1 Introduction
Organic syntheses involving greener process under solvent-free conditions have received significant attention in the previous years due to the simplification of experimental procedures, work-up techniques and saving labor to bring down pollution as well as handling costs [1]. Xanthenes and benzoxanthenes are important intermediates in organic synthesis due to their wide range of biological and pharmaceutical properties, such as antiviral [2], antibacterial [3], and anti-inflammatory [4] activities. The other useful applications of these heterocycles include their utilization as dyes [5], as fluorescent materials for the visualization of biomolecules [6] and in laser technologies [7]. In view of the great importance of xanthenes in pharmaceutical and industrial chemistry, although various methods have been reported for the synthesis of xanthenes and benzoxanthenes [8], there still remains a need for the development of new and simple synthetic methods for the efficient preparation of new xanthene derivatives.
Bisphenols have attracted great interest because of their importance in organic chemistry. Bisphenol-A, for example, is a key monomer in the production of epoxy resins and in the most common form of polycarbonate plastic, which is used to make a variety of common products, including water bottles, sports equipment, medical and dental devices, CDs and DVDs and eyeglass lenses [9].
Bisphenols are often prepared by the condensation reaction of phenols and aldehydes or ketones in the presence of various protonic or Lewis acids, such as boron trifluoride [10], polyphosphoric acid [11], dry hydrochloric acid [12], acetic acid [13], trifluoro-methanesulfonic acid (TfOH) [14], cetyltrimethyl-ammonium chloride [15], metal cation-exchanged montmorillonites [16], heteropolyacid (HPA) and supported HPA [17]. Although these methods are suitable for the synthesis of bisphenols, many of them suffer from one or more drawbacks, such as long reaction times, low yield, use of toxic solvents, requirement of an excess of reagents/catalysts, hash reaction conditions, tedious work-up and multistep purifications. The search for milder and more environmentally benign conditions is, therefore, highly demanding for the synthesis of these compounds.
Considering the attention given to the condensation of hydroxyaromatic compounds with aldehydes, it is rather surprising that the products from such reactions involving the use of xylenol derivatives have not been described in the literature. This general type of reaction has provided a convenient route to a variety of complex substances that are accessible only with difficulty with other methods.
2 Results and discussion
pTSA is a low-cost solid catalyst, which has been recently used as a substitute for conventional acidic catalytic materials [18]. A literature survey revealed that several articles have been published about the condensation of phenols and aldehydes in the presence of pTSA [19,20a]. In view of these reports and also due to our recent studies directed toward the development of practical, safe and environmentally friendly procedures for some important transformations [20], we are reporting herein the reaction of aldehydes and xylenols for the formation of 9H-xanthene and bisphenol derivatives in the presence of a catalytic amount of pTSA under solvent-free conditions (Schemes 1 and 2). To the best of our knowledge, this methodology has not been reported in the literature.

Synthesis of 9H-xanthene derivatives.

Synthesis of bisphenol derivatives.
In order to determine the most appropriate reaction conditions, the condensation reaction of 3,5-xylenol with 4-chlorobenzaldehyde was carried out in different solvents and under solvent-free condition in the presence of pTSA. The results are shown in Table 1. The best results were obtained with 10 mol% of the catalyst under solvent-free conditions at 100 °C and gave the corresponding 9H-xanthene in high yields. pTSA in excess of 0.2 equival. did not improve the yield to a greater extent (Table 1, entry 6). A slight excess of 3,5-xylenol was found to be advantageous, and hence, the molar ratio of 3,5-xylenol to aldehyde was kept at 2.2:1.
Optimization of the reaction conditions.
Entry | Conditions | Time (min) | Yield (%)a |
1 | EtOH, reflux, pTSA (0.1 equiv.) | 180 | 30 |
2 | CH3CN, reflux, pTSA (0.1 equiv.) | 180 | < 20 |
3 | CHCl3, reflux, pTSA (0.1 equiv.) | 180 | 40 |
4 | THF, reflux, pTSA (0.1 equiv.) | 180 | 53 |
5 | Solvent-free, 100 °C, pTSA (0.1 equiv.) | 40 | 90 |
6 | Solvent-free, 100 °C, pTSA (0.2 equiv.) | 40 | 90 |
a Isolated yields.
