1 Introduction
Synthesis of macrocyclic molecules and their applications as chelating agents for separation and/or determination of toxic metal ions have been reported [1–4]. These systems also possess bacteriostatic properties in vitro and facilitate the iron mobilization from 59Fe labeled reticulocytes [5]. Nanomers, e.g. macrocyclic ligands allow selective complexation and extraction of metallic cations and anions of environmental importance [6–11].
Recently, nanomeric molecules and macrocyclic transition metal complexes in which structural changes have made to the basic crown ethers in an attempt to enhance the selectivity of such class of compounds towards metal ions and catalysis [12–14]. Crown ethers of Schiff bases and their complexes (Fig. 1) have great potential to generate novel metabolites and act as fungitoxic than free ligands [14]. These compounds could also be used as selective agents for the maintenance of animal or human health from infections [15–17].
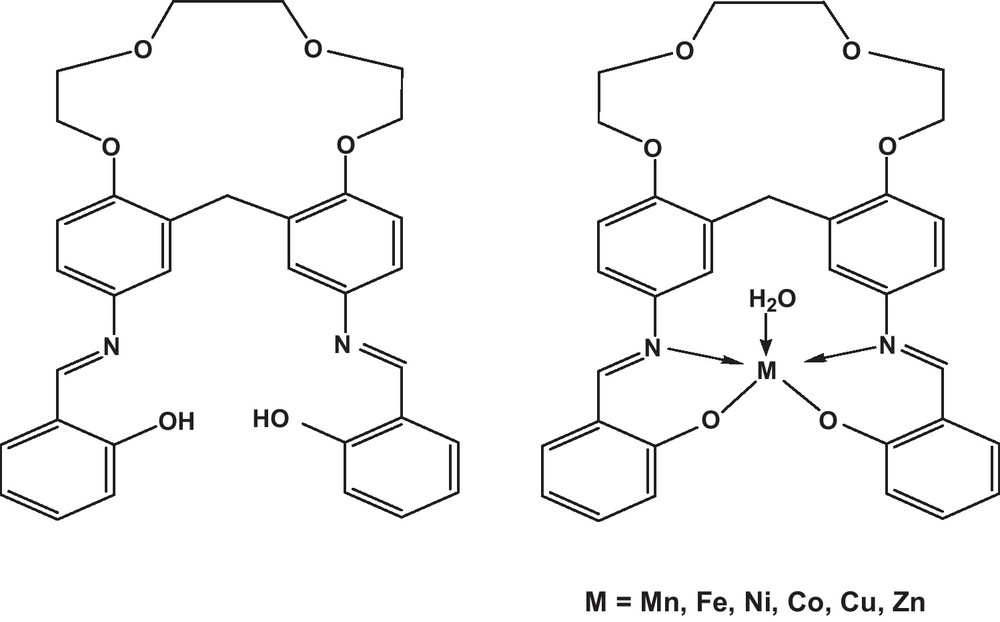
Macrocyclic transition metal complexes.
Nanomeric systems as strain allowed have been synthesized from heterocyclization of aryl - 1,3-dicarbox -aldehyde with 2-acetylpyridine in ethanolic sodium hydroxide. The resultant compound was refluxed with ammonium acetate-glacial acetic acid afforded 1,3-bis-compound 1 [17]. Reaction of compound 1 with RuCl3.xH2O in successive steps has produced a type of nanomer (Fig. 2) [17].
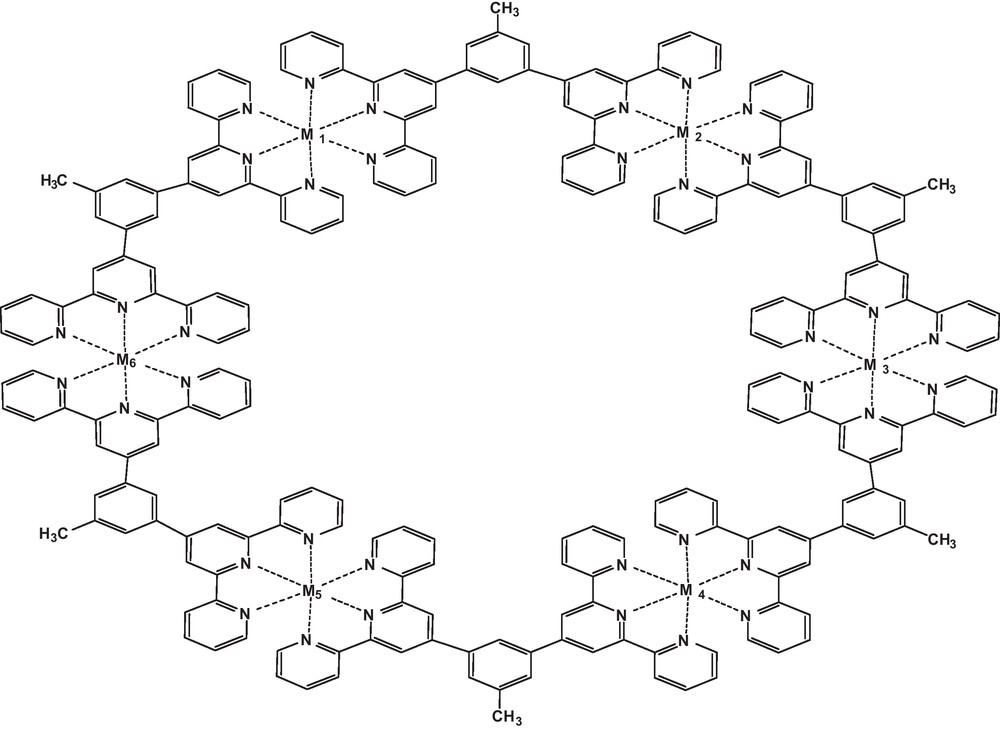
Formation of strain allowed nanomeric species.
The compounds 3-thioxo -1,2,4–triazines and their macrocyclic Schiff bases have great importance as medicinal and pharmacological agents [18–21]. Blanco et al., 2002 [22] have reported the nanomers derived from thiosemicarbazide and a cyclic bicarbonyl compounds. Therefore, the present article is focused on:
- • synthesis of some new nanomeric sulfur compounds derived from thiosemicarbazide and acyclic bicarbonyl compounds;
- • testing their toxicity properties as molluscicidal agents against Biomophalaria Alexandrina Snails;
- • studying the redox behavior of selected compounds for use as chelating agents in stripping voltametric analysis of arsenic;
- • application of compound 1 for separation of arsenic (III) & (V) species from industrial wastewater.
