1 Introduction
Heterocyclic chemistry occupies an important place in organic chemistry research worldwide [1–4] and forms the basis of many pharmaceutical, agrochemical and veterinary products. Especially, 2,3-dihydro-2-aryl-4(1H)-quinazolinones are a class of fused heterocycles that have drawn much attention due to their potentially biological and pharmaceutical activities as anti-tumor, diuretic, and herbicidal agents, as well as plant growth regulators [5]. Three-component reaction of isatoic anhydride, aldehyde and amine [6–10], reduction cyclization of o-nitrobenzamides and orthoformate, aldehydes or ketones [11] and reduction of quinazolin-4(3H)-ones [12] are also reported for the synthesis of 2,3-dihydroquinazolin-4(1H)-ones. However, most of the reported methods suffer from some limitations, such as long reaction times, harsh reaction conditions, low yields, tedious work-up and multi-step reaction. Therefore, the development of a clean, high yielding and eco-friendly approach is highly desirable. In recent years, the development of efficient and environmentally benign chemical processes or methodologies for widely used recyclable catalysts and unharmful solvents has been one of the major challenges for chemists in organic synthesis [13].
Cobalt is an important non-ferrous metal and is extensively applied in various processes due to its high activity and low cost. The unique characteristics of cobalt metal, especially its magnetic properties, make it attractive in wide fields, such as data information storage [14], recording devices, magnetic refrigeration, including biomedical systems [15], magnetic alloys, high-temperature alloys, cemented carbides, and catalysts [16]. The support plays a significant role in dispersion, reducibility changes, and catalytic behaviour of active cobalt metal [17–19]. In order to achieve high-surface active sites, supported cobalt catalysts were known to be highly active for various supports, including silica, alumina, titania, magnesia, kieselguhr, and zeolite [17,20–27]. A limitation of some of these support materials is their reactivity toward cobalt for the formation of mixed compounds that are reducible only at high reduction temperatures [20–23]. To avoid these problems, the use of activated carbon as a catalyst support has been reported. The metal–carbon interaction is not so strong and the cobalt catalyst can therefore be completely reduced [28–30]. On the other hand, interactions of CNTs with metal particles have been the topic of current investigations. The studies have pointed out that their special and steady structural characteristics and morphology are quite suitable for use as catalyst support materials [29–34]. Especially, carbon nanotubes (CNT) as support exhibit superior catalytic activity due to the fact they prevent the congregation of nanoparticles and enhance the catalytic active sites. Also, CNTs in the nanocomposites can provide continuous conducting pathways for faster electron transfer [35]. Therefore, the combination of Co NPs with CNTs has attracted considerable interest for their wide applications in the fields of catalysis. We herein disclose a novel, highly efficient condensation of the appropriate derivatives of aldehyde with o-aminobenzamide using Co-CNT as a catalyst and solvent for the preparation of 2-aryl-2,3-dihydroquinazolin-4(1H)-ones under solvent-free conditions (Scheme 1). To the best of our knowledge, this is the first report dealing with the eco-friendly formation of a 2-aryl-2,3-dihydroquinazolin-4(1H)-one heterocyclic system using this catalytic system.
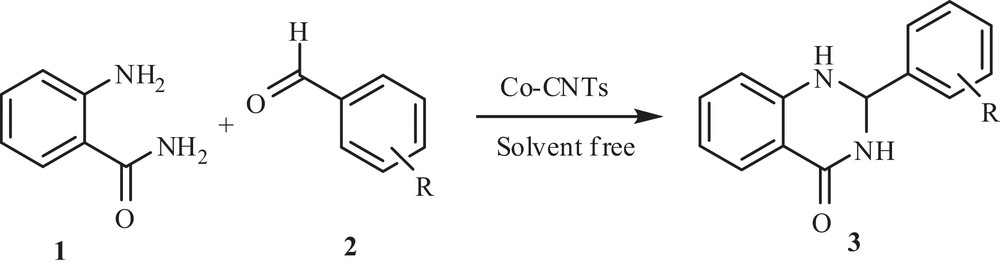
Co-CNT-catalyzed green synthesis of 2-aryl-2,3-dihydroquinazolin-4(1H)-ones.