After optimizing the conditions, next, we examined the generalization of this procedure to other substrates using 3,5-xylenol and different aromatic aldehydes (Table 2). Benzaldehyde and other aromatic aldehydes containing electron-withdrawing and electron-donating groups were employed and reacted to give the corresponding 9H-xanthenes in good to high yields. The presence of an electron-donating (methyl) group on the aromatic ring of the aldehyde (Table 2, entry 4) decreased both the rate and the yield of the reaction. However, when some aliphatic aldehydes, such as propionaldehyde and isobutyraldehyde, were used in this protocol under the above optimized conditions, unfortunately, the expected products could not be obtained.
Synthesis of 9H-xanthene and bisphenols derivatives.
Entry | Aldehyde | Phenol | Product | Time (min) | Yield (%)a | M.P. (°C) |
1 | 50 | 86 | 189–191 | |||
2 | 40 | 90 | 233–234 | |||
3 | 40 | 91 | 215–217 | |||
4 | 60 | 80 | 195–196 | |||
5 | 60 | 78 | 174–175 | |||
6 | 70 | 75 | 189–191 | |||
7 | 45 | 84 | 195–196 | |||
8 | 50 | 81 | 172–73 | |||
9 | 60 | 83 | 170–72 | |||
10 | 60 | 80 | 227–28 | |||
11 | 75 | 77 | 201–02 | |||
12 | 60 | 82 | 219–20 |
a Isolated yields.
Encouraged by the successful condensation of aldehydes and 3,5-xylenol under solvent-free condition to give 9H-xanthenes derivatives, we next attempted the reaction with other xylenols such as 2,4-xylenol, 3,4-xylenol, 2,5-xylenol, and aromatic aldehydes under optimized reaction conditions, which led to the exclusive formation of bisphenol derivatives (Scheme 2). Various aromatic aldehydes, bearing electron-withdrawing groups as well as electron-donating groups, were synthesized under optimized conditions. It was found that the corresponding bisphenol derivatives could also be obtained in good to high yields without any difficulty (Table 2). The obtained results indicated that 2,5-xylenol reacted with aldehydes only at the para positions, due to the lower steric hindrance at this position.
A mechanistic rationale portraying the probable sequence of events is given in Scheme 3. Phenol–aldehyde condensation in the presence of an acid catalyst is believed to proceed via a quinone methide (QM) intermediate [21], which has been used in many tandem processes [22] and [4+2] cycloaddition with a variety of dienophiles [23]. The reaction proceeds through the in situ formation of ortho or para-QM intermediates by nucleophilic addition of xylenol to the aldehyde, which is further attacked by a second molecule of xylenol to give bisphenols or by cyclodehydration to give the xanthene derivatives.

The most probable mechanism for the condensation of xylenols and aldehydes.
Comparing the structures of xylenols and of the corresponding products, it can be seen that in all the cases, xylenols reacted at positions with lower steric hindrance; the fact that 3,5-xylenol produced xanthene derivatives but that 2,4-xylenol and 3,4-xylenol gave bisphenol derivatives can be explained by the stability of the carbocation, resulting from the xylenol attack on the o-QM intermediate (Scheme 4). Each one of these carbocations is a hybrid of four structures: for example, I–IV for 3,5-xylenol. In the cases of structures III–IV, the positive charge is located on the carbon atom to which CH3 is attached. Thus, these carbocations are particularly stable. Because of the contribution of the structures III–IV, the hybrid carbocation resulting from 3,5-xylenol is more stable than the carbocation resulting from 2,4-xylenol and 3,4-xylenol.