2 Results and discussion
2.1 Chemistry
In continuation to our previous work on the preparation of sulfur organic compounds [18–21] to be used excellent biocidal reagents, the present investigation reports the preparation and spectroscopic characterization of macromolecules as nanomer systems to be used as Molluscicidal agents against Biomophalaria Alexandriaa Snails responsible for Bilharzias. Thus, treatment of methanolic acyclic 1,2–bicarbonyl compounds such as benzil, 4,4′–dimethoxybenzil and 4,4′ -difluorobenzil with methanolic thiosemicarbazide in hot concentrated HCl with stirring afforded 5- methoxy -5,6-diaryl-4,5-dihydro-1,2,4- triazine -3-thiones (1-3) (Scheme 1). The compounds were characterized by elemental analysis and spectral measurements.
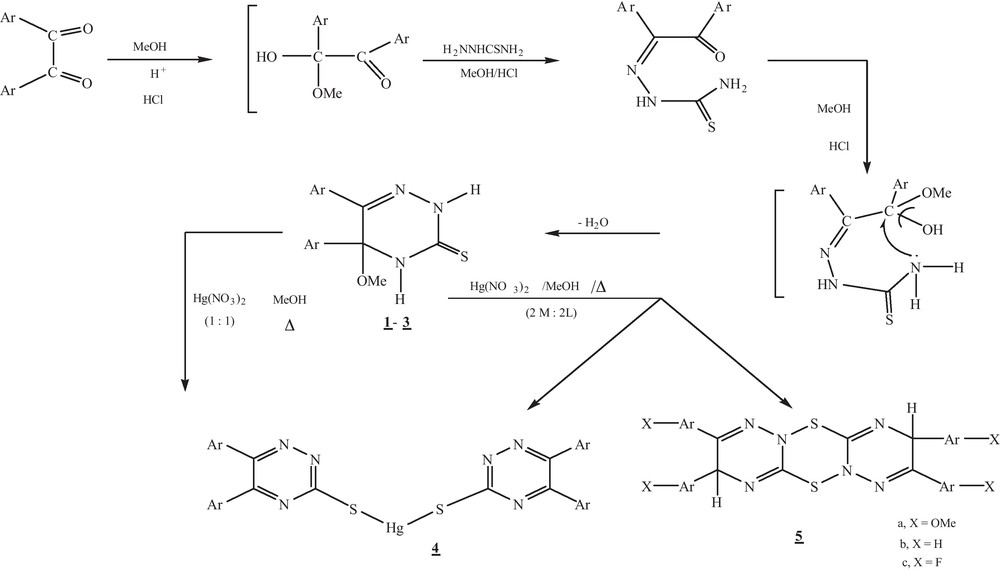
Formation of 1–5.
IR spectra of compound 1 in solid state and in chloroform showed the disappearance of NH and/or SH groups in the solution suggesting formation of a type of oxidation and/or complex formation products on treatment with HgCl2. Structure of compound 1 was deduced from elemental analysis and spectral data as given below:
- • UV absorption spectrum in DMF recorded λmax at 325, 280 and 210 nm assigned to due to n–π, π -π and n-sigma electronic transitions;
- • IR spectrum showed characteristic bands at IR: ν cm−1 3180 (NH), 2946 and 2835 (aliphatic CH), 1488 and 1446 (deformation of CH3), 1056 (C-O-Me), 976 and 886 cm−1 (phenyl groups);
- • Mass spectrometry recorded the molecular ion M+ at m/z 298 (12.5), M + 1 at 299 (3.8) with a base peak at m/z 77 (Scheme 2).
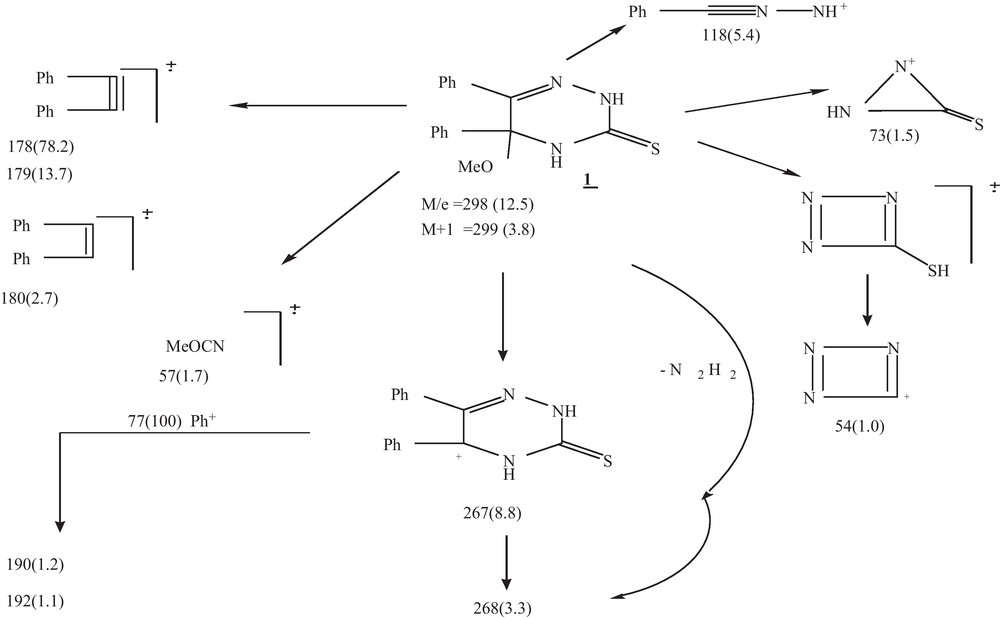
Mass fragmentation of compound 1.
Treating 5-methoxy-5,6–diaryl-4,5-dihydro-2H-1,2,4-triazin -3-thions (1-3) with HgCl2 in methanol at 1:2 molar ratio at room temperature under stirring, produced a type of macro cyclic thioether Schiff base 4 as [5,6,11,12–tetraaryl -1,2,4,7,8,10–hexa-aza cyclododoeca -4,6,10,12–tetraene -3,9-dithione] (Scheme 3).
