1 Introduction
Since the first report by Fieser et al. [1] on the N-formylation of amines, a number of strategies have been developed for their synthesis. N-formyl compounds have been widely used in the synthesis of pharmaceutically valuable compounds such as fluroquinlones [2], aryl imidazoles [3], 1,2-dihydro quinolines [4], nitrogen-bridged hetrocycles [5], oxazolidinones [6] and cancer chemotherapeutic agents [7]. They are also important precursors in the synthesis of fungicides and herbicides [3]. In addition, fomamides are Lewis bases, and act as catalysts in the allylation [8], hydrosilylation [9], Vilsmeier reactions [10] and for the synthesis of formamidines [11]. Various organic, inorganic, homogeneous, and heterogeneous catalysts/reagents have been employed for this purpose. The recent efforts highlighted the importance of N-formylation reactions [12]. However, there is always need for better methodology for this type of widely used reaction. Surprisingly, to the best of our knowledge, no N-formylation reactions utilizing β-lactams bearing amino groups as substrates have been reported to date.
The 2-azetidinone ring (β-lactam) as a synthetic intermediate has been widely recognized in organic synthesis. It is an active moiety present in most widely used antibiotics, such as penicillins [13], cephalosporins [14], carbapenems [15], nocardicins [16] and monobactams [17]. Moreover, it showed many other interesting biological properties, such as cholesterol absorption inhibitors [18], human cytomegalovirus protease inhibitors [19], thrombin inhibitors [20], anti-hyperglycemic [21], anti-tumour [22], anti-HIV [23], anti-inflammatory, analgesic activities [24] and serine-dependent enzyme inhibitors [25]. Hence, it is suspected that formylation of amino groups in new synthetic β-lactams potentially have some unique bioactivities.
Due to the high surface area, volume ratios, nanoparticles can effectively improve the loading and catalytic efficiency of immobilized catalysts. Therefore, the use of nano-structured materials as supports for different versions of catalyst immobilization is ever increasing.
2 Results and discussions
Recently, our laboratory has been engaged mainly on the development of modified metal oxide nano structures for environmental organic synthesis [26]. Nano-sulfated TiO2, was prepared by sol-gel method. Analysis of the SEM and TEM images of this catalyst reveals that particles are uniformly distributed in spherical shape. Hence, SO4−2 ion modification preferably retarded the aggregation. This facilitates the organic transformations. It is found from XRD and TEM that the catalyst is in nanoparticles (40–50 nm) and the anatase and rutile phases of TiO2 has been confirmed and sulfated modification does not change the phases. The surface area of nano-sulfated TiO2 was determined using the nitrogen gas adsorption method, which is the most important factor influencing the catalytic activity. BET surface area of nano-sulfated TiO2 is 218 m2/g. These results are shown in Table 1.
Characterization data for nano-sulfated TiO2 prepared by sol-gel method.
XRD | 2θ = 25 with crystallite size ∼40 nm |
FT-IR | 1221, 1138 cm−1 due to asymmetric and symmetric stretching of S = O |
1047 cm−1 related to asymmetric S-O bond | |
993 cm−1 related to symmetric stretching of S-O bond | |
1626, 3347 cm−1 related to -OH group | |
666 cm−1 related to Ti-O and Ti-O-Ti | |
mmol SO4/g [TGA] | ∼5.8% |
BET surface area | 218 m2/g |
Pore volume | 0.40 mL.g−1 |
Acidity | H0 value = 1.916 |
As a part of the ongoing research program on the catalytic applications of nano-sulfated titania in organic transformations, herein we report for the first time, an alternative method for the N-formylation of β-lactams bearing amino groups using nano-sulfated titania as catalyst. The catalyst used is readily and easily accessible and neither inert atmosphere nor solvents is required for the reaction to proceed. Moreover, the reaction work up and purification of the products are straightforward and provide the corresponding N-formylated amino β-lactams in excellent yields at room temperature.
We have previously demonstrated that ZnO is an efficient catalyst for the N-formylation of amines under solvent-free conditions at 70 °C [12e]. Unfortunately, ZnO was not successful at N-formylation of β-lactams even at room or high temperatures (Scheme 1). However, there was an assumption that nano-sulfated titania might be efficient for N-formylation of β-lactams under mild conditions and without the need for any reaction additives or solvents.
