1 Introduction
Quinazoline heterocycles are very well-known six-membered heterocyclic ring molecules that possess wide biological properties, such as antitumor, antidefibrillatory, analgesic, diuretic, antihistaminic, vasodilating, tranquilizing and antianxiety ones (Fig. 1) [1–9]. Also quinazolines are oxidized into quinazolin-4(3H)-ones moieties that are, as growth inhibitors, of great importance in the treatment of leukemia cells, and are also used as poly(ADP-ribose)polymerase-1 inhibitors [10,11]. Also quinazoline derivatives were found to be useful as fungicides, bactericides, insecticides, and plant-growth regulators [12]. Some of them display good pharmacological properties, such as sedative, anticholinesterase, hypotensive, soporific, antispasmodic, tranquilizing, muscle relaxing, antirheumatic, diuretic, antimalarial ones, as well as other activities [13,14].
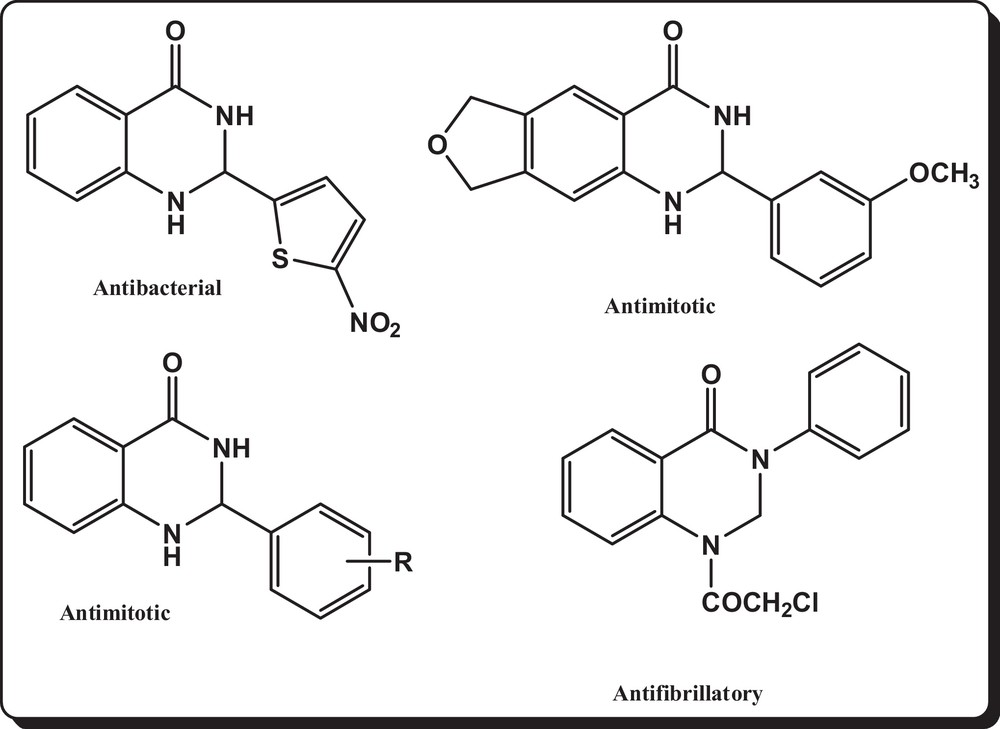
Reported biologically active quinazolinone and quinazolinone-based molecules.
Several methods have been reported for the synthesis of 2,3-dihydroquinazolin-4(1H)-one. Among them, the general method includes the reductive cyclization of aldehydes or ketones with 2-aminobenzamide in the presence of acid catalysts, such as cyanuric chloride, ZrCl4, cerium ammonium nitrate, PPA–SiO2, and gallium triflate [15–19]. Very recently, Ramesh et al. reported efficient methodologies for the synthesis of 2,3-dihydroquinazolin-4(1H)-ones using the three-component aniline/isatoic anhydride/aldehyde system and also the two-component aldehyde/anthranilamide system catalyzed by β-cyclodextrin as a reusable catalyst [20,21]. Also there are some methodologies that were reported recently using two-component or three-component systems in aqueous solutions, ionic liquids, and organic media at high temperature [22–26]. Also Wang et al. reported a manual grinding technique using CAN catalyst, which has some limitations, such as long reaction times, high temperatures, and, practically, the fact that the yield and time needed for manual grinding is variable because of grinding inconsistency [17].
Despite most of the reported protocols give good yields, there are some limitations associated with the reaction system, such as prolonged reaction times, high temperatures and the need for high catalyst loading. Therefore, the development of an efficient, cost effective and high-yield protocol for the synthesis of 2,3-dihydroquinazolin-4(1H)-ones is of great interest, with significant demand from the medicinal industries.