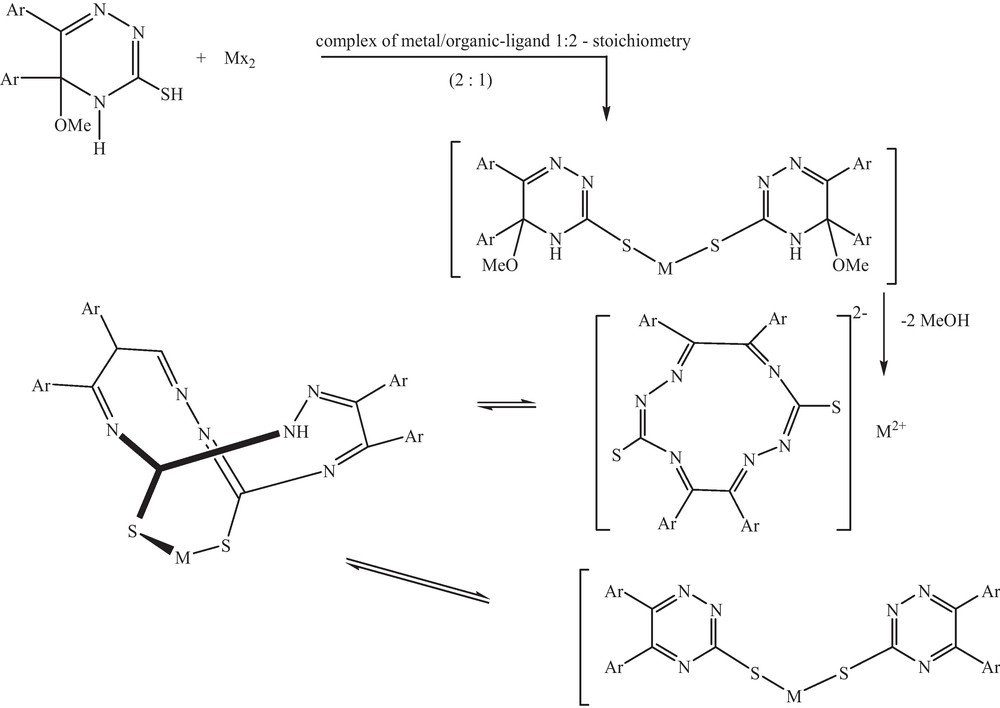
Formation of 4.
Mass spectrum of compound 4 showed two peaks which are assigned to [C34H28N6S2O4]Hg fragment and to the molecular ion of selective ligand C34H28N6S2O4, respectively. IR spectrum of [Hg(ligand)3(Cl) 2] recorded lack of both NH and SH functional groups, suggesting hydrogen bonding between the ligand molecules in the formed complex. 1H NMR spectra of the isolated complex species showed the absence of amine nitrogen's and OMe groups through complexation. 13C NMR spectrum of the formed complex also showed lacks of carbons of OCH3 and two imino carbons confirming the proposed structure.
On treatment compounds 1-3 with HgCl2 in 1:1 molar ratios in methanol at room temperature, compounds 5 a-c were isolated. Formation of these compounds may take place via simple–oxidation through mercuric ions. IR spectrum of compound 5c showed lacks of both NH and SH functional groups as well as M/z recorded M + 2, M + 1 and M+ at 608, 606 and 602 with a base peak at 214 (Scheme 4).
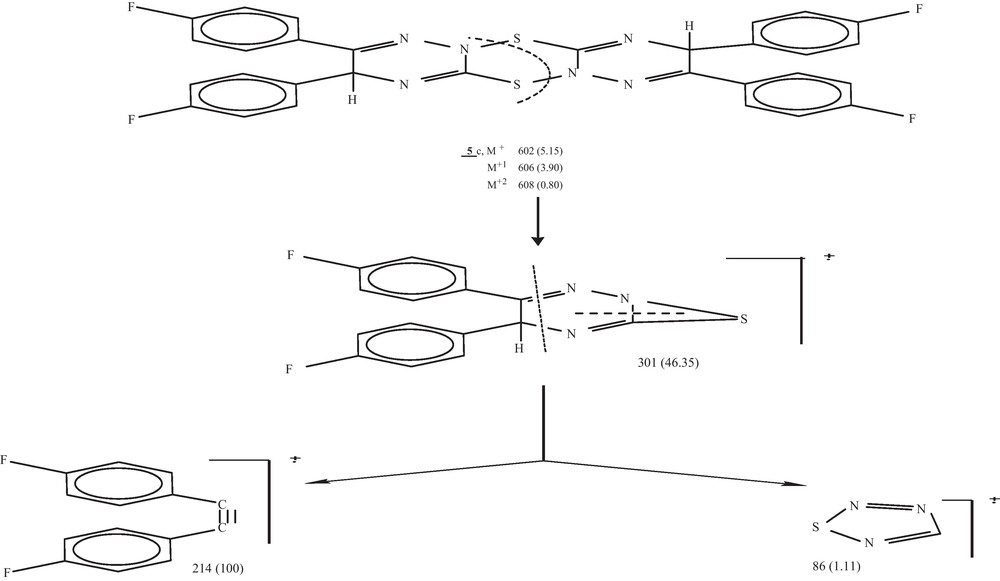
Mass fragmentation pattern of compound 5c.
It is interesting to noting that, on treating compound 3 with HgCl2in 1:1 molar ratio in methanol and stirring at room temperature, bis (5, 6–diaryl-5-methoxy-2,4-dihydro-1,2,4–triazin-3-thiato) mercury was separated out as solid complex 6, while complex 5c was soluble in the filtrate. A possible mechanism of formation of the complex 6 was suggested via addition of metal salts on C3 = N4 of 1,2,4- triazine (Scheme 5), while complex 5c was formed via oxidation of mercapto group followed by elimination of methanol molecules. IR spectrum of compound 6 recorded peaks at ν cm−1 3210, 3165 cm−1 of two NH, in addition at 1507, 1372, 1158 and 1039 cm−1 due to presence of thiocarbamide, -C-OMe functional groups. 1H NMR spectrum in DMSO recorded resonated signals at: δ: 12.5, 10.7, 8.8 and 8.5 ppm afforded to four NH with a signals due to aromatic protons at 7-7.3, 7.5 -7.7 in addition at 3.45 -3.25 ppm of 4-OMe. On the other hand, compounds 1-3 with HgCl2 gave the corresponding complexes of metal- ligand in 1:2 stiochiometry.