2 Results and discussion
As part of our interest in the synthesis of heterocyclic compounds and due to the present awareness of applying environmentally benign strategies in organic synthesis, we started a series of studies aimed at the development of a new selective method for the synthesis of 2-aryl-2,3-dihydroquinazolin-4(1H)-ones using cobalt/multi-walled carbon nanotube as the catalysts. The results obtained from the Co-supported-catalyzed synthesis of 2-aryl-2,3-dihydroquinazolin-4(1H)-ones (3a-j) from cyclo-condensation of o-aminobenzamide with various aldehydes under solvent-free conditions are summarized in Table 1. Indeed, in order to evaluate the novelty and impact of the result, a comparison catalytic test with a commercial Co/AC catalyst is carried out. As shown in Table 1, Co-CNTs can act as high-efficiency catalysts with short reaction times and high yields of the obtained products. In general, the main advantages of CNT supports compared to activated carbon (AC) are the high purity of the material, which can avoid self-poisoning, exceptionally high mechanical strength, medium to high specific surface areas, high thermal conductivity, high external surface area, and specific metal support interactions that can directly affect the catalytic activity, the full accessibility of the reactants to the active sites, and selectivity [36]. From the data in Table 1, it can be concluded that the presence of the electron-withdrawing substituents on the aromatic ring can increase the reaction rate and, as a result, the reaction times are shortened and the yields are increased while the electron-donating substituents have diverse effects. The reaction of o-aminobenzamide with benzaldehyde as a model experiment was examined during different times to determine the optimal reaction time (Fig. 1). Then, we examined the effect of the molar amounts of catalyst employed in this cyclo-condensation (Table 2). Finally, the recyclability of the catalyst was investigated. For this purpose, the reaction of anthranilamide and benzaldehyde in ethanol and in the presence of Co-CNT was investigated. After the reaction was complete (monitored by TLC), followed by filtration, the filtrate was saved for the next reaction. When the same reaction was performed in this filtrate containing the used catalyst, lower yields (94%) of the product were obtained. According to the same procedure, the recyclability in the next runs was examined under the same conditions. It could result from a loss of catalyst while the filtration was conducted. The results of this study are presented in Fig. 2.
Catalytic effect of Co-supported catalysts in cyclization of o-aminobenzamide with various aromatic aldehydes.
Entry | R | Product | Co-CNTs | Co-AC | Mp (°C) | |
Time (min)/Yield (%) | Time (min)/Yield (%) | Found | Reported | |||
1 | H | 3a | 8/96 | 25/52 | 216–218 | 217–219 [37] |
2 | 4-OH | 3b | 20/88 | 35/46 | 279–280 | 278–280 [8] |
3 | 3-NO2 | 3c | 5/92 | 20/55 | 196–198 | 195–196 [37] |
4 | 2,4-(OMe)2 | 3d | 17/95 | 40/59 | 184–186 | 186–187 [8] |
5 | 4-OMe | 3e | 15/85 | 35/40 | 181–183 | 182–184 [38] |
6 | 4-Cl | 3f | 12/91 | 30/58 | 205–206 | 206–207 [37] |
7 | 4-Me | 3g | 6/95 | 22/50 | 225–227 | 224–226 [37] |
8 | 4-CN | 3h | 5/89 | 20/49 | 250–252 | 249–251 [39] |
9 | 3-F | 3i | 12/93 | 30/54 | 264–266 | 266–267 [8] |
10 | 2-Naphthyl | 3j | 20/87 | 40/46 | 225–226 | 225–227 [40] |

Effect of time on the preparation of compound 3a.
Optimization of the molar amounts of catalyst for compound 3a.
