1 Introduction
Rhodanine heterocyclic skeletons are known to possess multiple biological activities, including the inhibition of numerous targets, such as PMT1 manosyl transferase [1], PRL-3 and JSP-1 phosphatases [2]. Classical methods for the preparation of alkylidine rhodanine need several steps and generally involve the preparation of the rhodanine moiety followed by a Knoevenagel condensation with aldehydes [3]. Recent methods are limited by the requirement of highly reactive functional groups in the substrates and by the fact that they do not proceed in smooth and mild conditions. Several methods have been reported for the synthesis of rhodanines [3]. However, they all rely on multistep reactions with either hard conditions or low yields, and the reaction times are long. Thus, the development of new synthetic methods remains an attractive goal.
Organic processes catalyzed by non-toxic magnetic nanoparticles (MNPs), such as Fe3O4 and Fe2O3, are often considered as following the principles of green chemistry, i.e. they consume a minimum of energy and reagents or auxiliaries, and minimize wastes [4]. Magnetic separation of MNPs is simple, economical and promising for industrial applications. From both economic and environmental viewpoints, organic reactions in aqueous medium using recoverable catalysts have gained attention in recent years [5].
For the above reasons, we were, thus, intrigued by the possibility of applying nano- and green chemistry to the design of an active, recyclable, and magnetically recoverable dispersed nano-γ-Fe2O3 catalyst in EtOAc-medium for the synthesis of alkyl rhodanines under mild reaction conditions.
2 Results and discussion
Recently, we have developed synthetic methods for the synthesis of biologically interesting heterocycles via multicomponent reactions [6]. The challenge in this field was to develop efficient and rapid green methods. Moreover, the synthesis of these heterocycles has usually been carried out in polar solvents, such as DMF and DMSO, leading to complex isolation and recovery procedures. Also, based on our recent success in the preparation of MNPs as catalysts [6], combined with our achievments in this area, we present, in this paper, the results of an extended investigation on the activity of dispersed γ-Fe2O3 in EtOAc-medium as a green magnetic separable nanocatalyst in a domino three-component process leading to the formation of alkyl rhodanines without any salts or additives. We have developed a synthetic method for the preparation of 5-oxo-2-thioxo-3-thiophenecarboxylate via the condensation of an aliphatic amine, carbon disulfide and the electron-deficient acetylene using a dispersed tecnique of small size (∼30 nm range, Fig. 1) of γ-Fe2O3 as the active catalyst. Herein, the condensation of benzyl amine, carbon disulfide and dimethyl acetylenedicarboxylate (DMAD) was selected to be the model (Table 1). Also, the MNP to form γ-Fe2O3 was synthesized by basic precipitation from the iron source [8].

Transmission electron microscopy (TEM) image of the γ-Fe2O3 nanoparticles.
Optimization of reaction conditions for the synthesis of rhodanine 3aa.
Entry | Fe-source | Solvent | Cat. (mol%) | T (°C) | Time (min) | Yield (%) |
1 | FeCl3·6H2O | THF | 5 | 40 | 5 | 24 |
2 | FeSO4 | CCl4 | 5 | r.t. | 5 | 17 |
3 | γ-Fe2O3/SiO2·MNPsb | CCl4 | 5 | 50 | 5 | 26 |
4 | Bulk-Fe3O4 | CCl4 | 5 | r.t. | 5 | 20 |
5 | Nano-Fe3O4 | CCl4 | 5 | r.t. | 5 | 31 |
6 | γ-Fe2O3·MNPs | CCl4 | 4 | r.t. | 5 | 37 |
7 | γ-Fe2O3·MNPs | H2O | 5 | r.t. | 5 | 43 |
8 | γ-Fe2O3 | EtOAc | 5 | r.t. | 5 | 61 |
9 | γ-Fe2O3 | EtOAc | 5 | r.t. | 10 | 75 |
10 | γ-Fe2O3 | EtOAc | 10 | r.t. | 5 | 73 |
11 | γ-Fe2O3 | EtOAc | 10 | r.t. | 10 | 95 |
12 | γ-Fe2O3 | EtOAc | 15 | r.t. | 10 | 94 |
a Reaction scale: 3 mmol.
b Synthesized based on our previous report [6i].
Initial studies of the influence of the reaction conditions were carried out with the model reaction. By screening a wide range of iron sources, mol% of catalyst and different solvents and temperatures, we found that the product 3a could be obtained in yields ranging from 17 to 95% (entry 1–12, Table 1). When we have changed the amount of the catalysts from 5 mol% to 15 mol%, finding out that 10 mol% was enough for the best yield.
