1 Introduction
Quinoline and its derivatives are important intermediates in organic synthesis and exhibit various physiological properties and pharmacological activities, such as antiparasitic [1], antitubercular [2], antifilarial [3], anti-platelet [4], HMG-CoA reductase inhibiting [5], anti-allergic, anti-inflammatory [6–9], anti-asthmatic [10,11], antibacterial [12–15], antihypertensive [16,17], anticancer [18–21], tyrosine kinase inhibitory agents [22], anti-proliferative [23], antimalarials [24,25], and antifungals [26,27]. Furthermore, these compounds find applications in chemistry of transition metal catalysts for uniform polymerization and luminescence chemistry [28]. Quinoline derivatives also act as antifoaming agents in refineries [29]. They are used in a variety of nano-structures and meso-structures with enhanced electronic and photonic functions [30–32].
Owing to such significance, the synthesis of quinoline and its derivatives has been an attractive goal for the organic and medicinal chemists. Several methods for the synthesis of the quinoline nucleus have been reported in the literature, including Skraup, Doebner–von Miller, Friedländer, Pfitzinger, Conrad–Limpach, and Combes [33].
These classical methods are well known and still frequently used for the preparation of pharmaceutically important materials bearing a quinoline backbone. Among them, the Friedländer synthesis is one of the simplest and most frequently used pathways to quinoline derivatives that involve a condensation reaction between an aromatic o-aminoaryl ketone or aldehyde with another carbonyl compound containing an activated α-methylene group followed by cyclodehydration [34,35]. The reaction can be catalyzed by strong acids or bases or may take place without a catalyst at higher temperatures. However, both harsh reaction conditions and the need for a strong acid or base catalyst, which is incompatible with either acid- or base-sensitive groups, reduce the synthetic scope of this reaction. Also, under basic or thermal conditions sometimes ortho-aminobenzophenone does not react with simple ketones or β-keto esters [36]. In general, better yields of quinolines were achieved under acid catalysis [37].
Several Brønsted acid catalysts such as hydrochloric acid in water [38], sulfamic acid [39], sulfuric acid [40], dodecylphosphonic acid [41], p-toluenesulfonic acid, and polyphosphoric acid [42], PEG-supported sulfonic acid [43], propylsulfonic silica [44], o-benzenedisulfonimide [45], oxalic acid [46] and H3PW12O40 [47] have been widely used for this purpose.
Also, modified methods, employing Lewis acids such as Zr(NO3)4 or Zr(HSO4)4 [48], LASC [49], ceric ammonium nitrate [50], GdCl3.6H2O [51], BiCl3 [52], Ag3PW12O40 [53], SnCl2 [54], FeCl3 [55], Mg(ClO4)2 [56], Nd(NO3)3 [57], Y(OTf)3 [58], NiCl2 [59], Bi(OTf)3 [60], ZnCl2 [61], NaAuCl4 [62], NaF [63], NiCl2·2H2O [46] and other effective agents and methods, such as I2/CAN [64], SiO2/I2 [65], NaHSO4–SiO2 [66], PMA·SiO2 [67], Amberlyst 15 [68], microwave [69], urea [70], KOtBu [71], sodium ethoxide (10 mol %) [72], nanosized MCM-41 [73], nanocrystalline aluminium oxide [74] and Nano-Flake ZnO [75] have been reported for this reaction. However, most of these methods suffer from many disadvantages such as harsh reaction conditions, use of harmful organic solvents, high reaction temperatures, prolonged reaction times, low yields, difficulties in the work-up, use of relatively expensive reagents, low selectivity and tedious experimental procedure. In several cases the recovery of the catalyst is also a problem. Therefore, development of simple, convenient, and environmentally benign methods for this synthesis is still required.
In recent years, ionic liquids (ILs) have gained much attention as “designer solvents” for a variety of chemical applications. These compounds are composed of ions and are liquid at or close to room temperature. ILs have become increasingly popular as reaction and extraction media in research and development. These compounds have also widely been used as powerful alternatives to the volatile organic compounds in the field of organic synthesis. In addition, the synthesis of task-specific ionic liquids (TSILs), which have a functional group in their framework, may expand the application of ILs in organic chemistry [76–78].