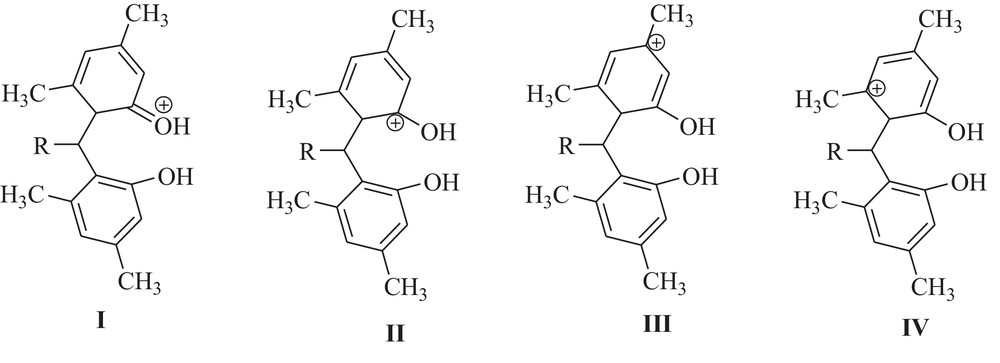
The resonance structures of the carbocation resulting from 3,5-xylenol attack to o-quinone methide intermediate.
3 Conclusion
In summary, a convenient and efficient procedure has been developed for the synthesis of 9H-xanthene and bisphenol derivatives by the condensation of various aromatic aldehydes with xylenols using pTSA as a catalyst. The present approach offers the advantages of clean reaction, simple methodology, short reaction time, high yield, easy purification, and economic availability of the catalyst.
4 Experimental
4.1 General
The melting points were measured by using capillary tubes on an electro thermal digital apparatus and are uncorrected. The progress of reactions was monitored by thin-layer chromatography (TLC) using n-hexane/EtOAc as the eluent. IR spectra were recorded on a KBr disc with a Mattson Galaxy Series FTIR 5000 spectrometer. NMR spectra were recorded with a Bruker spectrometer (300 MHz) in DMSO-d6 or CDCl3 with TMS as an internal standard. Elemental analyses were performed by Vario EL equipment at Arak University.
4.2 General procedure for the preparation of 9H-xanthene derivatives
To a mixture of aldehyde (1 mmol) and 3,5-xylenol (2.2 mmol), pTSA (0.1 mmol) was added. The reaction mixture was stirred magnetically at 100 °C for the appropriate time, as shown in Table 2. The progress of the reaction was monitored by TLC. After the completion of the reaction, the reaction mixture was cooled to room temperature, and a mixture of EtOH–H2O (5:1) was added to it. The suspension was stirred for 10 min and the precipitate was filtered. The crude products were purified by recrystallization from a mixture of EtOH–H2O (5:1).
4.2.1 1,3,6,8-tetramethyl-9-phenyl-9H-xanthene (Table 2, entry 1)
IR (KBr): νmax = 3022, 2964, 2918, 1631, 1616, 1564, 1454, 1330, 1294, 1219, 1147, 1055, 841, 752, 702 cm−1; 1H NMR (300 MHz, CDCl3): δ = 7.21 (d, J = 7.8 Hz, 2H), 7.12 (t, J = 7.4 Hz, 2H), 7.02 (t, J = 7.4 Hz, 1H), 6.80 (s, 2H), 6.61 (s, 2H), 5.08 (s, 1H), 2.25 (s, 6H), 2.22 (s, 6H); 13C NMR(75 MHz, CDCl3): δ = 151.9, 144.6, 137.4, 136.6, 128.6, 128.5, 126.3, 126.1, 122.3, 115.2, 40.1, 21.1, 19.3; Anal calcd for C23H22O: C, 87.86; H, 7.05. Found: C, 87.95; H, 7.15.