We decided to investigate the efficiency of the supported metal Lewis acid catalyst for the synthesis of 2,3-dihydroquinazolin-4(1H)-ones. With this aim, we synthesized a variety of silica-supported heterogeneous catalysts for this one-pot synthesis, and we established an efficient methodology for the cascade synthesis of 2,3-dihydroquinazolin-4(1H)-ones from aldehydes and 2-aminobenamide/2-aminobenzanilide using CAN·SiO2 as an expeditious catalyst at ambient temperature, with good yields (Scheme 1).
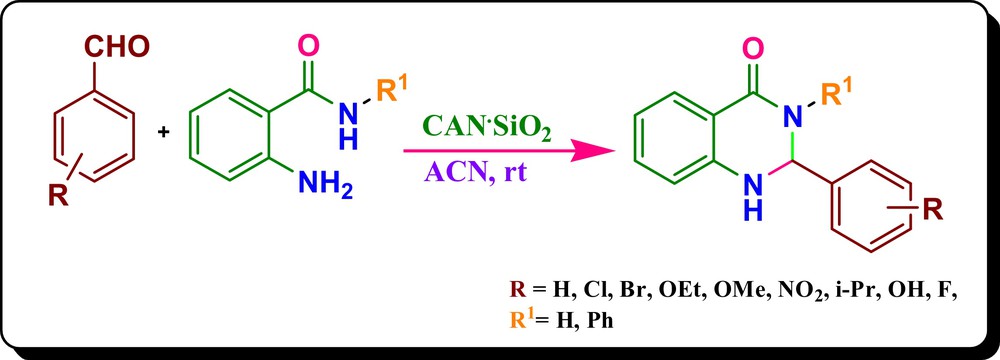
(Color online.) Synthesis of 2,3-dihydroquinazolin-4(1H)-ones.
Cerium (IV) ammonium nitrate (CAN) is a versatile catalyst, which has been widely used in organic transformations due to its many advantages, such as high reactivity, ease of storage, low cost and commercial availability. The use of this reagent for numerous transformations involving C–C, C–O, C–N, and C–S bond formation has been described [27–29]. However, the application of CAN (ceric ammonium nitrate) is limited due to its poor solubility in common organic solvents. Therefore, adopting silica as the supporting material has been reported as a good alternative [30–33]. Silica gel-supported ceric ammonium nitrate (CAN·SiO2) proximates the reactant, which fastens electron-transfer processes between reactants, and further enhances the rate of reaction with higher efficiency and thus with shorter reaction times. Moreover, the use of a heterogeneous metal Lewis catalyst instead of traditional homogeneous metal Lewis and Brønsted acid catalysts could be a more environmental friendly alternative.
In connection with our ongoing research on cost effective methodologies, we discovered a new reaction system for the synthesis of a variety of organic compounds of therapeutic and industrial significance, which are key intermediates for multistep synthesis. In addition, we have implemented a number of green strategies in organic transformations using environmental friendly catalytic reaction conditions [34–38]. Herein, we carried out the development of an efficient practical methodology for the one-pot synthesis of 2,3-dihydroquinazolin-4(1H)-ones by the reductive condensation of 2-aminobenzamide/2-aminobenzanilide and benzaldehyde using various supported catalysts. The best results were obtained when using CAN·SiO2 (containing 5 mol% CAN) at room temperature.
2 Results and discussion
The optimization of the reaction conditions begins with the investigation of the effect of various prepared heterogeneous catalysts and solvents (Table 1). We first carried out a model reaction between 2-aminobenzamide (5 mmol) and benzaldehyde (5 mmol) in the presence of 5 mol% of catalyst using various solvents at room temperature in the air. Also a test reaction was performed without catalyst to find out the actual role of the catalyst in this cascade synthesis. We observed that less than 40% of the product was obtained in the absence of catalyst, even after 2 h (Table 1, entry 12). In search of an effective, catalytic system for the synthesis of quinazolin-4(1H)-ones, the test reaction was performed using benzaldehyde (0.5 mmol), 2-aminobenzamide (0.5 mmol) with different supported metal acid catalysts, such as Cu(OTf)2·SiO2, Zn(OTf)2·SiO2, TiO2·SiO2, CAN·SiO2 and ZnCl2·SiO2 (Table 1). Among all screened catalysts, CAN·SiO2 (containing 5 mol% CAN) at room temperature gave the best yield in short reaction time (Table 1, entry 3). In contrast, TiO2·SiO2, Zn(OTf)2·SiO2, and ZnCl2·SiO2 did not afford the desired product in good yields. The careful analysis of the screened supported Lewis acid catalysts shows that silica-supported metal catalysts such as CAN·SiO2 and Cu(OTf)2·SiO2 were more effective than other silica-supported metal Lewis acid catalysts, but promising results were obtained with the silica-supported ceric ammonium nitrate catalyst with shorter times and better yields.