There have been many researches referring to some aspects of TSILs in physical chemistry, electrochemistry, designability of structure and catalytic ability [79–95]. They have received attention as eco-friendly and alternative reaction media in organic synthesis because of their unique properties, such as high chemical and thermal stability, cost effectiveness, air and water compatibility, negligible vapor pressure, non-miscibility, non-flammability, good reactivity and excellent electrical conductivity and ease of handling [96,97]. For this reason, recently, the field of TSILs is growing at a very fast rate, as the many beneficial properties of these liquids are identified and utilized. Among them, Brønsted acidic ILs had been identified as more effective and environmentally friendly catalysts and could catalyze those organic unit reactions which were promoted by the stronger acids [98].
Introduction of Brønsted acidic functional groups into cations or anions of ILs, especially SO3H and SO4H functional groups, obviously enhances their acidities and water solubility [99,100]. Moreover, their polar nature makes them useful for use under solvent-free conditions. Recently, sulfonic acid-functionalized ionic liquids received great attention due to their interesting properties like environmental compatibility, reusability, greater selectivity, and ease of isolation. They are used as dual solvent-catalyst [101], for several organic reactions, such as esterification [102], alkylation [103], nitration of aromatic compounds [104], hydrolysis [105] and heterocyclic synthesis, like Fisher indole synthesis [106]. Imidazolium ILs having a Brønsted acidic group such as DSIMHS [107,108] are important and they have been successfully used as catalyst in organic synthesis. According to the above-mentioned considerations and our interest in ILs chemistry, we have decided to develop an efficient and mild method for the synthesis of quinoline and its derivatives using the Friedländer annulation method in the presence of DSIMHS as an efficient catalyst, under solvent-free conditions.
2 Experimental
2.1 General
Chemicals were purchased from Fluka and Merck Chemical Companies and used without further purification. All yields refer to isolated products and Progress of the reactions was monitored by TLC using silica gel polygrams SIL G/UV 254 plates. The products were characterized by comparison of their spectral (IR, 1H NMR and 13C NMR) and physical data with those of authentic samples.
2.2 Instrumentation
Melting points were recorded on an electrothermal digital melting point apparatus model IA9100 in open capillary tubes. IR spectra were recorded using a PerkinElmer model Spectrum One FT-IR Spectrometer. The 1H NMR spectra were obtained on a Bruker DRX-400 Avance spectrometer and 13C NMRs were recorded on a Bruker DRX-100 Avance spectrometer (δ in ppm).
2.3 General procedure for the synthesis of quinoline derivatives in the presence of DSIMHS
A mixture of 2-aminoaryl ketone (1 mmol), α-methylene carbonyl compound (1.5 mmol) and DSIMHS (0.25 mmol) was heated in an oil bath at 70 °C under stirring for the appropriate time mentioned in Table 3. After completion of the reaction as monitored by TLC, the reaction mixture was washed with water, because DSIMHS is soluble in water and the product was precipitated with high purity. Then the crude solid product was filtered off and recrystallized from ethanol.
Synthesis of quinolines in the presence of DSIMHS under solvent-free conditions at 70 °Ca.
Entry | 2-Aminoaryl ketone | Ketone | Quinoline | Time (min) | Yield (%)b | M.P. (°C) | Ref. | |
Found | Lit. | |||||||
1 | 5 | 94 | 99–100 | 100–101 | [46] | |||
2 | 12 | 93 | 99–100 | 98–100 | [109] | |||
3 | 15 | 92 | 109–110 | 111–112 | [46] | |||
4 | 15 | 90 | 129–131 | 130–132 | [53] | |||
5 | 5 | 94 | 153–154 | 156–157 | [53] | |||
6 | 10 | 92 | 155–157 | 155–156 | [53] | |||
7 | 25 | 89 | 192–194 | 190–192 | [53] | |||
8 | 20 | 92 | 100–101 | 102–104 | [41] | |||
9 | 45 | 94 | 132–134 | 133–135 | [46] | |||
10 | 35 | 91 | 150–152 | 150–151 | [53] | |||
11 | 25 | 88 | 106–108 | 106–107 | [46] | |||
12 | 30 | 89 | 164–166 | 164–165 | [53] | |||
13 | 20 | 89 | 186–187 | 185–186 | [53] | |||
14 | 40 | 90 | 207–209 | 208–209 | [53] | |||
15 | 20 | 88 | 240–243 | This work | – |
a Products were characterized by their physical constants, comparison with authentic samples, IR and NMR spectroscopy.
b Isolated yield.
2.4 The spectral and analytical data for new compound are given below
8-chloro-10-phenyl-11H-indeno[1,2-b] quinolin-11-one (Table 3, entry 15).