Catalyst (g) | 0.001 | 0.003 | 0.005 | 0.008 | 0.01 |
Time (min) | 25 | 20 | 14 | 8 | 8 |
Yield (%) | 81 | 87 | 90 | 96 | 96 |
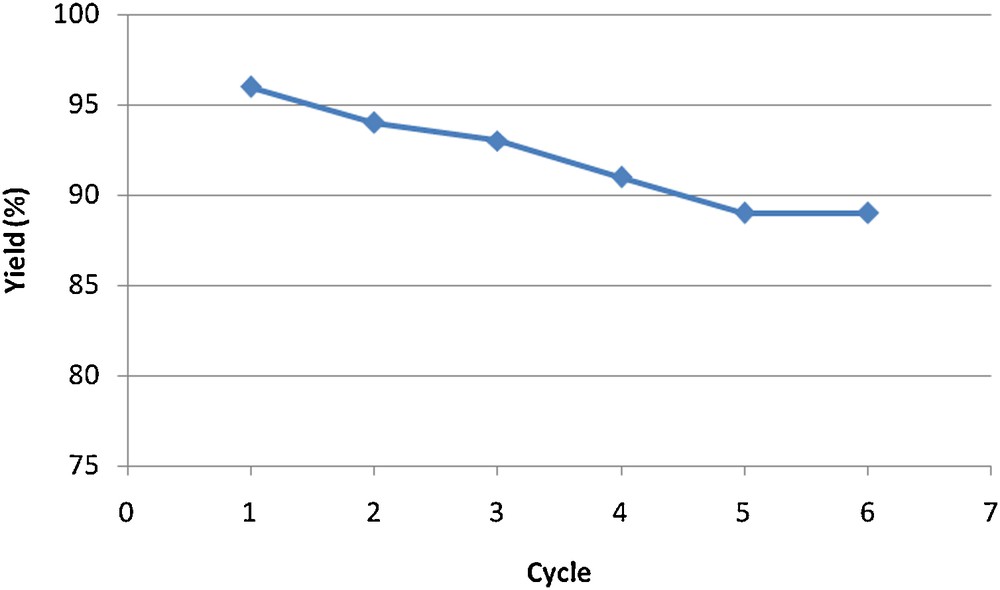
Reuse of Co-CNT for the synthesis of compound 3a.
Thus, the Co-CNT nanocomposites also exhibit a significant catalytic effect under solvent-free conditions for the synthesis of 2-aryl-2,3-dihydroquinazolin-4(1H)-one derivatives with short reaction times. The advantage of this synthesis route is its application to parallel synthesis that permits an easy and rapid access to a large number of derivatives for biological evaluation as well as potential chemotherapeutic agents. The spectral data, structures and purity of these compounds were confirmed by IR and (1H and 13C) NMR and were in complete agreement with the reported data. In each case, the target product was obtained in excellent yield and with a high degree of purity.
A cobalt sample supported on CNTs was analyzed by TEM and SEM. The TEM images of the supported catalyst revealed that the dark spots represent the cobalt, which was distributed inside the tubes and also on the outer surface of the CNTs. This can be attributed to the tubular morphology of graphene layers, which can induce capillary forces during the impregnation process. The most of the CNTs have been completely decorated with Co nanoparticles. Also, the distribution of Co nanoparticles inside the tubes is quite uniform and no local aggregation is detected. It is known that the exterior surfaces of the CNTs are electron-enriched, while the interior ones are electron-deficient [41–43], which could influence the structure and electronic properties of the substrates in contact with either surface. The scanning electron microscopy (SEM) picture reveals that nanotubes are completely covered with the supporting materials and that there are no other impurities. In addition, the SEM picture of the composites displays more efficiently the small cobalt particles outside the tubes. The particles inside the tubes are fairly uniform; this is due to the fact that CNT channels have regulated the growth of the metal particles inside the tubes [44] (Fig. 3).

TEM image of cobalt supported on acid-treated CNTs (a); SEM image Co-CNTs composites (b).
Fig. 4 presents the XRD patterns of CNT-COOH and Co/CNT composites. In the XRD spectrum of the acid treated CNTs, the diffraction peak at 25.92° is assigned to the (002) plane of CNTs, while the other peaks in the spectrum of the composite are related to different crystal planes of Co. The diffraction peaks at 45.16°, 48.19° and 76.64° are attributed to the (100), (101), and (220) planes of the hexagonal-phase structure of cobalt, and the peak at 50.36° corresponds to the (200) crystal plane of the cubic structure. This indicates that the structure of the Co nanoparticle-decorated CNTs is a mixture of hexagonal and cubic phases. In addition, the peak broadening indicates that the size of the Co nanoparticles is significantly small.

X-Ray diffraction pattern of acid treated CNTs and CNT/Co nanocomposites.