4.2.2 9-(4-chlorophenyl)-1,3,6,8-tetramethyl-9H-xanthene (Table 2, entry 2)
IR (KBr): νmax = 3028, 2914, 1632, 1616, 1568, 1485, 1408, 1298, 1221, 1147, 1058, 841, 804 cm−1; 1H NMR (300 MHz, CDCl3): δ = 7.14 (d, J = 8.5 Hz, 2H), 7.08 (d, J = 8.5 Hz, 2H), 6.79 (s, 2H), 6.62 (s, 2H), 5.06 (s, 1H), 2.23 (s, 12H), 13C NMR (75 MHz, CDCl3): δ = 151.7, 143.1, 137.6, 136.3, 131.9, 129.7, 128.6, 126.1, 121.6, 115.1, 39.4, 21.0, 19.2; Anal calcd for C23H21ClO: C, 79.18; H, 6.07. Found: C, 79.14; H, 6.11.
4.2.3 1,3,6,8-tetramethyl-9-(4-nitrophenyl)-9H-xanthene (Table 2, entry 3)
IR (KBr): νmax = 3030, 2953, 2916, 1632, 1614, 1591, 1518, 1450, 1346, 1298, 1221, 1147, 1059, 839, 746 cm−1; 1H NMR (300 MHz, CDCl3): δ = 7.99 (d, J = 8.8 Hz, 2H), 7.37 (d, J = 8.8 Hz, 2H), 6.82 (s, 2H), 6.64 (s, 2H), 5.21 (s, 1H), 2.23 (s, 12H), 13C NMR(75 MHz, CDCl3): δ = 151.8, 151.6, 146.2, 138.2, 136.3, 129.1, 126.3, 123.8, 120.7, 115.4, 39.9, 21.1, 19.2; Anal calcd for C23H21NO3: C, 76.86; H, 5.89; N, 3.90. Found: C, 76.95; H, 5.96; N, 3.88.
4.2.4 1,3,6,8-tetramethyl-9-p-tolyl-9H-xanthene (Table 2, entry 4)
IR (KBr): νmax = 3025, 2918, 1632, 1615, 1568, 1454, 1408, 1298, 1219, 1148, 1057, 843, 797 cm−1; 1H NMR (300 MHz, CDCl3): δ = 7.10 (d, J = 8.0 Hz, 2H), 6.92 (d, J = 7.9 Hz, 2H), 6.78 (s, 2H), 6.60 (s, 2H), 5.04 (s, 1H), 2.24 (s, 6H), 2.21 (s, 6H), 2.16 (s, 3H), 13C NMR(75 MHz, CDCl3): δ = 151.8, 141.7, 137.3, 136.5, 135.8, 129.2, 128.4, 126.1, 122.4, 115.1, 39.7, 21.2, 21.1, 19.4; Anal calcd for C24H24O: C, 87.76; H, 7.37. Found: C, 87.68; H, 7.44.
4.3 General procedure for the preparation of bisphenol derivatives
To a mixture of aldehyde (1 mmol) and xylenol (2.2 mmol), pTSA (0.1 mmol) was added. The reaction mixture was stirred magnetically at 100 °C for the appropriate time as shown in Table 2. The progress of the reaction was monitored by TLC. After the completion of the reaction, the reaction mixture was cooled to room temperature, and a mixture of EtOH–H2O (3:1) was added to it. The suspension was stirred for 10 min and the precipitate was filtered. The crude products were purified by recrystallization from a mixture of EtOH–H2O (3:1).
4.3.1 6,6′-(phenylmethylene)bis(3,4-dimethylphenol) (Table 2, entry 5)
IR (KBr): νmax = 3555, 3476, 3019, 2918, 1616, 1508, 1456, 1402, 1279, 1194, 1057, 860, 696 cm−1; 1H NMR (300 MHz, DMSO-d6): δ = 8.77 (s, 2H), 7.13 (t, J = 7.3 Hz, 2H), 7.05 (t, J = 7.0 Hz, 1H), 6.86 (d, J = 7.5 Hz, 2H), 6.47 (s, 2H), 6.31 (s, 2H), 5.81 (s, 1H), 2.00 (s, 6H), 1.89 (s, 6H); 13C NMR (75 MHz, DMSO-d6): δ = 151.2, 141.8, 136.5, 131.0, 129.3, 129.0, 128.6, 126.6, 126.1, 117.7, 44.3, 19.5, 19.1; Anal calcd for C23H24O2: C, 83.10; H, 7.28. Found: C, 83.14; H, 7.33.