MP 240–243 °C; IR (KBr) νmax/cm−1: 3046, 2917, 2850, 1717, 1612, 1572, 1504, 1443; 1H NMR (400 MHz, CDCl3): d (ppm) 7.43–7.46 (m, 2H), 7.51–7.55 (td, J1 7.60 Hz, J2 0.80 Hz, 1H), 7.60–7.634 (m, 3H), 7.66–7.67 (d, J 2.00 Hz, 1H), 7.69–7.70 (d, J 2.40 Hz, 1H), 7.72–7.75 (m, 1H), 8.10–8.15 (t, J 9.80 Hz, 2H); 13C NMR (100 MHz, CDCl3): d (ppm) 121.7, 123.3, 124.0, 127.4, 128.4, 128.7, 129.3, 129.4, 131.3, 131.8, 132.4, 133.1, 135.5, 137.5, 143.1, 147.1, 148.8, 162.2, 189.9.
3 Results and discussion
The reaction of 2-aminobenzophenon and ethyl acetoacetate (EAA) was chosen as a model for finding the optimization conditions and to achieve good yield of the desired products. In order to investigate the effect of temperature on the reaction, the concentration of ionic liquid was kept constant at 0.25 mmol and a mixture of substrates was treated with DSIMHS. The reaction was monitored by TLC at different temperatures ranging from 25 to 80 °C under solvent-free conditions (Table 1).
Temperature optimization for the synthesis of ethyl 2-methyl-4-phenylquinoline-3-carboxylatea.
Entry | Temperature (°C) | Time (min) | Yield (%) |
1 | 25 | 300 | 30 |
2 | 60 | 30 | 56 |
3 | 70 | 5 | 94 |
4 | 80 | 5 | 96 |
a Reaction condition: 2-aminobenzophenon (1 mmol), EAA (1.5 mmol) and DSIMHS (0.25 mmol) at different temperatures under solvent-free conditions.
Our results indicate that after stirring the reaction mixture at room temperature for 300 min under solvent-free conditions, the yield of the corresponding product was low (Table 1, entry 1). Subsequently, the mixture was heated in an oil bath. Increasing the reaction temperature from 60 to 70 °C gave better results in terms of yield and reaction time. Further increase in temperature did not show any significant improvement in the yield and the reaction time. Therefore, 70 °C was selected as the reaction temperature for all further reactions.
Then, we changed the catalyst amount and examine the effect of this variation on the reaction. According to the results presented in Table 2, in the absence of DSIMHS, the reaction did not produce any product, even after 3 h, which showed that the catalyst exhibits a high catalytic activity in this transformation (Table 2, entry 1). So, the best result was obtained using 0.25 mmol of DSIMHS for this reaction. Higher amounts of the catalyst improved neither the yield nor the reaction time further.
Temperature optimization for the synthesis of ethyl 2-methyl-4-phenylquinoline-3-carboxylatea.
Entry | Catalyst amount (mmol) | Time (min) | Yield (%) |
1 | – | 180 | – |
2 | 0.15 | 30 | 76 |
3 | 0.25 | 5 | 94 |
4 | 0.35 | 5 | 96 |
a Reaction condition: 2-aminobenzophenon (1 mmol), EAA (1.5 mmol) and different amounts of DSIMHS at 70 °C under solvent-free conditions.
On the basis of the above-mentioned studies, we concluded that the best results can be obtained when the relative ratio of the substrate/EAA/DSIMHS was 1.0 mmol/1.5 mmol/0.25 mmol, respectively, while the reaction is carried out at 70 °C under solvent-free conditions. Under the optimized conditions, the model reaction gave a 94% yield of ethyl 2-methyl-4-phenylquinoline-3-carboxylate after 5 min (Table 2, entry 3 and Scheme 1).

Reaction of 2-aminobenzophenone and EAA under solvent-free condition at 70 °C in the presence of DSIMHS.
Thereafter, to show the generality of this method, a series of ortho-aminoaryl ketones were reacted with various 1, 3-dicarbonyl compounds under the optimized conditions and the results are summarized in Table 3.
As shown in Table 3, this method is equally effective for cyclic ketones, both cyclic and acyclic diketones and β-ketoesters. These types of compounds are reacted smoothly with 2-aminobenzophenone and 2-amino-5-chlorobenzophenone to produce a range of quinoline derivatives. Complete conversion and good-to-excellent isolated yields were observed for all the substrates employed. This reaction is very effective in avoiding the use of strong mineral acids, high temperatures, and toxic and polluting reactants.
All synthesized products are known compounds and were characterized by comparing their melting points, IR, 1H and 13C NMR spectra, with those of authentic samples.