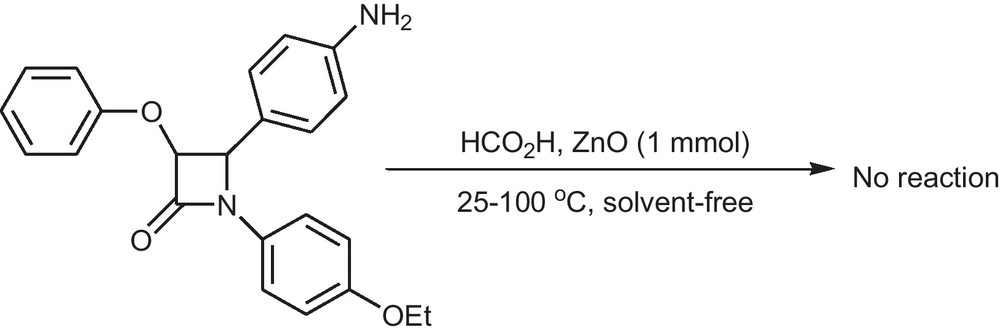
During the course of other studies in our laboratory, a new series of β-lactams was synthesized and then treated with formic acid as formylating agent by adding nano-sulfated titania as catalyst under solvent-free conditions at room temperature. After different optimization reactions, it was observed that the best conditions are: nano-sulfated TiO2 (0.015 mol%, 0.2 g), formic acid (85%) (1 mmol) and β-lactams (1 mmol). To investigate the scope of this reaction, a variety of amino β-lactams were screened. The results of these studies are depicted in Scheme 2 and summarized in Table 2.
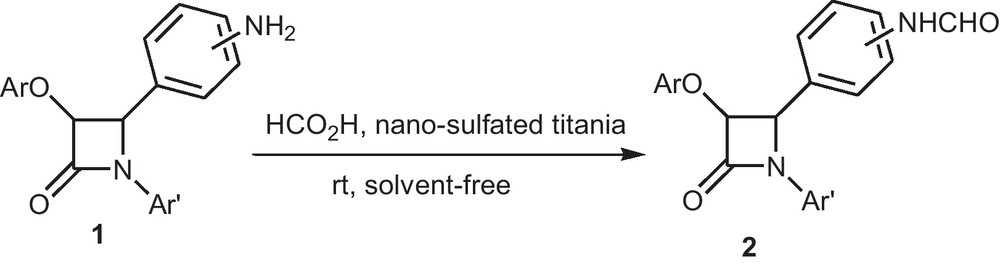
Substrate scope for N-formylation catalyzed by nano-sulfated TiO2 under solvent free condition.
Entry | Substrate | Product | Time (h) | Yielda (%) |
1 | 1a | 2a | 3 | 95 |
2 | 1b | 2b | 3.5 | 75 |
3 | 1c | 2c | 3 | 80 |
4 | 1d | 2d | 3.5 | 95 |
5 | 1e | 2e | 3.5 | 83 |
6 | 1f | 2f | 3 | 95 |
7 | 1 g | 2 g | 4 | 60 |
8 | 1 h | 2 h | 4.5 | 95 |
a Isolated yield.
All the compounds were synthesized as stable colorless powders and they were recrystallized from EtOH and no side-products were obtained. Their structures were confirmed by spectroscopic methods and elemental analysis. In the 1H NMR spectra of compounds 2a-2 h, the signals for the H-3 and H-4 protons of the β-lactam ring are well resolved. The assignment of the NMR signals of the formylated β-lactams indicated the stability of the four-membered ring in this reaction condition.
