1 Introduction
In recent years, considerable attention has been focused on the development of efficient and operationally simple protocols for carbon–carbon and carbon–heteroatom bonds formation for the construction of valuable molecules. The development of bis(indolyl)alkane synthesis has been of considerable interest in organic synthesis because of their wide occurrence in various natural products possessing biological activity and usefulness for drug design [1].
Bis(indolyl)alkane is known to promote estrogen metabolism in both women and men, and is expected to have an application in the prevention of breast cancer [2]. Thus, the development of facile and environmentally friendly synthetic methods for the preparation of these compounds constitutes an active area of investigation in pharmaceutical and organic synthesis [3–5].
Synthetically the reaction of 1H-indole with aldehydes or ketones produces azafulvenium salts that react further with a second 1H-indole molecule to form bis(indol-3-yl)methanes [6]. In recent years, synthesis routes to this class of molecules under mild conditions have been reported, with promoters such as montmorillonite clay K-10 [7], trichloro-1,3,5-triazine [8], AlPW12O40 [9], sodium dodecyl sulfate (SDS) [10], ZrCl4 [11], H2NSO3H [12], I2 [4], zeolites [13], bentonite [14], In(OTf)3/ionic liquid [15], CuBr2 [16], Dy(OTf)3/ionic liquid [17], HClO4–SiO2 [18], InCl3 [19], MW/Lewis acids (FeCl3, BiCl3, InCl3, ZnCl2, CoCl2) [20], NaHSO4 and Amberlyst-15 [21], sulfated zirconia [22], ZrOCl2/SiO2 [23], silica sulfuric acid (SSA) [24], TiO2 [25], (NH4)2HPO4 [26], acidic ionic liquid [27], NaBF4 [28], metal hydrogen sulfates [29], tetrabutylammonium tribromide [30], superacid SO42−/TiO2 [31], NaHSO4/ionic liquid [32], NBS [33], Ph3CCl [34], H3PW12O40 [35], LiClO4 [36], Zr(DS)4 [37], and Bi(NO3)3.5H2O [38]. However, most of the existing methods involve toxic metal ions and solvent, high cost and cumbersome work-up procedures. Consequently, new procedures that address these drawbacks are desirable.
2 Experimental
All commercially available chemicals were obtained from Merck and Fluka companies, and used without further purifications unless otherwise stated. 1H NMR spectra were recorded on a Jeol 90 MHz FT NMR spectrometer using TMS as internal standard and chemical shifts δ are in ppm. Infrared (IR) measurements were conducted on a PerkinElmer GX FT-IR spectrometer. All yields refer to isolated products.
2.1 Typical experimental procedure
To a flask containing anisole (1 mmol) were added POCl3 (1.1 mmol) and DMF (4 mmol) at 0 °C. After stirring for 3 h at 40 °C, indole (2 mmol) was added to the reaction mixture, which was then stirred for 1 h at r.t. After the reaction, the mixture was poured into a NaHCO3 (10%) solution and extracted with CHCl3 (3 × 20 mL). The organic layer was dried over Na2SO4, filtered, and evaporated to provide the corresponding bis(indolyl)methanes. The resulting crude material was purified by recrystallization from an ethanol/water mixture to afford the pure products.
2.2 Analytical data for the selected compounds
2.2.1 3,3′-Bisindolyl-4-methylphenylmethane (Table 1, entry 2)
Pinkish solid, m.p: 94–95 °C; IR (KBr): 3452, 3112, 3045, 2950, 1604, 1523, 1210, 1052, 765 cm−1; 1H NMR (CDCl3, 90 MHz, δ ppm): 2.38 (s, 3H), 5.85 (s, 1H), 6.70–7.55 (m, ArH, 14H), 7.85 (br s, NH, 2H); 13C NMR (75 MHz, CDCl3): δ 32.3, 55.0, 110.0, 110.9, 119.1, 119.2, 121.0, 121.9, 123.7, 127.6, 129.1, 132.5, 136.8, 156.2; MS: m/z 336; Anal. calcd. for C24H20N2: C, 85.68; H, 5.99; N, 8.33. Found: C, 85.45; H, 5.88; N, 8.14.