According to the evolution of the reaction conditions as well as to other reported mechanisms in the literature, a possible mechanism for the synthesis of these products is presented in Scheme 2. It is proposed that, at first, the intermediate I is formed through the nucleophilic attack of the amino group in 2-aminobenzamide at the activated carbonyl group of the aldehyde by the metal as a supported catalyst. Also, the part of amide in the imine intermediate I is converted into its tautomer in the presence of a catalyst. Then, the Schiff-base intermediate II is produced by dehydration of the intermediate I. Then, the intramolecular nucleophilic addition reaction between the amide group nitrogen and the activated Schiff base carbon gives the intermediate III and is followed by a 1,5-proton transfer, affording the products.
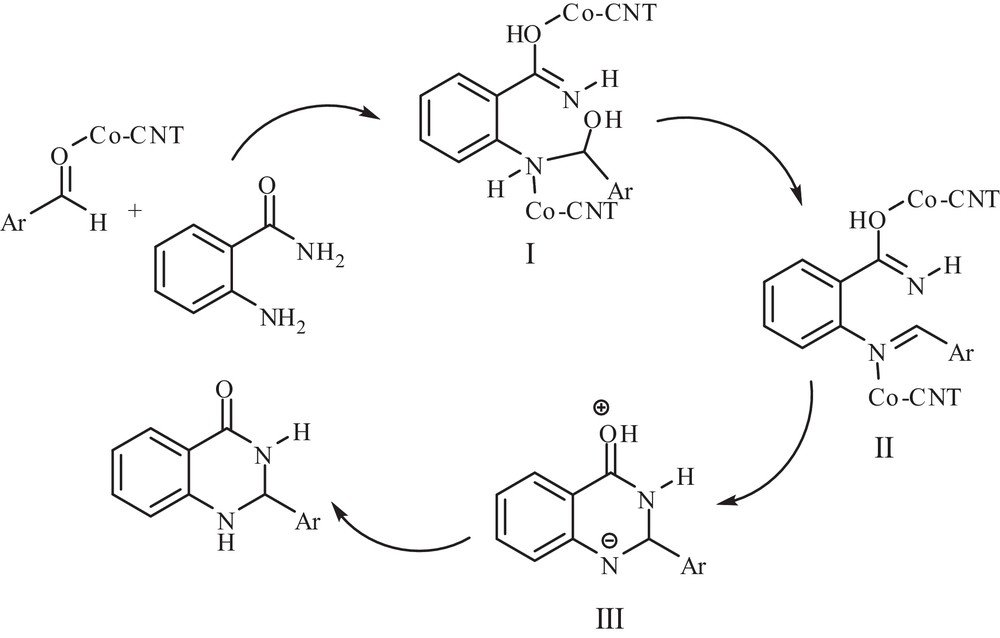
Possible mechanism for the formation of dihydroquinazolinone derivatives.
3 Experimental
All the chemicals were obtained from Merck Company and used as received. MWNTs were purchased from Nanotech Port Co. (Taiwan). These MWNTs were produced via the chemical vapor deposition (CVD, or sometimes called catalytic pyrolysis) method. The outer diameter of CNT was between 20 and 40 nm. Melting points (°C) were determined on an Electrothermal MK3 apparatus using an open-glass capillary and are uncorrected. The IR spectra were obtained with a PerkinElmer FT–IR 550 spectrophotometer (KBr pellets). 1H and 13C NMR spectra were recorded using a Bruker DRX–400 spectrometer and chemical shifts (δ) are reported in ppm downfield from an internal TMS standard. ESI–MS was recorded on a Micromass Quattro II triple-quadrupole mass spectrometer. Microwave oven reactions were performed on a CEM–Discover Focused Monomode, with a microwave input power of 300 W and a microwave frequency of 2450 MHz. The purity of the compounds and the completion of the reactions were monitored by thin layer chromatography (TLC) on silica gel plates in the solvent system petroleum ether–EtOAc (V/V = 1:1). Elemental analysis was determined by a Heraeus CHN-O-RAPID elemental analyzer. The structure of the products was also confirmed by conventional techniques (1H and 13C NMR). XRD patterns were obtained on a Holland Philips Xpert X-ray powder diffraction (XRD) diffractometer (Cu Kα radiation, λ = 0.154056 nm), at a scanning speed of 2°/min from 10° to 100° (2θ). SEM images were taken in a FEI Quanta 200 Scanning electron microscope (SEM), operating at a 20 kV. Transmission electron microscopy (TEM) micrographs were taken with a Jeol JEM-2100UHR operating at 200 kV.