With a general strategy for the N-formylation of amino β-lactams established, we were interested in applying it to the N-formylation of amines. Recently, Krishnakumar et al. [27] reported a useful method for N-formylation of anilines using sulfated titania; however, the success of this method is limited to aromatic primary amines, and the method did not avoid the use of organic solvents. Herein, we have been motivated to undertake the challenge to carry out N-formylation reaction applicable to all types of amines (aliphatic, alicyclic, aromatic, primary and secondary) at room temperature under solvent-free conditions by using nano-sulfated titania. The differences between our procedure and the previously reported methods could be explained in terms of the size of the catalyst, surface area and the amount of sulfuric acid functionalized on titania. Sulfated titania which was used in the present study is found to have large surface area (218 m2/g) and the size of particles is 40–50 nanometers. Therefore, encouraged by remarkable results obtained with the formylation of β-lactams, and in order to show the generality and scope of this new catalyst, we used various aromatic and aliphatic amines for N-formylation reaction (Scheme 3 and Table 3).

N-formylation of different amines catalyzed by nano-sulfated TiO2 under solvent-free condition.
Entry | Substrate | Product | Time (h) | Yielda (%) |
1 | 0.67 | 90 | ||
2 | 0.50 | 90 | ||
3 | 2.00 | 50 | ||
4 | 0.50 | 80 | ||
5 | 0.50 | 90 | ||
6 | 0.50 | 90 | ||
7 | 0.50 | 90 | ||
8 | 0.50 | 90 | ||
9 | 0.75 | 90 | ||
10 | 1.50 | 90 | ||
11 | 0.50 | 90 | ||
12 | 1.00 | 90 | ||
13 | 1.00 | 80 |
a Isolated yield.
According to Table 3, most types of primary and secondary amines (aliphatic, aromatic and heterocyclic) find an easy route to their N-formyl derivatives in the presence of formic acid and nano-sulfated titania at room temperature under solvent-free conditions. The open chain aliphatic amines reacted smoothly under such reaction conditions; even benzylamine that was previously reported to provide low yields [12f,k] (42 and 85%) furnished an excellent yield (90%) following this reaction protocol. Anilines bearing both electron-donating and electron-withdrawing functionalities were also found to undergo the conversion in a facile manner with excellent yields. Previously, the N-formylation of anilines having electron withdrawing groups was found to be difficult [12a]. The workup of the reaction mixtures is very simple and convenient, and in many cases the products are obtained with high purities.
Nano-sulfated TiO2 is found to be acidic (Pka = 1.911) [26a,b] and it is effective in N-formylation of amines. Hence, this reaction follows the mechanism of acid-catalyzed condensation reactions. A similar mechanism has been previously proposed [27,28].
Recycling of the nano-sulfated TiO2 catalyst was then examined. For this, the reaction of aniline with formic acid in the presence of nano-sulfated TiO2 was studied. When the reaction was completed, the catalyst was easily separated from the product by adding ethyl acetate and the soluble catalyst was centrifuged, and dried under vacuum and reused in a subsequent reaction. The recycled catalyst could be used five or six times without any loss in its catalytic effect (Table 4). XRD patterns, SEM, and FT-IR imagines of solid nano-sulfated TiO2 after using in the reaction are shown in Figs. 1 and 2. Clearly, the nano-sulfated TiO2 structure of the catalyst remains intact. Also the used catalyst was characterized by TG, whereas, according to the TG analysis, ∼99% of the catalyst was recovered after at most six times used of the catalyst in the synthesis of organic compounds. Thus, the catalyst is stable in the reaction condition.
Reuse ability of catalyst on N-formylation of aniline (1 mmol) at room temperature under solvent-free conditions.
Run nano-sulfated TiO2 | 1 | 2 | 3 | 4 | 5 | 6 |
Isolated yield (%) | 90 | 90 | 90 | 88 | 87 | 87 |

a: XRD patterns; b: SEM imagines of nano-sulphated TiO2 after reaction.