The reusability and recyclability of DSIMHS was also examined in the reaction of 2-aminobenzophenon and EAA at 70 °C under solvent-free conditions. For this purpose, the catalyst was easily recovered from the reaction mixture by a simple extraction with water. Then the recovered catalyst was dried and reused at least for four times without considerable loss of its activity (Fig. 1).
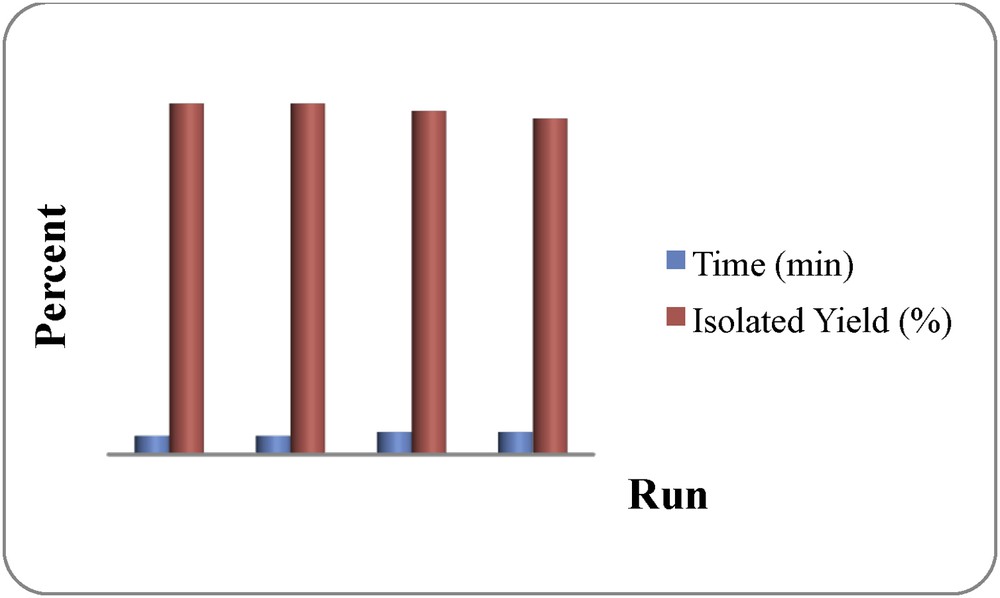
Reusability of DSIMHS in the synthesis of ethyl 2-methyl-4-phenylquinoline-3-carboxylate (Table 3, entry 1).
Furthermore, in order to show the excellent catalytic activity of DSIMHS and the efficiency of this procedure, we have compared some of the results obtained by our method with some of those reported in the literature, as shown in Table 4; the obtained results indicate the superiority of the present method in terms of yields and/or reaction times.
Comparison of some of the results obtained by the Friedländer reaction with 2-aminobenzophenone in the presence of DSIMHS (1), (BSPY)HSO4/MCM-41 (2) [73], Yb(OTf)3 (3) [110] and dodecylphosphonic acid (4) [41].
Entry | Substrate | Time (min)/Yield (%) | |||
1 | 2 | 3 | 4 | ||
1 | 5/94 | 70/93 | 60/80 | 30/90 | |
2 | 5/92 | 90/87 | – | 43/95 | |
3 | 10/90 | 40/87 | 120/80 | 16/95 | |
4 | 15/91 | 45/91 | 120/90 | 45/90 |
There are two possible reaction mechanisms that are shown in Scheme 2. The carbonyl group is activated by DSIMHS for a crossed-aldol reaction, creating an amino ketone (I and/or II). This intermediate subsequently condenses with itself, and produces the ring with concomitant formation of the carbon–nitrogen double bond.

Proposed reaction mechanism.
4 Conclusions
In conclusion, we have successfully demonstrated a green approach for the synthesis of quinoline derivatives via Friedlander annulation using DSIMHS as an efficient, reusable and environmentally acceptable catalyst under solvent-free conditions. The major advantages of this procedure include fast reaction times, moderate reaction conditions, high conversions, ease of preparation and separation of the catalyst, excellent yields, and avoidance of the usage of environmentally unfavorable organic solvents and toxic catalysts. Also, the catalyst was successfully recovered and recycled at least for four runs without significant loss in its activity, which proves that this method is much more convenient than those with conventional catalysts.
Further studies to develop other new uses of DSIMHS in other organic transformations are now in progress in our laboratory.
Acknowledgments
We are thankful to the University of Guilan Research Council for the partial support of this work. F. Shirini and K. Mohammadi wish to thank the Sobhandarou Pharmaceutical Company for the supply of some of the facilities in this work.