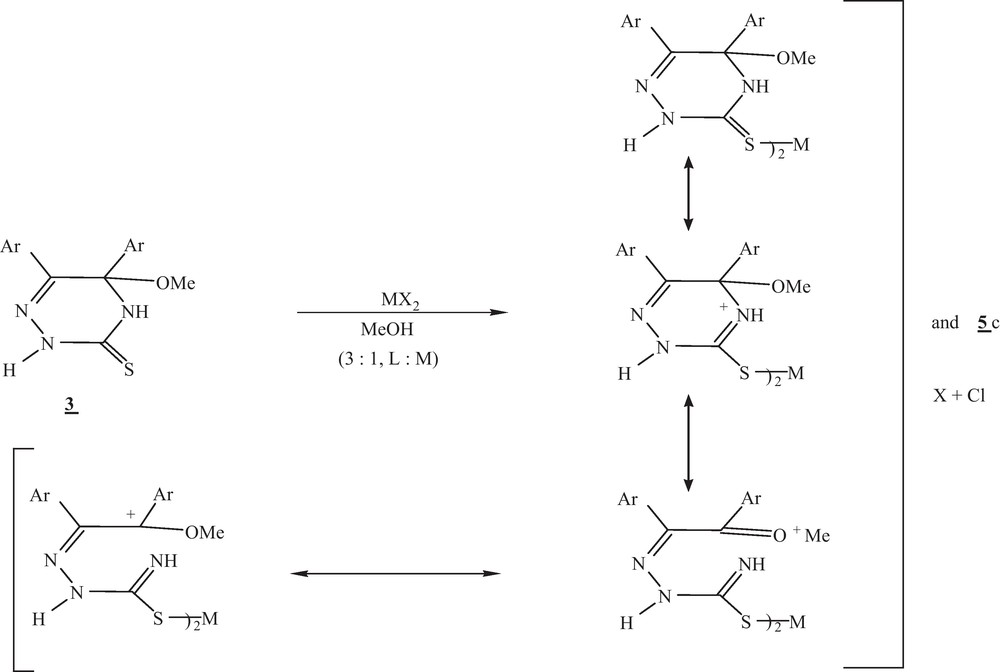
Mechanism of formation of compound 6 from 3.
2.2 Molluscicidal activity
Based upon the earlier work by Ali et al. [23] on the synthesis of phosphono-3- substituted -5, 6-diphenyl -1,2,4- triazine derivatives and their molluscicidal activities against Biomophalaria Alexandrina Snails responsible for Bilharziasis diseases, the prepared compounds were tested as molluscicidal agents against Biomophalaria Alexandrina Snails (shell in diameter 5–8 mm). The intermediate host of Sohistosoma mausoni in Giza Govern state that was not treated with molluscicides. The snails were adapted to laboratory conditions for two weeks before being used in toxicity tests to be sure that the snails are strong and healthy. Snails were kept in plastic aguaria filled with de chlorinated tap water at room temperature (25–27 oC). Stock solutions (500 μg mL−1) of the tested compounds were prepared in the least volume of ethanol and completed to the required volume with de - chlorinated tap water on the basis of weight/volume. A series of more diluted solutions were then prepared following the instructions given by WHO organization [24,25]. The results given in Table 1 revealed high activity towards snails and the following sequences:
The Molluscicidal activity of the prepared systems.
Compound No | Mortality of Snails at various concentrations, ppm | ||
50 | 100 | ||
1 | 20 | 100 | |
2 | 50 | 100 | |
3 | 40 | 100 | |
4a | 40 | 80 | |
4a | 70 | 100 | |
5b | 30 | 100 | |
Reference standard, Baylucide | 100 | 100 |
4a > 5b > 5a and 3 > 2 > 1 were achieved. Total electron barrier of molecular distribution of the evaluated systems led to inhibition of enzymatic effect on the living processes for the tested Snails thereby causing break of a vital-cyclic of snails. The presence of Hg and/or F atoms in system 4 enhanced the toxicity and a deposition of protein in the vital-cell of snails. The fact that Hg2+ ions are most likely able to react with protein containing sulfur forming stable chelate causing deposition of the vital-cell of the snails.
2.3 Voltammetric behavior
Compounds 1 and 4a were selected for cyclic voltammetric studies as representative for organic macromolecule 1 and its mercuric (II) complex. The choice of the two compounds was based upon the influence of mercury (II) on the redox characteristic of the resulting species. The CVs of compound 1 in DMF–tetramethylammonium chloride (TMA+.Cl−) as supporting electrolyte at Pt working electrode vs. Ag/AgCl reference electrode at various scan rates are demonstrated in Fig. 3. Two well-defined anodic peaks at –1.0 and + 0.75 V coupled with two cathodic peaks at –1.25 and –0.75 V were noticed. An ill-defined anodic peak at –0.55 V was also noticed at 100 mV s−1 (Fig. 3) suggesting the irreversible nature of the observed electrode couples. On increasing the scan rates from 100 to 1000 mV s−1, the cathodic and anodic peaks were shifted cathodically and anodically, respectively (Fig. 4). These results confirmed the irreversible nature of the electrode couples [26,27]. Continuous scan of the CV significantly decreased the peak current and the signal was hardly discernible from the baseline, indicating passivity of the surface of the Pt working electrode via formation of polymeric oxidation product or fouling of the electrode by the reduction products.
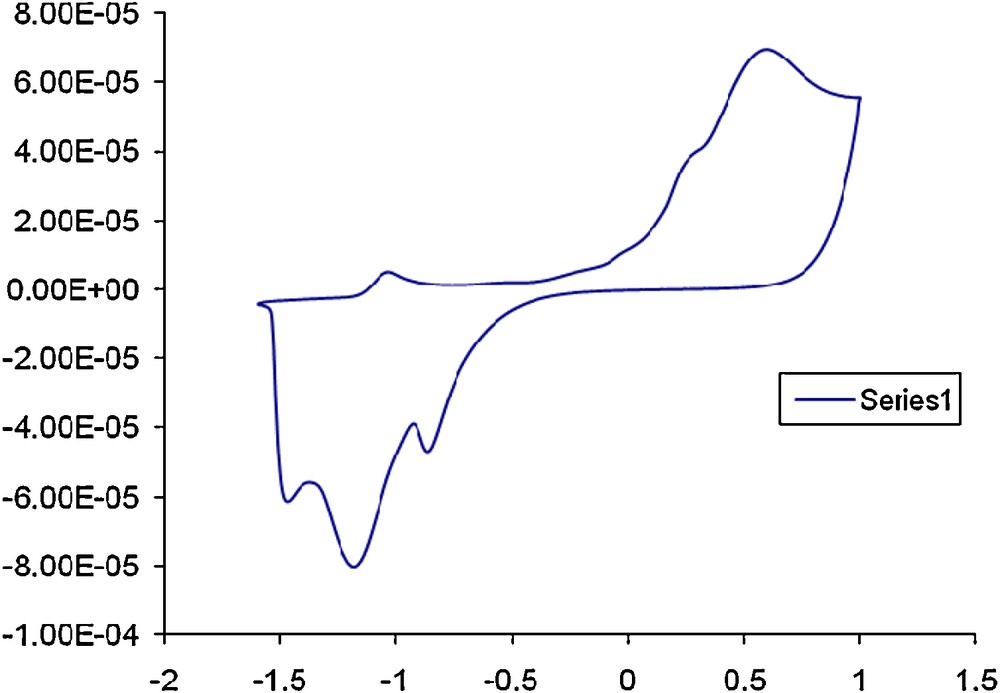
CVs of compound 1 at 100 mV s−1 in the potential window -1.5 to +1. V vs. Ag/AgCl reference electrode.
