O3-SG | 0.23 | 204 | 4.0 | 0.22 |
Commercial γ-Al2O3 | 0 | 170 | 13 | 0.70 |
MgO-γAl2O3 | 0.21 | 157 | 13 | 0.63 |
The two alumina-supported MgO samples displayed well-resolved XRD lines at 2θ ∼ 42.8° and ∼ 62.2°, readily attributed to crystalline MgO (periclase) and suggesting that the size of the particles formed are large enough to be detected by XRD (Fig. 2). Visual comparison with the stick diagrams summarizing information of JCPDS files indicated the presence of only MgO (JCPDS file 86-1627). Indeed, no other diffraction lines due to possible spinel MgAl2O4 phase were visible on the XRD patterns. In agreement with previously literature reported results [13], a decrease in specific surface area was logically observed after the magnesium precursor impregnation step, from 300 to 204 m2 g−1 (sample MgO-Al2O3-SG) and from 170 to 157 (MgO-γAl2O3), respectively. This corresponds to a specific surface area decrease of 32 and 8% with respect to that of the parent alumina support.

Wide angle XRD patterns of the alumina-supported MgO samples (MgO-Al2O3-SG and MgO-γAl2O3) calcined at 550 °C. γ: γ-Al2O3, P: periclase (MgO).
Fig. 3 compares the TG-DTA patterns of magnesium acetate impregnated on both sol-gel and commercial γ-alumina samples. It is clearly seen from both TG traces and DTA endotherms (non oxidative degradation of acetate ions at low temperature) and exotherms (at higher temperature), that the decomposition sequences in oxidative atmosphere of the impregnated Mg acetate followed a similar trend for both alumina supports. The main DTA exotherms between 270 and 500 °C correspond to the oxidative degradation of the acetate anions, which are in agreement with that of the unsupported magnesium precursor (TG-DTA traces not shown). The DTA peak observed at ∼ 535–540 °C for the impregnated magnesium acetate on the sol-gel alumina sample was attributed to the decomposition of magnesium carbonate species. As for lanthanum oxides, magnesium is also well-known to be sensitive to CO2 contained in the ambient atmosphere, leading to partial surface carbonation. Such TG-DTA results were confirmed by in situ TGA-DTA coupled to mass spectroscopy experiments (Fig. 4). Thermo-gravimetric analyses combined with mass spectroscopy measurements (TGA-MS) of water (m/z 18) and CO2 (m/z 44) signals indicated that a simultaneous production of carbon dioxide and water occurred in the temperature range of 300–500 °C. The first peak (300–365 °C) obviously corresponds to the decomposition of anhydrous acetate, while the second peak between 370 and 500 °C can be related to the decomposition of hydrogenocarbonate species. TGA-MS experiments at m/z 44 in the temperature range of 500–555 °C only showed the production of carbon dioxide, while no corresponding release of water was observed. This CO2 production process is associated with a low weight loss and evidences the decomposition of superficial carbonate species.