3.1 Preparation of the catalyst
Co-CNT nanocomposites were prepared according to the procedures reported in the literature [45]. Briefly, CNTs were first refluxed at 140 °C for 4 h in concentrated HNO3. Then, they were filtered out and washed with distilled water until a neutral pH was reached, and then dried at 120 °C for 8 h. An amount of 0.06 g of oxidized MWCNTs was dispersed in 100 mL of an ethylene glycol (EG) solution containing CoCl2 (0.05 M) under sonication. After 10 min, 0.6 g NaOH, 2.65 g Na2CO3, and 3.5 mL N2H4·H2O were added sequentially. Then, the solution was heated in a microwave oven for 60 s. After that, the product was washed with distilled water and absolute ethanol, then dried under vacuum at room temperature.
3.2 General method for preparing 2-aryl-2,3-dihydroquinazolin-4(1H)-one derivatives
Co-CNTs (0.008 g) were added to a stirred mixture of 2-aminobenzamide (1 mmol) and benzaldehyde (1 mmol) under solventless conditions. After completion of the reaction, which was monitored by TLC using chloroform:methanol (1:3) as the eluent, ice water (15 mL) was added to it and the resulting precipitate was filtered out, washed with water, dried under vacuum and purified by recrystallization from ethanol to give the corresponding product. Yields, melting points, and times of reaction of all the subsequent compounds are summarized in Table 1.
3.3 Spectral data of the products
3.3.1 2-Phenyl-2,3-dihydroquinazolin-4(1H)-one C14H12N2O (3a)
White solid; Mp 216–218 °C; Rf = 0.4 (petroleum ether–EtOAc, 1:1); Anal. calcd. for C14H12N2O (%) C 74.98, H 5.39, N 12.49. Found C 74.94, H 5.43, N 12.52. IR (KBr, cm−1): 3304 (NH), 3186 (NH), 1654 (CO), 1613 (CC). 1H NMR (400 MHz, DMSO-d6): δ 8.30 (s, 1H, NH–CO), 7.61 (t, J = 6.8 Hz, 1H, ArH), 7.50 (d, J = 7.2 Hz, 2H, ArH), 7.32–7.41 (m, 3H, ArH), 7.22–7.26 (m, 1H, ArH), 7.12 (s, 1H, NH), 6.75 (d, J = 8.0 Hz, 1H, ArH), 6.67 (t, J = 7.2 Hz, 1H, ArH), 5.75 (s, 1H, CH). 13C-NMR (100 MHz, DMSO-d6): δ 163.6, 147.9, 141.6, 133.3, 128.5, 128.3, 127.4, 126.9, 117.1, 115.0, 114.4, 66.6. HRMS (ESI) for [M+H] found (expected): 224.0945 (224.0949).
3.3.2 2-(4-Hydroxylphenyl)-2,3-dihydroquinazolin-4(1H)-one C14H12N2O2 (3b)
White solid; Mp 279–280 °C; Rf = 0.3 (petroleum ether–EtOAc, 1:1); Anal. calcd. for C14H12N2O2 (%) C 69.99, H 5.04, N 11.66. Found C 69.91, H 5.10, N 11.71. IR (KBr, cm−1): 3399 (OH), 3337 (NH), 3184 (NH), 1670 (CO), 1605 (CC), 1237 (C–O). 1H NMR (400 MHz, DMSO-d6): δ 9.51 (s, 1H, NH–CO), 8.35 (s, 1H, NH), 7.59–7.61 (m, 3H, ArH), 7.4 (d, J = 7.6 Hz, 2H, ArH), 7.23–7.27 (m, 1H, ArH), 7.15 (s, 1H, OH), 6.74 (d, J = 7.6 Hz, 1H, ArH), 6.74 (t, J = 7.6 Hz, 1H, ArH), 5.75 (s, 1H, CH). 13C NMR (100 MHz, DMSO-d6): δ 165.2, 149.3, 140.7, 130.4, 129.3, 127.7, 126.2, 125.4, 119.4, 115.0, 114.5, 66.7. HRMS (ESI) for [M+H] found (expected): 240.0895 (240.0898).