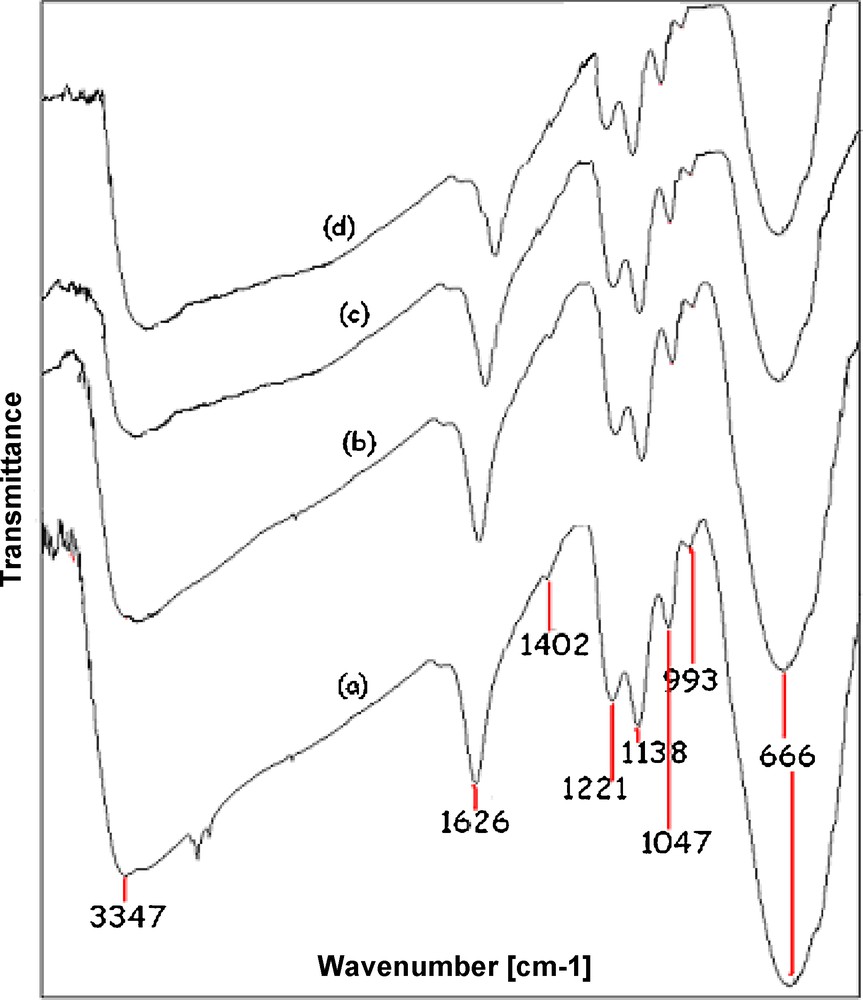
FT-IR spectra of the (a) nano-sulphated titania (b) recovered after first (c) third and (d) fifth reuse.
FT-IR spectroscopy of fresh nano-sulfated titania is shown in Fig. 2a. Four bands in the region between 1230 and 980 cm−1 attributed to vibrational modes of bidentate sulfate ions can be seen. Two bands at 1221 and 1138 cm−1 appear due to asymmetric and symmetric stretching of S = O vibrations, respectively. The band around 1047 cm−1 is attributed to the asymmetric S-O bond. The band at 993 cm−1 is related to the symmetric stretching of the S-O bond. All of these bands are related to the sulfate bounds to the TiO2 in the chelate form. The bands at 1626 and 3347 cm−1 are, respectively, associated with the bending and stretching vibrations of the OH group of water molecules on the surface of the solid [29]. The FTIR spectrum of the catalyst recovered after first, third, and fifth reaction showed two clear bands for the bending and stretching vibrations of the -OH group of water molecules which was shown that the water molecule can be adsorb during the work-up procedure. Also, after fifth recovered catalyst are shown the bands in the range of 990–1221 cm−1 which indicate the sulfonyl group.
In conclusion, the N-formylation of different kinds of amines and β-lactams bearing the amino group using nano-sulfated TiO2 as a catalyst was investigated in the present study. The catalyst was obtained by sol-gel method and characterized in terms of its acidity, and structural aspects. The advantages of this protocol are as follows: a) the use of a cheap and easily prepared catalyst, b) solvent free conditions, c) short reaction times and high yields, d) easily reaction procedure and workup, e) use of low amount of catalyst (0.015 mol%), and f) both components (amines and formic acid) are taken in stoichiometric amounts in order to avoid environmental waste, fulfilling the philosophy of green chemistry.