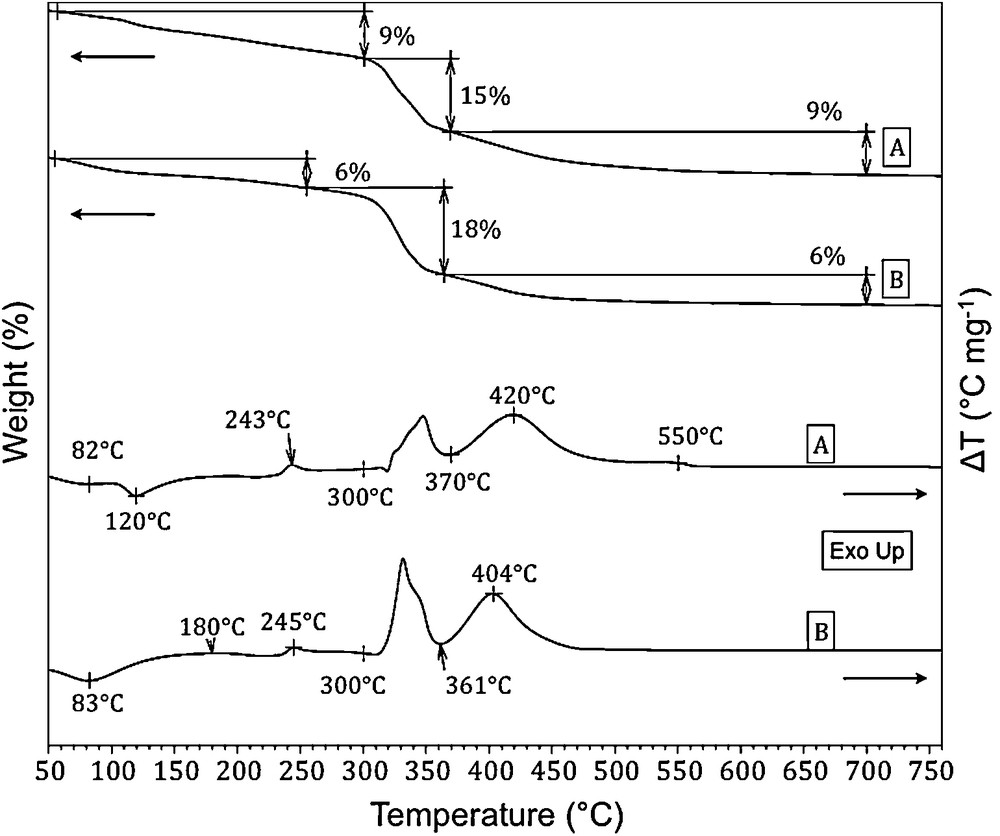
TG (up) and DTA (down) traces of magnesium acetate in interaction with (A) the sol-gel alumina after impregnation (uncalcined MgO-Al2O3-SG sample) and (B) with the commercial γ-alumina (uncalcined MgO-γAl2O3 sample).

TGA-MS measurements of uncalcined MgO-Al2O3-SG sample recorded under nitrogen flow at 10 °C/min for m/z 18 (H2O) and m/z 44 (CO2). For the sake of clarity, the DTA trace of magnesium acetate in interaction with the sol-gel alumina after impregnation is also indicated.
The resulting decomposition sequence in oxidative atmosphere of the supported Mg acetate is summarized by the following equations:
(1) |
(2) |
(3) |
3.2 Catalytic behavior of various alumina-supported MgO samples
When the transesterification of methyl dodecanoate with β-sitosterol was performed in the absence of catalyst, the main products are the phytosterol ester and the conjugated diene compound (stigmasta-3,5-diene) which is the side-product of sterol dehydration. Since the selectivity to the phytosterol ester and diene are close to 50%, there is a competition between the transesterification reaction and the sterol dehydration due to the high temperature and the resulting phytosterol ester yield is very low (15%).
In order to replace homogeneous catalysts by basic and reusable solids, two Mg-containing alumina compounds (MgO-Al2O3-SG and MgO-γAl2O3) were considered for the selective synthesis of phytosterol esters and compared with lanthanum oxide, which was previously used [12].
We first checked that both alumina supports (Al2O3-SG and commercial γ-Al2O3) were not selective to phytosterol esters (Table 2). While high sterol conversion values were obtained (∼92%) due to the intrinsic alumina basic sites, the side-reaction of sterol dehydration was strongly favored, leading to phytosterol esters yield ranging from 9 to 49% for the commercial and sol-gel alumina, respectively. The fact that the selectivity to sterol esters exhibited by the alumina prepared through the sol-gel route was higher than that of the commercial alumina, may account for different surface properties (nature and strength of acidic centers) due to distinct synthesis and activation procedures.
Synthesis of phytosterol esters from methyl dodecanoate and β-sitosterol in the presence of various basic oxide catalysts and pure alumina supports.
Sample | Conversion | Selectivity | Yield (%) | Activity (mmol/h/g) | |
β-sitosterol (%) | Sterol esters (%) | Dienes (%) | |||
Commercial γ-Al2O3 | 91 | 9 | 91 | 8 | 9 |
Al2O3-SG | 92 | 53 | 47 | 49 | 20 |
MgO-γAl2O3 | 97 | 56 | 44 | 54 | 36 |
MgO-Al2O3-SG | 98 | 95 | 5 | 93 | 30 |
La2O3 | 93 | 88 | 12 | 83 | 43 |
MgOa | 98 | 80 | 20 | 78 | 21 |
a From reference [11].