4.3.2 6,6′-(p-tolylmethylene)bis(3,4-dimethylphenol) (Table 2, entry 6)
IR (KBr): νmax = 3470, 3416, 3017, 2918, 1611, 1510, 1456, 1404, 1273, 1186, 1058, 999, 852 cm−1; 1H NMR (300 MHz, DMSO-d6): δ = 8.71 (s, 2H), 6.93 (d, J = 7.4 Hz, 2H), 6.74 (d, J = 7.7 Hz, 2H), 6.45 (s, 2H), 6.31 (s, 2H), 5.76 (s, 1H), 2.16 (s, 3H), 2.00 (s, 6H), 1.89 (s, 6H); 13C NMR (75 MHz, DMSO-d6): δ = 153.0, 142.1, 134.6, 134.5, 131.1, 129.4, 128.9, 128.1, 125.5, 116.8, 42.0, 21.1, 19.7, 19.2; Anal calcd for C24H26O2: C, 83.20; H, 7.56. Found: C, 83.29; H, 7.47.
4.3.3 6,6′-((4-chlorophenyl)methylene)bis(3,4-dimethylphenol) (Table 2, entry 7)
IR (KBr): νmax = 3466, 3412, 3013, 2920, 1616, 1578, 1508, 1489, 1404, 1279, 1192, 1155, 1089, 1012, 860, 812 cm−1; 1H NMR (300 MHz, DMSO-d6): δ = 8.82 (s, 2H), 7.19 (d, J = 7.8 Hz, 2H), 6.86 (d, J = 7.9 Hz, 2H), 6.48 (s, 2H), 6.31 (s, 2H), 5.78 (s, 1H), 2.01 (s, 6H), 1.90 (s, 6H); 13C NMR (75 MHz, DMSO-d6): δ = 153.0, 144.3, 134.9, 131.2, 131.1, 130.5, 128.2, 127.4, 125.8, 116.9, 42.1, 19.7, 19.2; Anal calcd for C23H23ClO2: C, 75.30; H, 6.32. Found: C, 75.33; H, 6.26.
4.3.4 6,6′-((4-chlorophenyl)methylene)bis(2,4-dimethylphenol) (Table 2, entry 8)
IR (KBr): νmax = 3503, 3025, 2917, 1616, 1487, 1406, 1254, 1219, 1184, 1136, 1088, 1014, 847, 725 cm−1; 1H NMR (300 MHz, DMSO-d6): δ = 7.93 (s, 2H), 7.22 (d, J = 8.2 Hz, 2H), 6.90 (d, J = 8.3 Hz, 2H), 6.66 (s, 2H), 6.20 (s, 2H), 6.05 (s, 1H), 2.03 (s, 6H), 1.98 (s, 6H); 13C NMR(75 MHz, DMSO-d6): δ = 150.7, 144.1, 131.4, 131.3, 130.7, 129.8, 128.3, 128.1, 127.5, 124.6, 43.2, 20.9, 17.2; Anal calcd for C23H23ClO2: C, 75.30; H, 6.32. Found: C, 75.23; H, 6.37.