One-pot conversion of aromatics into the corresponding bis(indolyl)methanes through aromatic N,N-dimethyliminium chlorides.
Entry | Carbonyl compound | Product | Temperature (°C) | Time (min) | Yield (%)a | Mp (°C) | Mp (°C) references |
1 | 40 | 5 h | 55 | 124–125 | 124–125 [42] | ||
2 | 40 | 3 h | 65 | 94–95 | 93–94 [10] | ||
3 | 40 | 3 h | 90 | 190–192 | 192–193 [39] | ||
4 | 40 | 2 h | 90 | 225–227 | 226–227 [39] | ||
5 | 80 | 2 h | 85 | 224–226 | 225–226 [10] | ||
6 | 40 | 20 min | 80 | 243–245 | 243–245 [10] | ||
7 | 40 | 2 h | 90 | 253–254 | 251–253 [39] | ||
8 | 100 | 4 h | 60 | 278–279 | 278–279 [36] | ||
9 | 100 | 6 h | 80 | 290–292 | 291–293 [10] | ||
10 | 100 | 2 h | 82 | 282–284 | 280–282 [39] | ||
11 | 80 | 4 h | 70 | 150–152 | 150–153 [39] | ||
12 | 80 | 15 min | 75 | 322–324 | 320–322 [10] |
a Products were characterized by their physical properties, by comparison with authentic samples and by spectroscopic methods.
2.2.2 3,3′-Bisindolyl-4-methoxylphenylmethane (Table 1, entry 3)
Red solid; m.p: 190–192 °C; IR (KBr): 3408, 3056, 2932, 1597, 1486, 1450, 1335, 1102, 745 cm−1; 1H NMR (90 MHz, 90 MHz, δ ppm): 3.82 (s, 3H), 6.32 (s, 1H), 6.61 (s, 2H), 6.81–7.40 (m, ArH, 12H), 7.80 (br s, NH, 2H); 13C NMR (75 MHz, CDCl3): δ 32.3, 56.0, 110.0, 110.8, 119.2, 119.8, 120.6, 121.9, 123.7, 127.2, 129.9, 132.5, 136.9, 157.1; MS: m/z 352; Anal. calcd. for C24H20N2O: C, 81.79; H, 5.72; N, 7.95. Found: C, 81.24; H, 5.45; N, 7.86.
2.2.3 Tri-(indolyl)methane (Table 1, entry 6)
Yellow solid, m.p: 243–245 °C; 1H NMR (DMSOd6, 90 MHz, δ ppm): 9.67 (br s, 2H), 6.24 (s, 3H), 6.27–6.87 (m, 12H), 5.47 (s, 1H); IR (KBr) ν: 3403, 3043, 2918 cm−1; MS: m/z 361; 13C NMR (75 MHz, CDCl3): δ 31.6, 110.9, 111.9, 118.4, 119.5, 1121.2, 124.0, 126.3, 127.1, 128.5, 128.6, 137.0, 145.2; MS: m/z 361; Anal. calcd. for C25H19N3: C, 83.08; H, 5.30; N, 11.63. Found: C, 83.41; H, 5.48; N, 11.75.
2.2.4 3,3′-Bisindolyl-[2]thienylmethane (Table 1, entry 11)
Brown solid, m.p: 185–186 °C; IR (KBr): 3412, 1715, 1452, 1260, 1105 cm−1; 1H NMR (CDCl3, 90 MHz, δ ppm): 6.18 (s, 1H), 6.87 (s, 2H), 6.92–7.48 (m, ArH, 11H), 7.98 (br s, NH, 2H); 13C NMR (CDCl3): δ 42.1, 102.1, 106.7, 110.6, 112.6, 119.3, 120.3, 121.5, 122.6, 131.1, 136.6, 142.1, 152.5; MS: m/z 328; Anal. calcd. for C21H16N2S: C, 76.80; H, 4.91; N, 8.53. Found: C, 76.52; H, 5.10; N, 8.35.