To our delight, we found that the sol-gel MgO-containing alumina sample (MgO-Al2O3-SG) was far more selective (95%) than sole MgO or supported MgO on the commercial alumina catalyst (56%), affording the desired product with 93% yield (Table 2, Fig. 5). We first assumed that such difference of behavior could originate from variation in the nature, number, location and possibly strength of the acidic and basic catalytic centers between the two alumina-supported MgO samples. The determination of the acidity of basic samples is crucial, because the acid sites present can be responsible for undesired side-reaction during the transesterification of natural sterols with fatty methyl esters. Indeed, as already observed with unsupported MgO [11], the presence of acid sites, also present on MgO in high amounts along with the strong basic sites, was shown to favor the formation of diene by-products through a sterol dehydration side reaction. The assessment of the surface acidity of the Mg-containing alumina samples was carried out by infrared spectroscopy of adsorbed probe molecules. IR-sensitive probe molecules, such as pyridine (py), are routinely used to determine the surface acidity of metal oxides. It could form coordinated species on Lewis acid sites (pyL) and pyridinium ions on protonic sites (pyH+), whose corresponding spectra are markedly different from each other. Pyridine adsorption was performed on samples evacuated at low temperature (240 °C) in order to work under conditions similar to those used during the catalytic tests. However, in our case, pyridine was not suited to unravel the number, location and strength of both surface acid sites (pyL and pyH+), because the useful pyridine absorption region (1650–1300 cm−1) was completely obscured by the signal of carbonate species present on the surface of the alumina supported MgO samples. CO was also used as probe molecule to evaluate the acidity of the samples. Unfortunately, CO was shown to be too weakly bound on the samples activated at 240 °C and proved to be inappropriate to characterize the acid properties of the samples in these conditions.
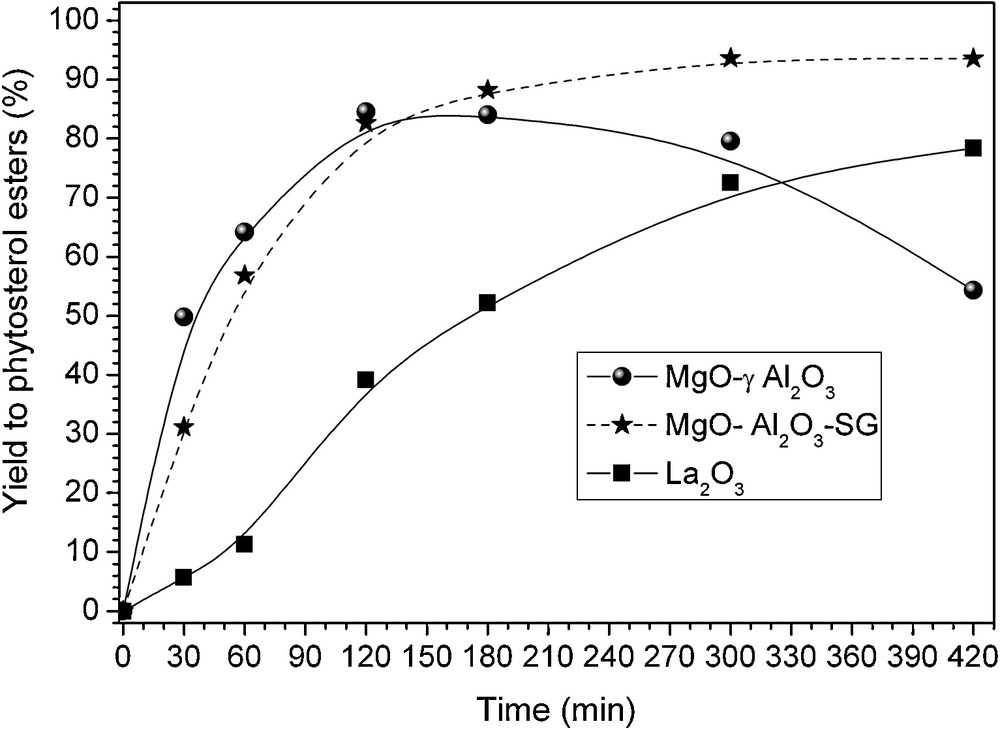
Phytosterol esters yield as a function of time on stream over various basic oxides: () MgO-Al2O3-SG sample, () La2O3 sample, () MgO-γAl2O3 sample.
Using such a low activation temperature typically results in the presence of high amounts of impurities (residual carbonates, hydrated catalyst surface…) on the surface of the catalysts, which hinders further analysis of the samples with common IR acidity probes such as CO and pyridine.