3 Experimental part
All needed chemicals were purchased from Merck, Fluka and Acros chemical companies. IR spectra were run on a Shimadzu FT-IR 8300 spectrophotometer. 1H NMR and 13C NMR spectra were recorded in DMSO-d6 using a Bruker Advance DPX instrument (1H NMR 250 MHz, 13C NMR 62.9 MHz). Chemical shifts were reported in parts per million (δ) downfield from TMS. All of the coupling constants (J) are in hertz. The mass spectra were recorded on a Shimadzu GC–MS QP 1000 EX instrument. Elemental analyses were run on a Thermo Finnigan Flash EA-1112 series. Melting points were determined in open capillaries with Buchi 510 melting point apparatus. Thin-layer chromatography was carried out on silica gel F254 analytical sheets obtained from Fluka. Column chromatography was performed on Merck Kiesel gel (230–270 mesh).
3.1 Typical procedure for the synthesis of 2a-2 h
To a mixture of nano ST (0.2 g, 0.015 mmol) and formic acid (1 mmol), free amino β-lactam or amine (1 mmol) was added. The reaction mixture was stirred for a certain period of time (Tables 1 and 2) as required to complete the reaction (monitored by TLC) at room temperature. The reaction mixture was diluted with EtOAc and centrifuged to remove the catalyst. Then the organic layer was washed with aqueous solution of sodium bicarbonate and dried over anhydrous sodium sulfate. Evaporation of solvent furnished practically pure products. The identity of these compounds was easily established by comparison of their 1H NMR spectra with those of authentic samples.
3.1.1 N-(4-(1-(4-Ethoxyphenyl)-4-oxo-3-phenoxy-azetidin-2-yl)phenyl)formamide (2a)
White powder purified by column chromatography (eluent: 5:1 CHCl3-EtOAc) (yield 95%, 0.38 g); Mp: 148–152 °C; IR (KBr, cm−1): 1749 (CO β-lactam), 1661 (CO Amidic), 3339 (NH); 1H NMR (250 MHz, DMSO-d6) δ 1.26 (3H, t, J 7.5 Hz, -CH3), 3.93 (2H, q, J 7.5 Hz, -OCH2), 5.61 (1H, d, J 5.0 Hz, H-4), 5.70 (1H, d, J 5.0 Hz, H-3), 6.78–6.89 (5H, m, Ar-H), 7.01–7.13 (4H, m, Ar-H), 7.19 (2H, d, J 8.9 Hz, Ar-H), 7.45 (2H, d, J 7.1 Hz, Ar-H), 8.16 (1H, s, CHO), 10.05 (1H, s, NH); 13C NMR (62.9 MHz, DMSO-d6) δ 14.5 (Me), 60.7 (OCH2), 63.1 (C-4), 80.5 (C-3), 115.0, 117.0, 118.4, 118.8, 121.6, 128.1, 128.6, 129.2, 129.9, 138.3, 155.2, 156.4 (aromatic carbon), 159.3 (CO-Amidic), 162.1 (CO β-lactam); GC-MS m/z(%) = 402 (1.7) [M+], 388 (4.7), 295 (3.2), 267 (1.8), 239 (100.0), 211 (19.5), 134 (14.2), 106 (23.0), 77 (11.2); analysis calculated for C24H22N2O4: C, 71.63; H, 5.51; N, 6.96. Found: C, 71.59; H, 5.49; N, 6.96%.