3.3.3 2-(3-Nitrophenyl)-2,3-dihydroquinazolin-4(1H)-one C14H11N3O3 (3c)
Light yellow solid; Mp 196–198 °C; Rf = 0.4 (petroleum ether–EtOAc, 1:1); Anal. calcd. for C14H11N3O3 (%) C 62.45, H 4.12, N 15.61. Found C 62.53, H 4.14, N 15.54. IR (KBr, cm−1): 3337 (NH), 3146 (NH), 1673 (CO), 1635 (CC), 1526 (NO2), 1347 (NO2). 1H NMR (400 MHz, DMSO-d6): δ 8.56 (s, 1H, NH–CO), 8.38 (t, J = 2.0 Hz, 1H, ArH), 8.20–8.23 (m, 1H, ArH), 7.96 (d, J = 7.6 Hz, 1H, ArH), 7.71 (t, J = 8.0 Hz, 1H, ArH), 7.63 (d, J = 1.6, 7.6 Hz, 1H, ArH), 7.37 (s, 1H, NH), 7.26–7.30 (m, 1H, ArH), 6.80 (d, J = 8.0 Hz, 1H, ArH), 6.69–6.73 (m, 1H, ArH), 5.97 (s, 1H, CH). 13C NMR (100 MHz. DMSO-d6): δ 163.5, 147.7, 147.4, 144.3, 133.7, 133.4, 130.1, 127.5, 123.3, 121.6, 117.6, 115.0, 114.7, 65.2. HRMS (ESI) for [M+H] found (expected): 269.0796 (269.0800).
3.3.4 2-(2,4-Dimethoxyphenyl)-2,3-dihydroquinazolin-4(1H)-one C16H16N2O3 (3d)
White solid; Mp 184–186 °C; Rf = 0.35 (petroleum ether–EtOAc, 1:1); Anal. calcd. for C16H16N2O3 (%) C 67.59, H 5.67, N 9.85. Found C 67.54, H 5.71, N 9.90. IR (KBr, cm−1): 3363 (NH), 3178 (NH), 1670 (CO), 1639 (CC), 1253 (C–O), 1048 (C–O). 1H NMR (400 MHz, DMSO-d6): δ 7.95 (s, 1H, NH-CO), 7.62 (d, J = 8.0 Hz, 1H, ArH), 7.32 (d, J = 8.4 Hz, 1H, ArH), 7.20–7.23 (m, 1H, ArH), 6.76 (d, J = 8.0 Hz, 1H, ArH), 6.72 (s, 1H, NH), 6.66 (t, J = 7.6 Hz, 1H, ArH), 6.5–6.59 (m, 2H, ArH), 5.95 (s, 1H, CH), 3.81 (s, 3H, OCH3), 3.75 (s, 3H, OCH3). 13C-NMR (100 MHz, DMSO-d6): δ 164.0, 160.7, 157.5, 148.2, 133.2, 127.8, 127.3, 121.3, 117.0, 114.8, 114.5, 104.5, 98.4, 60.8, 55.6, 55.3. HRMS (ESI) for [M+H] found (expected): 284.1156 (284.1160).
3.3.5 2-(4-Methoxyphenyl)-2,3-dihydroquinazolin-4(1H)-one C15H14N2O2 (3e)
White solid; Mp 181–183 °C; Rf = 0.35 (petroleum ether–EtOAc, 1:1); Anal. calcd. for C15H14N2O2 (%) C 70.85, H 5.55, N 11.02. Found C 70.81, H 5.48, N 11.07. IR (KBr, cm−1): 3370 (NH), 3296 (NH), 1650 (CO), 1608 (CC), 1240 (C–O), 1037 (C–O). .1H NMR (400 MHz, DMSO-d6): δ 8.19 (br s, 1H, NH–CO), 7.61 (d, J = 7.2 Hz, 1H, ArH), 7.42 (d, J = 8.4 Hz, 2H, ArH), 7.24 (t, J = 7.2 Hz, 1H, ArH), 7.01 (br s, 1H, NH), 6.94 (d, J = 7.6 Hz, 2H, ArH), 6.74 (d, J = 8.0 Hz, 1H, ArH), 6.67 (t, J = 7.2 Hz, 1H, ArH), 5.71 (s, 1H, CH), 3.74 (s, 3H, OCH3). 13C NMR (100 MHz, DMSO-d6): δ 163.7, 159.4, 148.0, 133.4, 133.2, 128.2, 127.4, 117.1, 115.0, 114.4, 113.6, 66.3, 55.2. HRMS (ESI) for [M+H] found (expected): 254.1050 (254.1055).