2.2.5 3,3′-Bisindolyl-2-furylmethane (Table 1, entry 12)
Pinkish solid, m.p: 322–324 °C; 1H NMR (CDCl3, 90 MHz, δ ppm): 7.74 (br s, 2H), 7.32–7.63 (m, 9H), 7.09 (s, 2H), 6.21–6.35 (m, 2H), 5.82 (s, 1H); 13C NMR (CDCl3): δ 42.2, 102.4, 106.2, 110.6, 112.6, 119.3, 120.3, 121.4, 122.6, 131.1, 136.3, 142.1, 152.5; MS: m/z 312; Anal. calcd. for C21H16N2O: C, 80.75; H, 5.16; N, 8.97. Found: C, 80.67; H, 5.20; N, 8.85.
3 Result and discussion
Here, as part of our ongoing studies on the synthesis of heterocyclic compounds [39–42], we would like to report on the one-pot conversion of aromatics into the corresponding bis(indolyl)methanes (Scheme 1).
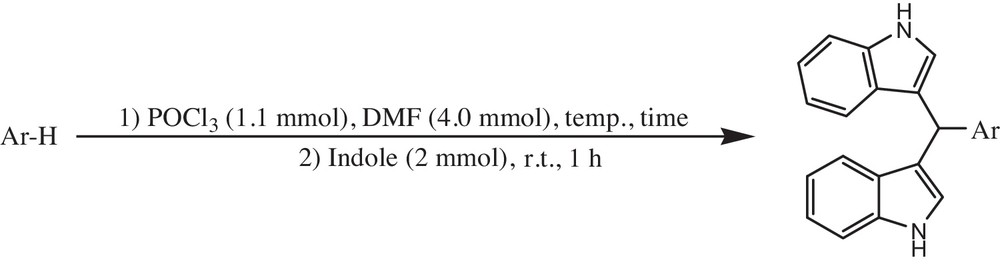
We planned to perform the metal-free one-pot conversion of aromatic compounds into the corresponding bis(indolyl)methanes using POCl3 and DMF, followed by treatment with indole at room temperature. The reaction was carried out as follows: to a flask containing aromatics were added POCl3 and DMF at 0 °C. Then, the mixture was warmed at room temperature, 40 °C, 80 °C, or 100 °C, depending on the substrate, for a few hours. Then, molecular indole was added to the reaction mixture, which was mixture was stirred for one hour at room temperature to provide the corresponding bis(indolyl)methanes. The results are shown in Table 1. All the substrates gave the corresponding bis(indolyl)methanes as a single isomer in good yields.
The plausible reaction mechanism is shown on Scheme 2. The initial step involves the Vilsmeier–Haack reaction to form aromatic N,N-dimethyliminium salt I [43]. Once the aromatic N,N-dimethyliminium salt I is formed, it reacts smoothly with indole to produce the corresponding azafulvene II, which further reacts with indole to generate the corresponding bis(indolyl)methane III.

4 Conclusion
In conclusion, a simple and convenient method has been developed for the direct conversion of various electron-rich aromatics into the corresponding bis(indolyl)methanes in good to moderate yields by the Vilsmeier–Haack reaction. This is the first report on the synthesis of bis(indolyl)methanes from the corresponding electron-rich aromatic compounds. The present reaction is a novel metal-free one-pot conversion of aromatics into the corresponding bis(indolyl)methanes, although the reaction is limited to electron-rich aromatics. The advantages of the present reaction are operational simplicity, low cost, low toxicity, and easy availability of reaction materials. Therefore, we believe that the present reactions are a useful and environmentally benign method for the preparation of bis(indolyl)methanes from aromatics directly.
Acknowledgment
We are thankful to Payame Noor University for partial support of this work.