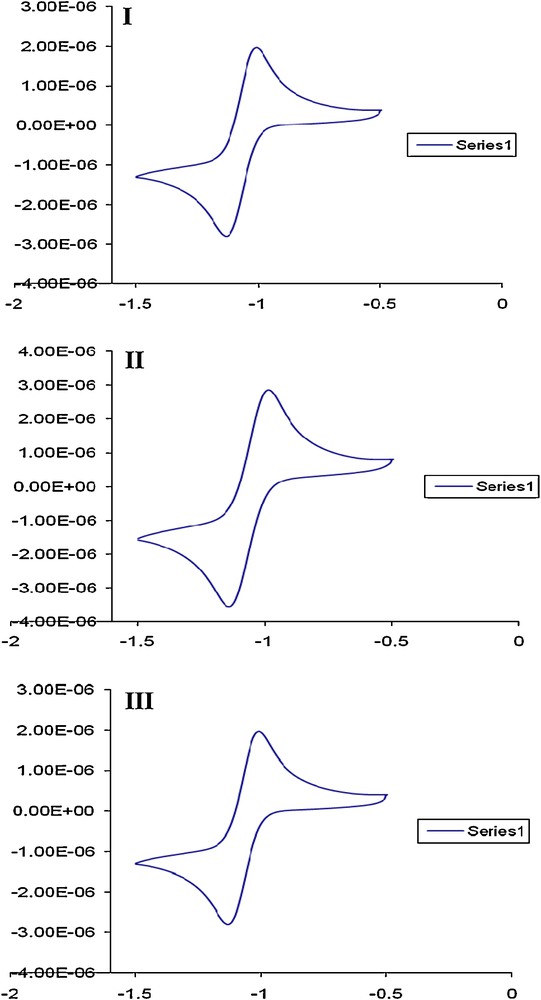
CVs of compound 1 at 50 (I), 100 (II) and 1000 (III) mV/s 500 mV s−1 in the potential window -2 to 0.0. V vs. Ag/AgCl electrode.
The CVs of compound 4 a in DMF-TMA+.Cl− at various scan rates (Fig. 5) showed one well-defined cathodic and anodic peaks in the range −1.2 – 0.8 V with peak–peak potential separation (60 mV) suggesting reversible electrochemical process [26]. The plot of anodic (at –0.91 V) or cathodic (-0.97 V) peak current vs. square root of the scan rate increased linearly on increasing the scan rate, indicating that, the electrochemical reduction process is diffusion controlled electro–chemical process [26,27]. The plot of the cathodic peak potential vs. log scan rate was also linear on raising the scan rate. The current function (ip, c/to) decreased on raising the square root of the scan rate continuously. Thus, the first reduction processes of the compound preceded according to the well-known electrode- coupled chemical reaction mechanism of type EC [26,27]. These results suggested the possible use of compound 1 and its derivatives as complexing agent in stripping voltammetric determination and also in solid phase extraction for separation of arsenic (III, V) ions in industrial water.
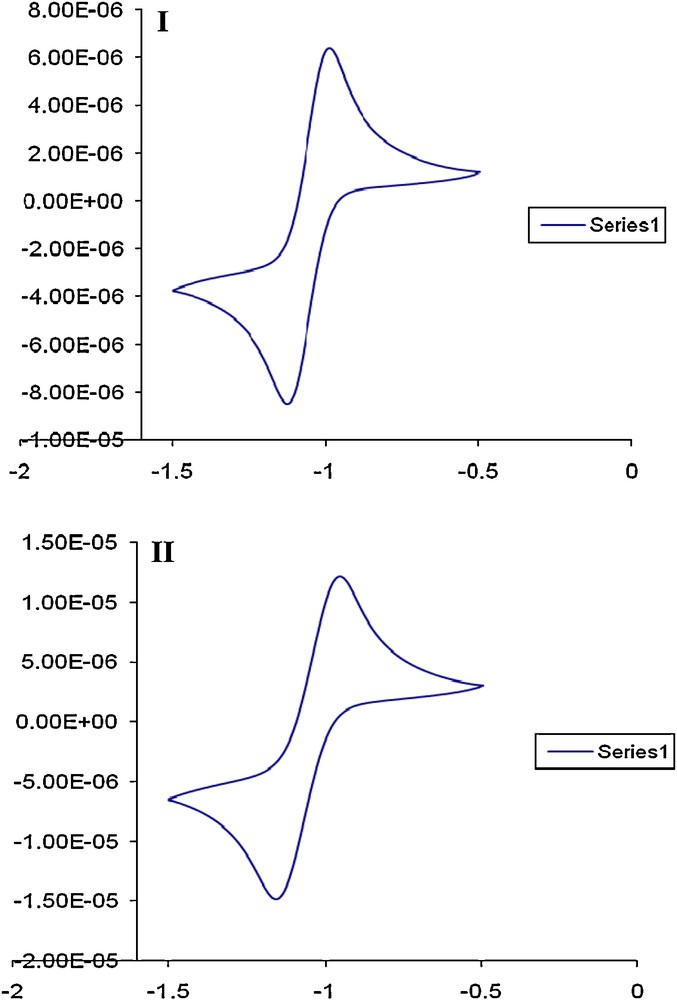
CVs of compound 4a at 50 (I) and 200 (II) mV s−1 V vs. Ag/AgCl electrode.
2.4 Retention profile of arsenic (III) by reagent 1 immobilized polyurethane foams
Polyurethane foams (PUFs) sorbent represent one of the most effective solid sorbent due to its high available surface area, aerodynamic, cellular and membrane structure and extremely low cost [28,29]. A recent literature survey revealed no data on the use of compound 1 or its derivatives as chelating agents for metal separation and/or determination in different matrices. Thus, considerable attention was focused on preconcentration of trace and ultra trace concentrations of arsenic (III) ions in water by compound 1 physically immobilized onto PUFs prior its determination by inductively coupled plasma-optical emission spectrometry (ICP-OES. Preliminary investigation revealed considerable retention of arsenic (III) species onto compound 1 treated PUFs). Therefore, the influence of shaking time, pH and acidity on arsenic (III) uptake by PUFs was investigated. The sorption profile of an aqueous solution of arsenic (ІІІ) at pH 3 by untreated PUFs and compound 1 loaded PUFs at room temperature was studied. Arsenic (ІІІ) in the aqueous phase after equilibrium was determined by ICP-MS. The extraction percentage (%E) and the distribution ratio (D) of arsenic (ІІІ) reached maximum within 90 minutes (Fig. 6). Thus, a shaking time of 90 min was adopted in the subsequent work.