4.3.5 6,6′-((3-nitrophenyl)methylene)bis(2,4-dimethylphenol) (Table 2, entry 9)
IR (KBr): νmax = 3432, 3007, 2912, 1523, 1487, 1348, 1319, 1217, 1165, 862, 739 cm−1; 1H NMR (300 MHz, DMSO-d6): δ = 8.05 (s, 2H), 7.97 (d, J = 8.1 Hz, 1H), 7.66 (s, 1H), 7.49 (t, J = 7.9 Hz, 1H), 7.38 (d, J = 7.4 Hz, 1H), 6.70 (s, 2H), 6.24 (s, 2H), 6.19 (s, 1H), 2.05 (s, 6H), 1.99 (s, 6H); 13C NMR (75 MHz, DMSO-d6): δ = 150.7, 148.1, 147.6, 136.4, 130.4, 130.1, 129.9, 128.1, 127.8, 124.8, 123.5, 121.4, 43.6, 20.9, 17.2; Anal calcd for C23H23NO4: C, 73.19; H, 6.14; N, 3.71. Found: C, 73.31; H, 6.22; N, 3.75.
4.3.6 4,4′-(phenylmethylene)bis(2,5-dimethylphenol) (Table 2, entry 10)
IR (KBr): νmax = 3545, 3397, 3020, 2966, 2922, 1622, 1587, 1506, 1462, 1410, 1279, 1157, 1068, 991, 900, 739, 700 cm−1; 1H NMR (300 MHz, DMSO-d6): δ = 8.91 (s, 2H), 7.19 (t, J = 7.1 Hz, 2H), 7.10 (t, J = 7.0 Hz, 1H), 6.91 (d, J = 7.4 Hz, 2H), 6.49 (s, 2H), 6.23 (s, 2H), 5.34 (s, 1H), 1.90 (s, 6H), 1.85 (s, 6H); 13C NMR (75 MHz, DMSO-d6): δ = 153.7, 144.2, 134.4, 132.9, 131.1, 129.8, 128.6, 126.3, 120.6, 117.1, 48.7, 19.4, 16.4; Anal calcd for C23H24O2: C, 83.10; H, 7.28. Found: C, 83.07; H, 7.21.
4.3.7 4,4′-((4-bromophenyl)methylene)bis(2,5-dimethylphenol) (Table 2, entry 11)
IR (KBr): νmax = 3441, 2947, 2920, 1616, 1508, 1458, 1406, 1281, 1153, 1072, 995, 902, 810, 758 cm−1; 1H NMR (300 MHz, DMSO-d6): δ = 8.95 (s, 2H), 7.38 (d, J = 8.1 Hz, 2H), 6.85 (d, J = 8.1 Hz, 2H), 6.49 (s, 2H), 6.20 (s, 2H), 5.33 (s, 1H), 1.89 (s, 6H), 1.86 (s, 6H); 13C NMR(75 MHz, DMSO-d6): δ = 153.8, 143.8, 134.5, 132.3, 132.0, 131.5, 131.1, 120.8, 119.4, 117.2, 48.1, 19.3, 16.4; Anal calcd for C23H23BrO2: C, 67.16; H, 5.64. Found: C, 67.25; H, 5.55.
4.3.8 4,4′-((3-nitrophenyl)methylene)bis(2,5-dimethylphenol) (Table 2, entry 12)
IR (KBr): νmax = 3441, 3348, 3017, 2914, 1618, 1591, 1529, 1458, 1406, 1357, 1284, 1155, 1074, 993, 904, 804, 735 cm−1; 1H NMR (300 MHz, DMSO-d6): δ = 9.01 (s, 2H), 8.00 (d, J = 7.5 Hz, 1H), 7.74 (s, 1H), 7.51 (t, J = 7.9 Hz, 1H), 7.38 (d, J = 7.7 Hz, 1H), 6.52 (s, 2H), 6.20 (s, 2H), 5.57 (s, 1H), 1.92 (s, 6H), 1.86 (s, 6H); 13C NMR(75 MHz, DMSO-d6): δ = 154.1, 148.3, 146.9, 136.5, 134.7, 131.7, 131.2, 130.0, 123.9, 121.6, 121.1, 117.4, 48.2, 19.3, 16.3; Anal calcd for C23H23NO4: C, 73.19; H, 6.14; N, 3.71. Found: C, 73.10; H, 6.24; N, 3.69.
Acknowledgment
We gratefully acknowledge the financial support from the Research Council of Arak University.