With these results in hand and to demonstrate the diversity of the dispersed MNPs (DMNPs) of γ-Fe2O3 as a nanocatalyst and to expand the scope of the process, the optimized conditions were applied to a series of substrates 1 and 2, as shown in Table 2.
The synthesis of the 5-oxo-2-thioxo-3-thiophenecarboxylate skeleton by γ-Fe2O3a.
3 | R | R′′ | R′ | Yield% 3 | Mp (°C) | |
Reported | Found | |||||
3a | C6H5 | CH3 | H | 95 | 128–130 [6e] | 131–133 |
3b | C6H5 | CH3 | CH3 | 95 | 89–91 [6e] | 87–90 |
3c | o-Cl–C6H4 | CH3 | H | 93 | 112–114 [6e] | 113–115 |
3d | o-Cl–C6H4 | CH3CH2 | H | 90 | 104–106 [6e] | 105–109 |
3e | p-Cl–C6H4 | CH3 | H | 90 | – | 114–117 |
3f | iPr | CH3 | H | 90 | 88–90 | 85–90 |
3g | CH3 | CH3 | CH3 | 95 | – | 60–66 |
3h | Vinyl | CH3 | H | 85 | 140–142 [6e] | 139–144 |
a Reaction scale: 3 mmol.
The catalytic activity of γ-Fe2O3 in the domino three-component process for synthesizing rhodanine 3a and 4 was also studied under optimized conditions. When we set the reaction with a mixture of an aromatic amine (aniline) and an aliphatic amine (benzyl amine) in the presence of carbon disulfide and dimethyl acetylenedicarboxylate (DMAD), the yield of 3a was 89% after 15 min, without any detected product 4 (Scheme 1).
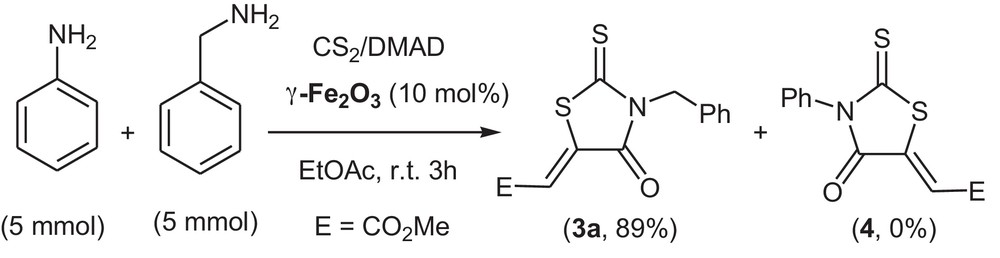
Chemoselective synthesis of alkyl rhodanines.
The possibility of recycling the dispersed magnetic catalyst was also studied. When the reaction was completed, the catalyst was easily separated from the product by attaching an external magnet onto the reaction vessel. As illustrated in Table 3, the catalyst could be still reused after the sixth run.
Recyclability study on the synthesis of model compound 3a.
Run | 1 | 2 | 3 | 4 | 5 | 6a |
Yield% 3a | 95 | 93 | 91 | 87 | 88 | 73 |
a Time of the reaction was 2 h.
Although we have not established the exact mechanism for our method in an experimental manner, the following rationalization may be advanced to explain the product formation [6e,7]. Presumably, the γ-Fe3O4 stabilized zwitterion 5 from amine and carbon disulfide was attached by active acetylene to furnish a cumulene 6 or dithiocarbamate 7 intermediate. The final product 3a is produced by γ-Fe3O4 accelerated methanol elimination and cyclization of 6 or 7 (Scheme 2).