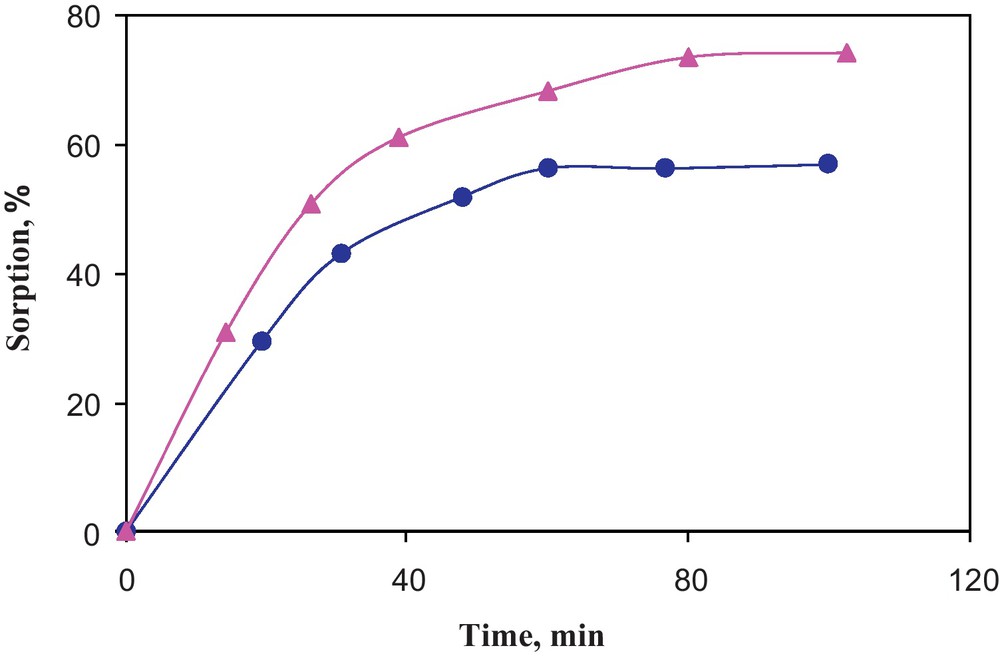
Influence of shaking time on arsenic (III) sorption from aqueous solution onto the unloaded (--) and compound 1 immobilized (--) PUFs from aqueous solutions of pH 3-4.
The sorption profile of arsenic (III) ions at 10.0 μg mL−1 concentration level from the aqueous solutions (50 mL) at different pH by the immobilized PUFs (0.2 ± 0.01 g) was investigated after 90 min shaking time. The sorption profile of arsenic (III) by the immobilized PUFs decreased on increasing the solution pH and maximum sorption percentage was achieved at pH 3. At pH > 3, the decrease in the uptake of arsenic (III) by the loaded PUFs is most likely due to the instability or the hydrolysis of the produced complex species formed between arsenic (III) and the reagent immobilized PUFs. The retention of arsenic (ІІІ) in pH 2-3 is most likely attributed to the protonation of the ether (_ CH2_ OH+_ CH2_) and/or urethane linkages group (- NH2+- COO-) available in the PUFs sorbent membrane. Thus, the retention of analyte proceeded via “solvent extraction and/or weak base anion exchange mechanism” [28,29]. The pKa of the protonated ether group (_ CH2_ OH+_ CH2_)foam and amide group (- NH2+- COO-) foam are _ 3 and _ 6, respectively [28,29]. Thus, the uptake of arsenic (III) is easily proceeded via ether group of the PUFs than the protonated amide group of the PUFs.
2.5 Performance of the reagent 1 treated polyurethane foams packed column
The performance of compound 1 loaded PUFs packed column was determined via critical (CC) and breakthrough capacities (BC) [31,32]. The CC was defined as the amount of arsenic (III) that could be retained on the PUFs column at 2 mL min−1 flow rate until the arsenic (III) was first detected in the effluent. Practically, this value equal the actual volume of the effluent collected just before first appearance of arsenic (III) in the effluent minus the free column volume. The calculated values of CC and BC of arsenic (III) ions from the breakthrough (S–shaped) curve (Fig. 7) were found equal 0.55 and 0.88 mg g −1 to be at a flow rate of 2 mL min−1 [28,29].
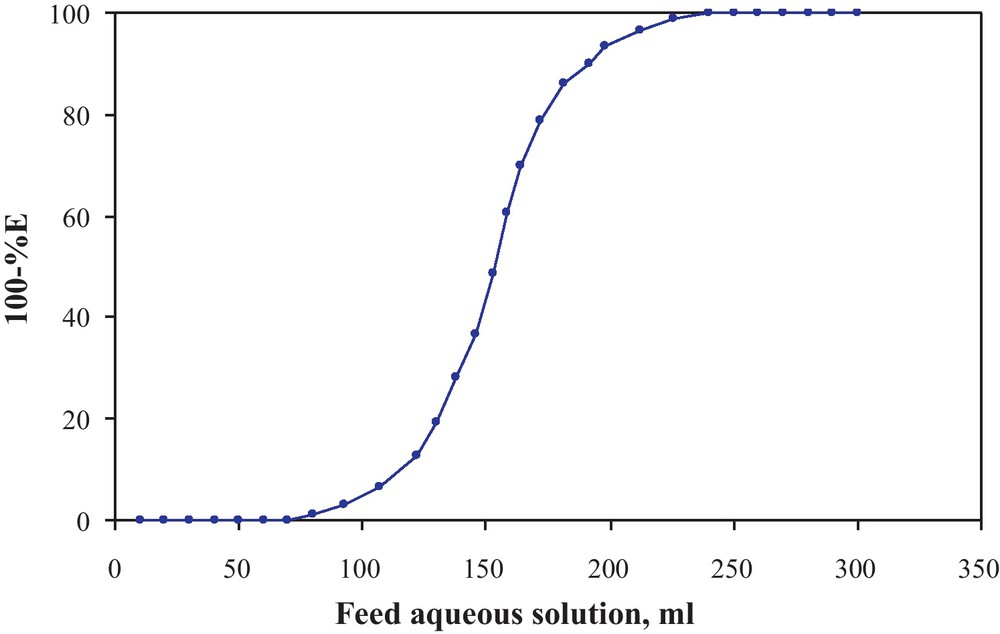
Breakthrough curve of aresnic (III) uptake onto compound 1 loaded PUFs (1.0 ± 0.01 g) packed columns at 5.0 mL min.−1.