3.1.2 N-(4-(3-(2,4-Dichlorophenoxy)-1-(4-methoxyphenyl)-4-oxoazetidin-2-yl)phenyl)formamide (2b)
White crystal purified by thin layer chromatography (eluent: 4:1 CHCl3-EtOAc) (yield 75%, 0.31 g); Mp: 120–123 °C; IR (KBr, cm−1): 1679 (CO Amidic), 1740 (CO β-lactam), 3358 (NH); 1H NMR (250 MHz, DMSO-d6) δ 3.67 (3H, s, OMe), 5.64 (1H, d, J 5.0 Hz, H-4), 5.84 (1H, d, J 5.0 Hz, H-3), 6.82 (2H, d, J 10.3 Hz, Ar-H), 6.91 (2H, d, J 7.5 Hz, Ar-H), 7.06 (2H, d, J 9.0 Hz, Ar-H), 7.16–7.25 (4H, m, Ar-H), 7.49–7.54 (2H, m, Ar-H), 8.17 (1H, s, CHO), 10.09 (1H, s, -NH); 13C NMR (62.9 MHz, DMSO-d6) δ 55.2 (OMe), 60.6 (C-4), 80.5 (C-3), 114.5, 116.8, 118.2, 118.4, 119.1, 123.5, 125.5, 128.7, 129.0, 129.9, 133.8, 138.1, 155.2, 156.0, 159.5, 161.6 (aromatic carbon), 162.3 (CO β-lactam); GC-MS m/z (%) = 422 (10.1) [M+], 258 (2.9), 169 (4.1), 139 (4.5), 111 (14.8), 83 (29.7), 57 (100.0); analysis calculated for C23H19ClN2O4: C, 65.33; H, 4.53; N, 6.62. Found: C, 60.61; H, 4.44; N, 6.48%.
3.1.3 N-(4-(3-(4-Chlorophenoxy)-1-(4-ethoxyphenyl)-4-oxoazetidin-2-yl)phenyl)formamide (2c)
White crystal purified by thin layer chromatography (eluent: 4:1 CHCl3-EtOAc) (yield 80%, 0.34 g); Mp: 158–160 °C; IR (KBr, cm−1): 1665 (CO Amidi), 1749 (CO β-lactam), 3302 (NH); 1H NMR (250 MHz, DMSO-d6) δ 1.02 (3H, t, J 5.0 Hz, -CH3), 3.92 (2H, q, J 6.8 Hz, -OCH2), 5.60 (1H, d, J 5.0 Hz, H-4), 5.80 (1H, d, J 5.0 Hz, H-3), 6.81–6.89 (4H, m, Ar-H), 7.07 (2H, d, J 7.5 Hz, Ar-H), 7.18–7.22 (2H, m, Ar-H), 7.30 (2H, d, J 7.5 Hz, Ar-H), 7.60 (2H, d, J 8.4 Hz, Ar-H), 8.26 (1H, s, CHO), 10.15 (1H, s, NH); 13C NMR (62.9 MHz, DMSO-d6) δ 14.5 (CH3), 60.4 (C-4), 63.1 (OCH2), 80.5 (C-3), 115.0, 116.7, 117.0, 118.5, 118.8, 125,5, 128.7, 129.1, 129.8, 138.4, 155.2, 155.2, 159.5, (aromatic carbon), 161.6 (CO Amidic), 162.3 (CO β-lactam); GC-MS m/z (%) = 436 (1.9) [M+], 368 (1.3), 273 (28.0), 245 (100.0), 211 (19.9), 135 (14.4), 106 (31.7); analysis calculated for C24H21ClN2O4: C, 67.56; H, 5.16; N, 6.85. Found: C, 66.98; H, 5.10; N, 6.91%.
3.1.4 N-(4-(1-(4-Ethoxyphenyl)-3-(naphthalene-2-yloxy)-4-oxoazetidin-2-yl)phenyl)formamide (2d)
White crystal purified by thin layer chromatography (eluent: 4:1 CHCl3-EtOAc) (yield 71%, 0.32 g); Mp: 160–162 °C; IR (KBr, cm−1): 1679 (CO Amidic), 1740 (CO β-lactam), 3339 (NH); 1H NMR (250 MHz, DMSO-d6) δ 1.26 (3H, t, J 6.9 Hz, -CH3), 3.93 (2H, m, -OCH2), 5.70 (1H, d, J 5.0 Hz, H-4), 5.94 (1H, d, J 5.0 Hz, H-3), 6.89 (2H, d, J 8.9 Hz, Ar-H), 6.95-7.04 (1H, m, Ar-H), 7.23 (2H, d, J 8.9 Hz, Ar-H), 7.30–7.47 (7H, m, Ar-H), 7.69 (1H, d, J 9.0 Hz, Ar-H), 7.75–7.79 (2H, m, Ar-H); 13C NMR (62.9 MHz, DMSO-d6) δ 14.5 (Me), 60.6 (OCH2), 63.2 (C-4), 80.5 (C-3), 108.6, 115.0, 116.9, 117.8, 118.5, 118.7, 124.1, 126.5, 127.5, 128.7, 128.8, 129.3, 130.0, 133.6, 138.1, 154.1, 155.2 (aromatic carbon), 159.5 (CO amidic), 161.9 (CO β-lactam); GC-MS m/z (%) = 452 (14.4), [M+], 308 (12.5), 289 (100.0), 268 (32.1), 239 (19.5), 144 (28.7), 106 (13.8), 83 (20.2), 57 (45.5); analysis calculated for C28H24N2O4: C, 74.32; H, 5.35; N, 6.19. Found: C, 71.98; H, 5.21; N, 6.48%.