With the aim of understanding the role of acid sites onto the catalyst surface on the occurrence of the side reaction during the transesterification of natural sterols with fatty methyl esters, we next investigated the formation of the conjugated diene by-products from a mechanistic point of view. The reaction was first performed in the presence of the sole sterol and the commercial alumina-supported MgO catalyst (without the fatty methyl ester). In that case, sterol degradation was observed resulting in the formation of diene compounds. Such formation of by-products was also detected when the reaction was carried out with free phytosterol esters and the MgO-γAl2O3 catalyst, indicating a possible degradation of the main product during the catalytic reaction. This indicates that the fatty methyl ester is highly adsorbed on the catalyst surface onto the active sites that are responsible for the formation of dienes by dehydration of sterol or sterol esters transformation. Consequently, in the course of the reaction, the coverage of MgO-γAl2O3 catalyst with free sterols and sterol esters increased drastically with respect to that with methyl dodecanoate, thereby resulting in a significant formation of conjugated diene by-products especially for high conversion rate values (i.e. 80% for MgO-γAl2O3 sample). Note that the side reaction was substantially limited when the reaction was conducted in the presence of the MgO-Al2O3-SG catalyst (formation of side compounds not exceeding 5 wt%).
While the catalytic activity of the lanthanum oxide sample is far higher than that of the Mg-containing alumina catalysts, the corresponding phytosterol esters selectivity and yield were significantly lower (88 and 83%, respectively), indicating that the most efficient catalyst is the supported magnesium oxide on the alumina prepared by the sol-gel procedure.
On the course of our work, we next investigated the influence of the calcination temperature on the catalytic behavior of the alumina-supported MgO material prepared by the sol-gel route. The MgO-Al2O3-SG sample was calcined at a temperature varying between 450 and 650 °C under flowing air. The catalytic results are indicated in Table 3 with phytosterol esters selectivity values expressed at 80% sterol conversion. The highest selectivity to phytosterol esters value was obtained for the catalyst calcined at 550 °C (99%) compared with 97 and 92% for the samples thermally treated at 450 and 650 °C, respectively. While all samples exhibited a similar (initial) catalytic activity (Table 3), the results reported in Fig. 6 showed major changes in the phytosterol esters selectivity as a function of sterol conversion. Indeed, the selectivity of the MgO-Al2O3-SG sample calcined at 450 °C markedly decreased between 80 and 100% sterol conversion, by contrast to that of the corresponding sample calcined at 550 °C (very slight variation from 99 to 95%). A significant drop of selectivity was still observed for the alumina-supported MgO catalyst calcined at 650 °C, with a selectivity value ended at ∼84%. Such results strongly suggest that the calcination temperature impacts on the nature, strength and location of the basic sites at the catalyst surface. It was already mentioned in previous studies that the increase of calcination temperature resulted in a decrease of the catalyst specific surface area, but more importantly in the decrease of the amount of basic centers of various magnesium oxides [14–17]. Moreover, we have also observed that the calcination temperature led to a strong modification of the surface properties of various La-based compounds used in the transesterification reaction (of the fatty methyl ester with β-sitosterol), with as a result a strong decrease of the resulting selectivity and yield to phytosterol esters [18].
Synthesis of phytosterol esters from methyl dodecanoate and β-sitosterol in the presence of the alumina-supported MgO catalyst (MgO-Al2O3-SG). Influence of the calcination temperature.
Calcination temperature (°C) | Selectivitya Sitosterol esters (%) | Yield (%) | Activity (mmol/h/g) |
450 | 97 | 77 | 32 |
550 | 99 | 80 | 30 |
650 | 92 | 74 | 30 |
a At 80% conversion.

Phytosterol esters selectivity as a function of sterol conversion over MgO-Al2O3-SG sample calcined at different temperature: () 450 °C, () 550 °C, () 650 °C.
As already mentioned in the characterization section, the MgO-Al2O3-SG sample calcined at 550 °C exhibited dispersed magnesium oxide particles on the porous alumina surface. However, in the particular case of the compound calcined at the highest temperature (650 °C), the presence of a small fraction of MgAl2O4 phase, along with the supported MgO nanoparticles, was also observed in the corresponding XRD pattern (not shown). Such spinel phase obviously stemmed from a stronger acid-base interaction between the two oxidic MgO and Al2O3 species (amphoteric character) favored by the higher thermal treatment temperature, confirming that an adequate calcination temperature is necessary for achieving appropriate catalyst acid-base properties well adapted for a particular catalytic reaction.