The reagent PUFs packed column was tested for retention and recovery of arsenic (III) from wastewater samples (1.0 L). The sample was spiked with arsenic (III) (5–10 μg) and percolated through PUF column at 2.0 mL min−1 flow rate. Complete recovery of the retained arsenic (III) species was achieved by percolating acetone (10 mL) at 2.0 mL min−1 flow rate as indicated from the ICP-OES analysis of arsenic before and after extraction. Arsenic (V) can also be preconcentrated by the developed treated PUFs packed column after reduction with SO2 gas to arsenic (III) in water.
3 Experimental
3.1 Apparatus
The following apparatuses were afforded by the Chemistry Department, Faculty of Sciences, King Abdulaziz University. A Perkin Elmer (Lambda EZ-210) double beam spectrophotometer (190–1100 nm) with 1 cm (path width) quartz cell was used for recording the electronic spectra of the compounds. A Perkins Elmer model RXI-FT-IR system 55529 was used for recording the IR spectra of the compounds. A Brucker advance DPX 400 MHz model using TMS as an internal standard was used for recording the 1HNMR and 13C NMR spectra (Chemical shift in ppm) of the compounds on deuterated DMSO. A GC-MS-QP 1000-Ex model was used for recording the mass spectra of the compounds. Melting points were determined with an electro thermal Bibbly Stuart Scientific Melting Point SMPI (US). Molecular weights and C, H, N analysis of the compounds was performed on Micro analytical center, Cairo University, Egypt. Cyclic voltammetric measurements were carried out on a Metrohm 757 VA trace analyzer and 747 VA stand (Basel, Switzerland). A digital-micro-pipette 10–100 μL (Volac) was used for transferring sample solutions to the electro-chemical cell. The data were recorded at room temperature and the peak current heights were measured using the “tangent fit method”.
3.2 Reagents and materials
Analytical chemicals and reagents grade quality were purchased from BDH chemicals. Low density polyethylene (LDPE) bottles, Nalgene were used for the collection of the different water samples. LDPE bottles and electrochemical cell were carefully cleaned first with hot detergent, soaked in 50% HCl (Analar), HNOBB3 BB(2.0 mol lPP−1PP), subsequently washed with dilute HCl (0.5 mol lPP−1PP) and finally rinsed with distilled water. The sample solution was stored in LDPE bottles and stored at -20 °C. Stock solutions (0.1%w/v) of the reagent were prepared in the appropriate solvent. A stock BDH stock solution of metal ions to be analyzed (1.0 mg/mL) in dilute nitric acid was used. Commercial white sheets of open cell PUFs based polyether type were used as solid sorbent for the separation of the tested metal ions. The foam cubes were then washed with acetone in a Soxhlet extractor for 6 hours, and dried at 80 °C in an oven for 2 hours. The dried foam cubes were then stored in dark low density polyethylene bottles.
3.3 Organic preparation
3.3.1 5- Methoxy -5,6-diaryl -4,5-dihydro-2 H-1, 2, 4-triazine -3-thiones (1-3)
A solution of thiosemicarbazide (12.50 mmol) in dry methanol (150 mL) was added drop wise to a solution of benzil and 4, 4–di(methoxy/fluoro) benzil (12.5 mmol) in absolute methanol with concentrated HCl (25 mL, 2 mol L−1) with constant stirring for 8 hours. The solution was left to cool overnight. The formed precipitates were filtered off, washed and dried to give 1-3, respectively.
Compound 1 was crystallized from ethanol as yellow crystals. Yield = 75%, m.p. 190 oC. Analytical data: Found: C = 64.39, H = 4.99, N = 13.98, S = 10.53%; Calculated for C16H15N3SO: C = 64.64, H = 5.05, N = 14.14, S = 10. 77%; m/z 298 (C16H15N3SO +1, 35%) IR: ν cm−1 = 3184, 3131 (NH), 1608 (C = N), 1550, 846 (thioamide I & II). 1H NMR (DMSO): δ: 12.0 (1 H, s, NH), 10.0 (1 H, s, NH), 7.5 (2 H, m, 5- H), 7.7 -7.2 (8 H, m, diphenyl), 3.1 (3 H, s, OCH3).
Compound 2 was crystallized from ethanol as yellow crystals. Yield (65.2%), m.p. 183 -185 oC. Analytical data: Found: C = 59.49, H= 5.85, N = 11.74, S = 8.97%; Calculated for C18H19N3SO3: C = 60.14, H = 5.35, N = 11.74, S = 8. 97%; m/z 359 (C18H19N3SO3 +2, 15%) IR: ν cm−1 = 3210, 3190 (NH), 1610(C = N), 1560, 860 (thioamide I & II), 1050 (-C-O-Me). 1H NMR (DMSO): δ: 12.3, 10.8 (each 1 H,s, NH), 7.8 -7.3 (8 H, m, aryl protons),3.55 (6 H,s, two OCH3), 3.35 (3H,s, – OCH3), 13C NMR 29.35 – 30.39, 55.74 – 56.25, 106.94, 115, 125.91 – 133.09, 146.72, 160.39, 166.01, 180.37 and 194.48 and 197.64.
Compound 3 was crystallized from ethanol as faint yellow crystals. Yield (82%), m.p. 225-226 0C. Analytical data: Found: C = 57.25, H = 3.85, N = 12.46, S = 9.49; F = 11.27%. Calculated for C16H13N3SF2O: C =57.61, H = 3.95, N = 12.94, S = 9. 67; F = 11.41 %; m/z 334. (C16H13N3SF2O +1, 26%) IR: ν cm−1 = 3250 – 2850 (b, NH, aromatic & aliphatic CH), 1610 (C = N), 1485 (deformation CH3), 1530, 880 (thioamide I, II), 1200 (C = S), 1060 (-C-O-Me), 680 (C-F). 1H NMR (DMSO): δ: 11.2, 10.8 (each 1 H,s, NH), 7.8 – 7.2 (8 H, m, aryl protons), 3.35 (3H,s, – OCH3), 13C NMR 29.58,31.09, 36.23, 40.85, 162.86 and 206.45.