3.1.5 N-(3-(1-(4-Methoxyphenyl)-3-(naphthalene-2-yloxy)-4-oxoazetidin-2-yl)phenyl)formamide (2e)
White crystal purified by thin layer chromatography (eluent: 4:1 CHCl3-EtOAc) (yield 83%, 0.36 g); Mp: 152–154 °C; IR (KBr, cm−1): 1689 (CO Amidic), 1751 (CO β-lactam), 3278 (NH); 1H NMR (250 MHz, DMSO-d6) δ 3.68 (3H, s, -OMe), 5.75 (1H, d, J 4.7 Hz, H-4), 5.97 (1H, d, J 4.7 Hz, H-3), 6.91 (2H, d, J 9.0 Hz, Ar-H), 7.14–7.44 (10H, m, Ar-H), 7.62–7.77 (3H, m, Ar-H), 8.15 (1H, s, CHO), 10.14 (1H, s, NH); 13C NMR(62.9 MHz, DMSO-d6) δ (ppm); 60.5 (OMe), 66.6 (C-4), 85.8 (C-3), 114.2, 117.2, 119.3, 119.7, 121.2, 123.2, 123.7, 129.3, 131.7, 132.0, 132.7, 133.8, 134.1, 134.5, 135.5, 138.9, 153.6, 159.7, 161.1 (aromatic carbon), 162.2 (CO Amidic), 167.3 (CO β-lactam); GC-MS m/z (%) = 438 (43.6) [M+], 410 (12.7), 368 (17.1), 289 (54.8), 254 (86.9), 226 (30.6), 144 (28.5), 111 (18.5), 83 (45.2), 57 (100.0); analysis calculated for C27H22N2O4: C, 73.96; H, 5.06; N, 6.39. Found: C, 71.96; H, 5.20; N, 6.73%.
3.1.6 N-(3-(1-(4-Methoxyphenyl)-4-oxo-3-phenoxy-azetidin-2-yl)phenyl)formamide (2f)
White crystal purified by thin layer chromatography (eluent: 4:1 CHCl3-EtOAc) (yield 95%, 0.36 g); Mp: 160–163°C; IR (KBr, cm−1): 1684 (CO Amidic), 1749 (CO β-lactam), 3339 (NH); 1H NMR (250 MHz, DMSO-d6) δ 3.67 (3H, s, -OMe), 5.65 (1H, d, J 5.0 Hz, H-4), 5.83 (1H, d, J 5.0 Hz, H-3), 6.80 (2H, d, J 7.8 Hz, Ar-H), 6.91 (3H, d, J 9.0 Hz, Ar-H), 7.14–7.25 (6H, m, Ar-H), 7.49–7.55 (2H, m, Ar-H), 8.16 (1H, s, CHO), 10.16 (1H, s, NH); 13C NMR (62.9 MHz, DMSO-d6) δ 55.2 (OMe), 61.3 (C-4), 80.6 (C-3), 112.8, 114.0, 114.5, 115.3, 116.0, 118.3, 121.7, 128.6, 129.3, 130.3, 133.7, 148.4, 155.8, 156.4 (aromatic carbon), 159.48 (CO Amidic), 162.08 (CO β-lactam); GC-MS m/z (%) = 388 (7.3) [M+], 368 (7.8), 295 (5.4), 255 (10.1), 239 (100.0), 211 (16.5), 134 (13.4), 106 (15.8), 83 (30.8), 57 (65.5); analysis calculated for C23H20N2O4: C, 71.12; H, 5.19; N, 7.21. Found: C, 70.95; H, 5.06; N, 7.15%.