3.3 Recycling study
Performing the transesterification reaction by heterogeneous catalysis provides solid catalyst reusability and, hence, reduces operational costs by facilitating easy separation of products, as well as improves industrial processes by avoiding wax formation.
To prove the efficiency of the catalyst, a series of four tests was performed with the MgO-Al2O3-SG sample calcined at 650 °C. At the end of the first test, the catalyst was centrifuged and washed thoroughly with 2-methoxyethyl-ether, before being reintroduced inside the reactor without having been calcined. After three cycles of reaction, the catalytic reaction behavior (sterols conversion, phytosterol esters selectivity and yield) was shown to be totally similar, confirming that MgO-Al2O3-SG is a stable and reusable catalyst. As indicated in Table 4, the phytosterol esters selectivity at 80% conversion varied from 92 to 95% with analogous yield trend (74–77%). Such behavior was already observed by Pouilloux et al. [11] with sole MgO or ZnO, but in that case a significant amount of dienes by-products was generated over MgO and in the case of ZnO, the catalytic activity was lower than that obtained with sol-gel alumina-supported MgO.
Synthesis of phytosterol esters from methyl dodecanoate and β-sitosterol in the presence of the alumina-supported MgO catalyst calcined at 650 °C (MgO-Al2O3-SG-C650). Recycling study.
Run | Selectivitya Sitosterol esters (%) | Yield (%) |
1 (Fresh catalyst) | 92 | 74 |
2 | 95 | 77 |
3 | 95 | 77 |
4b | 94 | 75 |
a At 80% conversion.
b Recovered catalyst after the first cycle, then calcined at 650 °C before being reintroduced inside the reactor.
A recycle test was also carried out after having regenerated the catalyst by calcination at 650 °C after the first reaction run before reintroduced the recovered catalyst inside the reactor. Here again, no differences in the catalytic properties were observed compared with that of the first run (Table 4).
In all cases, the concentration of leached magnesium species was extremely low (variation between 0.005 and 0.011 wt%), confirming that the Mg-based active sites were stabilized and strongly retained onto the porous alumina surface due to a strong acid-base interaction. Such performances are indeed required for efficient sterols conversion and for a reduced contamination of residues by alkaline-earth metal ions stemming from leached catalysts.
4 Conclusions
The synthesis of phytosterol esters from transesterification of a fatty methyl ester (dodecanoate) with β-sitosterol was carried out in the presence of basic solid catalysts, such as alumina supported magnesium oxides, differing by their synthesis procedure. Supported MgO on the alumina prepared by the sol-gel procedure (MgO-Al2O3-SG) proved particularly active and selective to phytosterol esters compared with sole MgO or with MgO supported on a commercial γ-Al2O3. The competition between the transesterification reaction and the side reaction of dehydration of sterol was strongly inhibited, resulting in phytosterol ester selectivity of 95%. The high conversion and selectivity values displayed by that catalyst led to an increased phytosterol ester yield (93%), compared with other basic oxide catalysts (MgO, La2O3) previously used. The most relevant results were obtained with the MgO-Al2O3-SG catalyst calcined at 550 °C. For higher calcination temperature, the appearance of a secondary spinel phase (MgAl2O4) onto the surface of the porous alumina support, resulted in a decrease of phytosterol esters selectivity and yield, owing to a modification of the catalyst acidobasic properties. After four reaction cycles, the catalytic properties were unchanged since the selectivity to phytosterol esters was above 94% with extremely low leached Mg species, thereby confirming that the MgO-containing alumina compound is a very stable and reusable catalyst, which is also a crucial property in catalysis.
Acknowledgements
The authors warmly thank Expanscience Laboratory for constructive discussions and for their continuous support. S. R.-M. gratefully acknowledges CONACyT Mexico for his PhD research grant (no. 172373/217762) at the University of Poitiers.
1 M.P. Van Amerogen, L.C. Lievense, C. Van Hoosten, World Patent, WO 98/01126, 15.01.98, Unilever.
2 I. Wester, J. Ekblom, World Patent, WO 99/56558, 11.11.99, Raisio Benecol Oy.
3 T. Miettinen, H. Vanhanen, I. Wester, US patent, 5 502 045, 26.03.96, Raision Tehtaat Oy.
4 N. Milstein, M. Biermann, P. Leidl, R. Von Kreis, World Patent, WO 99/30569, 24.06.99, Henkel Corp.