3.3.2 5,6,11,12–Tetraaryl -1,2,4,7,8, 10 hexaaza–cyclododeca -4, 6,10,12–tetraene -3,9-dithiones (4)
A solution of mercuric chloride (0–70 mmol) in methanol (50 mL) was added to a solution of compound 2 (1.4 mmol) in absolute methanol (50 mL). The reaction mixture was stirred for 4 h at room temperature. The solid precipitate was filtered off, washed with methanol and dried to give 4. Dilution of the filtrate yielded the cyclic thioether 5. The solution was filtered and crystallized from methanol. This compound was crystallized from methanol as yellow crystals. Yield (45%), m.p. 110 oC. Analytical data: Found: C = 47.52, H = 3.26, N = 9.78, S = 7.45%, Calculated for [C34H28N6S2O4]Hg,: C = 48.5, H = 3.29, N = 9.95, S =7.53%; IR: ν cm−1 lacks of both NH, SH; 1H NMR (DMSO): δ: 3.15, 10.8 (4 s, OCH3), 7.07, 7.1,7.2, 7.3, 7.5, 7.6, 7.7 and 7.8 (each s, 8 H, 8 H aryl protons).
3.3.3 Cyclic thioether 5a
A solution of mercuric chloride (0–70 mmol) in methanol (50 mL) was added to a solution of compound 2 (0–70 mmol) in absolute methanol (50 mL). The reaction was stirred for 4 hours at room temperature and the solid precipitate was filtered off, washed with methanol and dried.
Compound 5a was crystallized from ethanol as pale yellow crystals. Yield (35%), m.p. 130 Analytical data: Found: C = 62.29, H = 4.56, N = 12.79, S = 9.49%. Calculated, for (C34H30N6S2O4) C = 62.76, H = 4.61, N = 12.92, S =9.84%. IR: ν cm−1 lacks of both NH, SH with peaks at 1620 (C = N), 1180 (C-S), 1055 (C-O-Me); 1H NMR (DMSO): δ: 8.8 (2 H, s< H-C5 of 1,2,4–triazine), 7.7 -7.0 (16 H, m, aryl protons), 3.65 -3.55 (12 H, s, 4-OCH3).
3.3.4 Cyclic thioether, 5b
Compound 5b was prepared as mentioned using compound 1 and mercuric chloride salt in 1: 1 molar ratio and crystallized from ethanol as yellow crystals. Yield (80%), m.p. 120. Analytical data: Found: C = 67.37, H = 4.10, N = 15.67, S = 11.94%. Calculated for C30H22N6S2: C = 67.9, H = 4.15, N = 15.84, S = 12.07%. IR: ν cm−1 2704 (N-S-N), 1525, 1329 (cyclic amide I, II), 1024 (C-S-N), 867, 807 (phenyl CH), 1H NMR (DMSO): δ: 7.3 -7.8 (22 H, m, phenyl & H–C5 of 1,2,4–triazine), 13C NMR 167 (CS), 156, 1, 156.0 (C-N), 131.31 and 128.84 (carbon - phenyls).
3.3.5 Bis [5,6- di (4-flurophenyl)-5-methoxy-2,4-dihydro-1,2,4- triazin-3-thiato] – mercury, 6 and cyclic thioether, 5c
A methanolic solution of mercuric chloride (0.01 mmol) in methanol (50 mL) was added to a solution of compound 3 (0.03 mmol) in absolute methanol (50 mL). The reaction mixture was stirred for 8 hours at room temperature. The solid precipitate was filtered off and dried to give a type of complex 6. On diluting the filtrate, a solid compound was separated out, filtered off, washed with methanol and dried to give 5c.
Compound 5c was crystallized from THF as pale yellow crystals. Yield (45%), m.p. 280 oC. Analytical data: Found: C = 59.50, H = 2.90, N = 13.70, S = 10.35, F = 12.45%. Calculated for C30H18N6S2F4, C = 59.80, H = 2.99, N = 13.95, S = 10.63, F =12.62%. M/z 602 (M+, 5.15), 606 (M + 1, 3.901, 608) (M + 2, 0.80), 301 (46.35), 214 (100) and 86 (1.11).
Compound 6 was crystallized from ethanol as yellow crystals. Yield (40%), m.p. 190 Analytical data: Found: C = 40.90, H = 2.84, N = 8.18, S = 6.09, F = 8.31%, M/z = 938. Calculated for C32H26N6S2F4O2HgCl2, C = 40.93, H = 2.87, N = 8.95, S = 6.82, F = 8.39%. IR: ν cm−1 3210, 3165 (NH, NH), 1507, 1372 (thiocarbamide), 1156 (C = S) and 1039 (C-O-Me); 1H NMR (DMSO): δ: 12.5 (1H, s, NH), 10.7 (1 H,s, NH), 8.8 & 8.5 (1 H, each s, NH), 7.7–7.5 (4 H, m, aryl), 7.3 – 7.0 (4 H, m, aryl) and 3.45 (6 H, each s, O- CH3); 13C NMR 29.35, 30.39 (O- CH3); 116.45,116.71 (C-N), 131 -134 (aryl carbons), 206.19 (C = S).
3.4 Analytical applications
3.4.1 General cyclic voltammetry
The general procedure for recording cyclic voltammetric at differential sweep rates was carried out as follows. An accurate concentration (10 mmol) of the test compound and supporting electrolyte tetra methyl ammonium chloride (100 mmol) in DMF were transferred into the voltammetric cell. The solution was stirred with nitrogen for 15 minutes to release oxygen. The voltammograms were then recorded in the potential ranges e -1.5 to 1.V at Pt working electrode versus Ag/AgCl reference electrode.
3.4.2 Preparation of polyurethane foam packed column
Compound 1 was physically immobilized onto PUFs as follows: PUFs cubes (1.0 cm3) were shaken well with compound 1 for 10 minutes as reported [28].An accurate weight (1.0 ±0.01 g) of the reagent 1 immobilized PUFs was packed in a column (1000 × 10 id mm) using the vacuum method of foam packing [28,29]. The aqueous solutions (0.1 L) containing arsenic (III) at concentrations < 10 μg mL−1 were percolated through the PUFs packed column at 5 mL min−1 flow rate after adjustment the solution to pH = 2–3 with B-R buffer. The sample and blank PUFs packed columns were then washed with 100 mL of B-R buffer solution at the same pH. Quantitative retention of arsenic (III) took place on the immobilized PUFs as indicated from the ICP-AES measurement of arsenic in the effluent solutions. The retained arsenic (III) species were then recovered quantitatively from the foam column with acetone 910 mL at 5 mL min−1 flow rate.
Acknowledgement
The authors would like to thank the Deanship of Scientific Research, King Abdul Aziz University, Kingdom of Saudi Arabia for the financial support under the grant number MX/11/20 and the Chemistry Department for the facilities provided.