3.1.7 N-(4-(1-(4-Methoxyphenyl)-4-oxo-3-phenoxy-azetidin-2-yl)phenyl)formamide (2g)
White crystal purified by thin layer chromatography (eluent: 4:1 CHCl3-EtOAc) (yield 60%, 0.23 g); Mp: 160–163 °C; IR (KBr, cm−1): 1689 (CO Amidic), 1743 (CO β-lactam), 3348 (NH); 1H NMR (250 MHz, DMSO-d6) δ 3.75 (3H, s, -OMe,), 5.70 (1H, d, J 5.0 Hz, H-4), 5.87 (1H, d, J 5.0 Hz, H-3), 6.88 (2H, d, J 8.2 Hz, ArH), 6.97 (2H, d, J 8.4 Hz, Ar-H), 7.13–7.31 (5H, m, Ar-H), 7.38 (2H, d, J 8.3 Hz, Ar-H), 7.53 (2H, d, J 8.0 Hz, Ar-H), 8.26 (1H, s, CHO), 10.22 (1H, s, NH); 13C NMR (62.9 MHz, DMSO-d6) δ 55.0 (OMe), 60.7 (C-4), 80.5 (C-3), 114.1, 115.0, 117.0, 118.4, 118.8, 121.6, 128.5, 129.0, 130.0, 138.0, 155.8, 156.4 (aromatic carbon), 159.1 (CO Amidic), 161.9 (CO β-lactam); GC-MS m/z (%) = 388 (17.5) [M+], 255 (12.2), 239 (45.4), 210 (17.0), 165 (11.2), 149 (17.5), 111 (20.6), 83 (44.5), 57 (100.0); analysis calculated for C23H20N2O4: C, 71.12; H, 5.19; N, 7.21. Found: C, 70.95; H, 5.07; N, 7.17%.
3.1.8 N-(4-(3-(2,4-Dichlorophenoxy)-1-(4-methoxy-phenyl)-4-oxoazetidiyl)phenyl)formamide (2h)
White crystal purified by thin layer chromatography (eluent: 4:1 CHCl3-EtOAc) (yield 95%, 0.43 g); Mp: 155–158 °C; IR (KBr, cm−1): 1651 (CO Amidic), 1735 (CO β-lactam), 3237 (NH); 1H NMR (250 MHz, DMSO-d6) δ 3.68 (3H, s, -OMe), 5.66 (1H, d, J 5.0 Hz, H-4), 5.96 (1H, d, J 5.0 Hz, H-3), 6.87 (2H, d, J 8.9 Hz, Ar-H), 7.00 (1H, d, J 7.5 Hz, Ar-H), 7.14 (2H, d, J 8.6 Hz, Ar-H), 7.18–7.21 (4H, m, Ar-H), 7.30–7.32 (1H, m, Ar-H), 7.40 (1H, d, J 8.1 Hz, Ar-H); 13C NMR (62.9 MHz, DMSO-d6) δ 60.3 (OMe), 66.0 (C-4), 85.8 (C-3), 117.9, 119.2, 119.6, 120.9, 121.4, 123.6, 127.4, 130.8, 132.8, 133.7, 134.4, 135.2, 138.1, 153.6, 156.1, 161.1 (aromatic carbon), 162.2 (CO-Amidic), 166.3 (CO β-lactam); GC-MS m/z (%) = 456 (32.0) [M+], 307 (35.9), 272 (27.4), 254 (57.6), 209 (19.6), 180 (20.3), 149 (100.0), 117 (24.7), 91 (41.5), 57 (32.9); analysis calculated for C23H18Cl2N2O4: C, 60.41; H, 3.97 N, 6.13 Found: C, 60.25; H, 4.10; N, 6.20%.
Acknowledgments
We gratefully acknowledge the support of this work by the Shiraz University Research Council.