3.3.6 2-(4-Chlorophenyl)-2,3-dihydroquinazolin-4(1H)-one C14H11N2OCl (3f)
White solid; Mp 205–206 °C; Rf = 0.4 (petroleum ether–EtOAc, 1:1); Anal. calcd. for C14H11N2OCl (%) C 65.00, H 4.29, N 10.83. Found C 64.96, H 4.34, N 10.78. IR (KBr, cm−1): 3310 (NH), 3187 (NH), 1660 (CO), 1611 (CC), 1184 (C-Cl). 1H NMR (400 MHz, DMSO-d6) δ 8.36 (br s, 1H, NH-CO), 7.62 (d, J = 7.6 Hz, 1H, ArH), 7.52 (d, J = 8.0 Hz, 2H, ArH), 7.46 (d, J = 8.0 Hz, 2H, ArH), 7.25 (t, J = 7.6 Hz, 1H, ArH), 7.16 (br s, 1H, NH), 6.75 (d, J = 8.0 Hz, 1H, ArH), 6.69 (t, J = 7.6 Hz, 1H, ArH), 5.78 (s, 1H, CH). 13C NMR (100 MHz, DMSO-d6): δ 163.5, 147.7, 140.7, 133.4, 133.0, 128.8, 128.3, 127.4, 117.3, 115.0, 114.5, 65.8. HRMS (ESI) for [M+H] found (expected): 258.0556 (258.0560).
3.3.7 2-P-tolyl-2,3-dihydroquinazolin-4(1H)-one C15H14N2O (3g)
White solid; Mp 225–227 °C; Rf = 0.35 (petroleum ether–EtOAc, 1:1); Anal. calcd. for C15H14N2O (%) C 75.61, H 5.92, N 11.76. Found C 75.57, N 5.89, N 11.72. IR (KBr, cm−1): 3310 (NH), 3190 (NH), 1667 (CO), 1606 (CC). 1H NMR (400 MHz, DMSO-d6): δ 8.26 (s, 1H, NH–CO), 7.62 (d, J = 7.6 Hz, 1H, ArH), 7.38 (d, J = 8.0 Hz, 2H, ArH), 7.17–7.25 (m, 3H, ArH), 7.07 (s, 1H, NH), 6.75 (d, J = 8.0 Hz, 1H, ArH), 6.66 (t, J = 7.6 Hz, 1H, ArH), 5.72 (s, 1H, CH), 2.28 (s, 3H, CH3). 13C NMR (100 MHz, DMSO-d6): δ 163.7, 148.0, 138.7, 137.8, 133.3, 128.9, 127.4, 126.9, 117.1, 115.0, 114.5, 66.5, 20.8. HRMS (ESI) for [M+H] found (expected): 238.1101 (238.1106).
3.3.8 2-(4-Cyanophenyl)-2,3-dihydroquinazolin-4(1H)-one C16H10N4O (3h)
White solid; Mp 250–252 °C; Rf = 0.4 (petroleum ether–EtOAc, 1:1); Anal. calcd. for C16H10N4O (%) C 70.07, H 3.67, N 20.43. Found C 70.02, H 3.62 N 20.39. IR (KBr, cm−1): 3336 (NH), 3353 (NH), 2227 (CN), 1666 (CO), 1611 (CC). 1H NMR (400 MHz, DMSO-d6): δ 8.46 (s, 1H, NH–CO); 7.86 (d, J = 8.30 Hz, 2H, ArH), 7.66 (d, J = 8.25 Hz, 1H, ArH), 7.60 (d, J = 7.20 Hz, 1H, ArH), 7.23–7.27 (m, 2H, ArH and NH), 6.76 (d, J = 8.05 Hz, 1H, ArH), 6.67 (t, J = 7.45 Hz, 1H, ArH), 5.85 (s, 1H, CH). 13C NMR (100 MHz, DMSO-d6): δ 162.1, 150.9, 136.9, 134.7, 132.5, 128.6, 127.7, 127.2, 125.9, 121.2, 118.3, 113.6, 65.5. HRMS (ESI) for [M+H] found (expected): 274.0851 (274.0855).