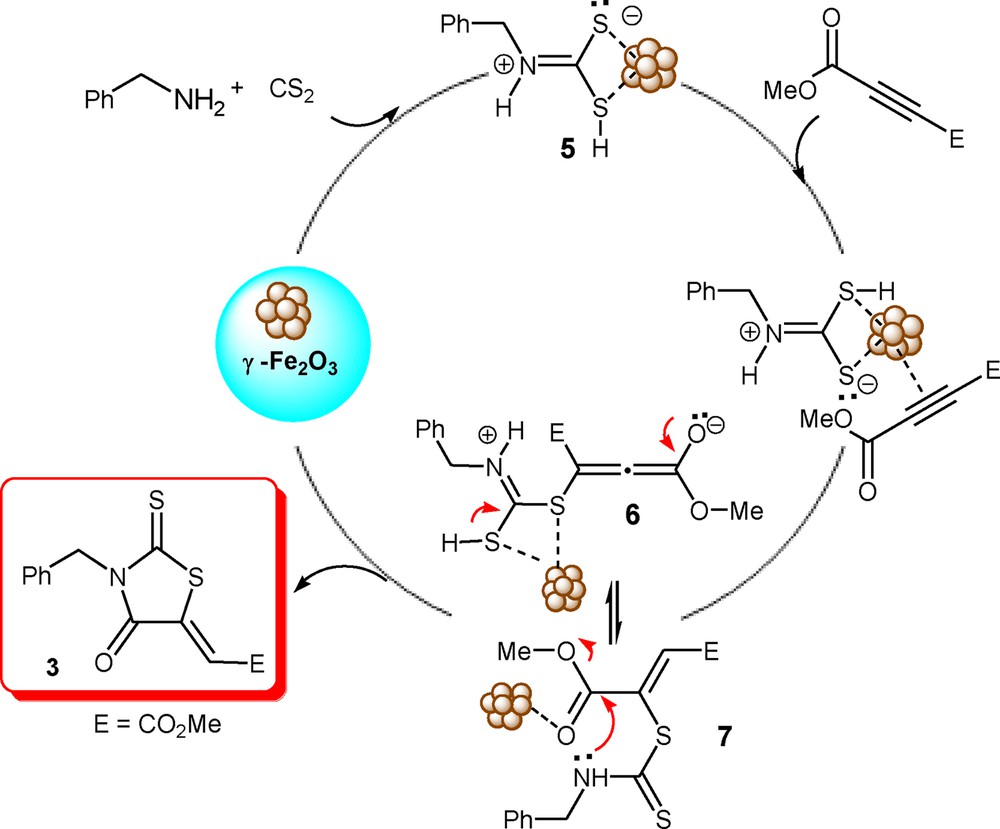
Plausible mechanism for the magnetic nanoparticles-catalyzed synthesis of rhodanine.
3 Conclusion
In summary, an extensive and systematic study allowed us to identify MNPs of γ-Fe2O3 as a heterogeneous, green and reusable catalyst for the synthesis of rhodanines via a domino process. This rapid, green method, in which the catalyst can be recycled, should be an interesting alternative to other synthesis methods. The method has several advantages, including high yields of products, recyclability of the catalyst, non-toxicity, easy experimental work-up procedure, and the fact that the catalyst is environmentally friendly.
4 Experimental
All the reagents were obtained from Merck (Germany) and Fluka (Switzerland) and were used without further purification. IR spectra were recorded on a Shimadzu IR- 460 spectrometer. The melting points were measured on an Electrothermal 9100 apparatus. The progress of reactions was monitored by thin layer chromatography (TLC).
4.1 Synthesis magnetic nanoparticles of γ-Fe2O3 (MNPs)
Maghemite γ-Fe2O3 nanoparticles were synthesized based on an interesting method reported by Kolvari, Khazaei and Zolfigol [8], with minor modifications. After adding a Fe-source and a reductive reagent, and after the color of the solution turned back from red to light yellow, the solution was added to an ammonia solution under stirring; to achieve uniform particle size and stability of MNPs, oleic acid was added.
4.2 General procedure for the preparation of compound 3a–h: preparation of 3a
Benzyl amine (3 mmol) and carbon disulfide (3.3 mmol) were added to a dispersion of MNPs (10 mol%) in ethyl acetate (3 mL), and the mixture was stirred at room temperature for 30 s; then 3 mmol of DMAD were added to the mixture during 30 s. The reaction progress was monitored by TLC. Finally, the catalyst was extracted under a magnetic external field, and reaction products were diluted in water, then the organic phase was extracted and the pure product was recrystallized. All isolated products gave satisfactory spectral and physical data. All the products were previously reported [6e] and were characterized by comparison with IR and physical data. Spectral data for the selected product: Ethyl 2-[3-((4-chlorophenyl)methyl)-4-oxo-2-thioxo-1,3-thiazolan-5-yliden]ethanoate (3e) Orange powder. IR (KBr) (νmax, cm−1): 1728 (CO), 1649 (CC), 1310 and 1177 (CS). 1H NMR (500 MHz, CDCl3): δH = 3.87 (3 H, s, OCH3), 5.24 (2 H, s, CH2Ph), 6.84 (1 H, s, CCH), 7.28 (2 H, d, 3JHH = 8.4 Hz, 2 CH of Ar), 7.37 (2 H, d, 3JHH = 8.4 Hz, 2 CH of Ar) ppm. 13C NMR (125.7 MHz, CDCl3): δC = 46.62 (OCH3), 52.89 (CH2Ph), 117.16 (CCH), 128. 86 (2 CH of Ar), 130.49 (2 CH of Ar), 132.79 (Cipso of Cl), 134.36 (Cipso of NC), 141.82 (CCH), 165.45 (CON), 166.46 (CO2CH3), 195.43 (CS) [6e,7].