3.3.9 2-(3-Fluorophenyl)-2,3-dihydroquinazolin-4(1H)-one C14H11N2OF (3i)
White solid; Mp 264–266 °C; Rf = 0.35 (petroleum ether–EtOAc, 1:1); Anal. calcd. for C14H11FN2O (%) C 69.41, H 4.58, N 11.56. Found C 69.33, H 4.62, N 11.52. IR (KBr, cm−1): 3360 (NH), 3124 (NH), 1675 (CO), 1620 (CC), 1190 (C–F). 1H NMR (400 MHz, DMSO-d6): δ 8.42 (s, 1H, NH–CO), 7.62 (d, J = 7.2 Hz, 1H, ArH), 7.41–7.46 (m, 1H, ArH), 7.15–7.35 (m, 5H, ArH & NH), 6.78 (d, J = 8.0 Hz, 1H, ArH), 6.69 (t, J = 7.2 Hz, 1H, ArH), 5.80 (s, 1H, CH). 13C NMR (100 MHz, DMSO-d6): δ 163.5, 162.0 (d, 1JCF = 242.7 Hz), 147.6, 144.8, 133.5, 130.4 (d, 3JCF = 7.6 Hz), 127.4, 122.8, 117.3, 115.2 (d, 2JCF = 21.2 Hz), 115.0, 114.5, 113.6 (d, 2JCF = 22.0 Hz), 65.6. HRMS (ESI) for [M+H] found (expected): 242.0850 (242.0855).
3.3.10 2-(2-Naphtyl)-2,3-dihydroquinazolin-4(1H)-one C18H14N2O (3j)
White solid; Mp 225–226 °C; Rf = 0.45 (petroleum ether–EtOAc, 1:1); Anal. calcd. for C18H14N2O (%) C 78.81, H 5.14, N 10.21. Found C 78.82, H 5.12, N 10.25. IR (KBr, cm−1): 3282 (NH), 3188 (NH), 1649 (CO), 1611 (CC). 1H NMR (400 MHz, DMSO-d6): δ 8.56 (d, J 6.8 Hz, 1H, ArH), 8.27 (s, 1H, NH–CO), 7.98 (t, J 8.4 Hz, 2H, ArH), 7.70 (t, J 6.4 Hz, 2H, ArH), 7.57 (m, 3H), 7.26 (t, J 7.6 Hz, 1H, ArH), 7.08 (s, 1H, NH), 6.74 (dd, J 8.0, 12.8 Hz, 2H, ArH), 6.49 (s, 1H, CH). 13C NMR (100 MHz, DMSO-d6): δ 163.9, 148.3, 135.1, 133.7, 133.2, 130.4, 129.2, 128.5, 127.4, 126.0, 125.7, 125.1, 124.4, 117.2, 114.9, 114.4, 65.8. HRMS (ESI) for [M+H] found (expected): 274.1102 (274.1106).
4 Conclusions
Because of the importance of quinazolinone analogues as synthons in organic synthesis, we have reported the synthesis of a number of these compounds through the Co-CNT catalyzed heterocyclization of o-aminobenzamide with different aldehydes. Mildness, short reaction times, work-up easiness are the superiorities of this green protocol for the synthesis of 2-aryl-2,3-dihydroquinazolin-4(1H)-ones, which can moreover be applied to large-scale reactions. Also, the synthesis route to Fe3O4/MWCNT composites is simple and can be carried out under ambient conditions. This method might be useful in the future for the preparation of similar derivatives.
Acknowledgment
We are thankful to the University of Kashan Research Council for partial support of this work (Grant No. 159198/20).