Optimization of reaction condition using various solvent and catalyst.
Entry | Catalysta (5 mol%) | Solvent | Time | Yieldc |
1 | Cu(OTf)2·SiO2 | Toluene | 60 min | 82 |
2 | Zn(OTf)2·SiO2 | Toluene | 120 min | 68 |
3 | CAN·SiO2 | Toluene | 60 min | 84 |
4 | ZnCl2·SiO2 | Toluene | 120 min | 67 |
5 | TiO2·SiO2 | Toluene | 120 min | 58 |
6 | CAN·SiO2 | DCM | 60 min | 65 |
7 | CAN·SiO2 | ACN | 20 min | 88 |
8 | CAN·SiO2 | Water | 180 min | 85 |
9 | CAN·SiO2 | Ethanol | 60 min | 68 |
10 | CAN·SiO2 | CHCl3 | 60 min | 61 |
11 | CAN·SiO2 | Neat | 60 min | No reaction |
12 | – | ACN | 12 h | < 40 |
13b | CAN·SiO2 | ACN | 60 min | ≤ 90 |
a Reaction conditions: all reactions were carried out at room temperature using benzaldehyde (0.5 mmol), 2-aminobenzamide (0.5 mmol) and 5 mol% of catalyst.
b CAN·SiO2 containing 10 mol% CAN.
c Isolated yield.
Once we had found CAN·SiO2 as the best catalyst for this reaction in toluene as a solvent, the solvent optimization was carried out by screening various solvents, such as EtOH, CH3CN, DCM, chloroform, and water at room temperature. Among all the screened solvents, it was found that acetonitrile is the most suitable one for this reaction: it gives a maximum yield in short times with an easy work-up procedure. It is worthy to note that when we performed the reaction in aqueous media (warm conditions), the reaction was completed with a moderate yield, but took a long time as compared to acetonitrile media. After optimization of the solvent system, the optimization of the appropriate catalyst loading was carried out.
In an attempt to optimize the catalyst, a model reaction was carried out in the absence of catalyst and with 10 mol% of ceric ammonium nitrate supported on silica at room temperature in acetonitrile (Table 1, entries 12, 13). It was found that in the absence of catalyst, less than 40% of yield was observed even after 12 h of stirring of the reaction medium at room temperature. A larger amount of catalyst loading (10 mol%) neither increases the yield nor shortens the conversion time (Table 1, entry 13). So, an amount of 5 mol% of CAN loaded on silica was found to be the optimal quantity sufficient to convert the reactant into the product. Furthermore, CAN·SiO2 was recovered simply by dissolving the reaction mixture in acetone and filtering it to get a solid heterogeneous catalyst with excellent yields (about ≥ 92%). The crude product was obtained by evaporating acetone, and was further purified by recrystallization from ethanol.
The reusability of the catalyst was tested for two more reaction cycles. The recovered catalyst was reused as in other two consecutive reaction cycles accordingly. After the first fresh run with 88% yield, the recovered catalyst was washed, dried, and used for a second and a third times. Yields of 86 and 78% were obtained respectively, thus proving the lesser leaching of the catalyst in the solvent and the catalyst's reusability (Table 2).
Reuse of CAN·SiO2 in the synthesis of 2,3-dihydro-2-phenylquinazolin-4(1H)-one.
Entry | Reaction cycle | Yield (%)a |
1 | Ist (“fresh” run) | 88 |
2 | IInd cycle | 86 |
3 | IIIrd cycle | 78 |
a Isolated yield.
Once the effective catalytic amount of the CAN·SiO2 catalyst (containing 0.05 equiv. of CAN) was proven, we extended, to further generalize this, this methodology to the synthesis of a variety of 2-aryl-2,3-dihydroquinazolin-4(1H)-ones/2,3-diaryl-2,3-dihydroquinazolin-4(1H)-ones derivatives using 2-aminobenzamide/2-aminobenzanilide and various electron-donating moieties, such as methoxy, ethoxy, and isopropyl, as well as electron-withdrawing compounds, such as nitro-, bromo- and chloro-substituted aromatic aldehydes (Table 3). Various aromatic aldehydes with different substituent at ortho, meta or para-positions show equal ease towards the product formation in high yields (78–94% yields, Table 3). In contrast, aromatic aldehydes having groups like Cl, F, Br, MeO, EtO, and nitro showed better reactivity, and the reactions were completed in shorter times. Particularly, the reaction with 2-aminobenzanilide afforded the desired product in moderate yields. The overall study made us conclude that CAN·SiO2 is the best catalytic system for this cascade synthesis, as it gives excellent yields in very short durations in the milder conditions.
Entry | Aldehyde | Product | Time (min) | Yield (%)b | Reportedc |
1 | C6H5 | 20 | 88 | 21 | |
2 | 4-Cl–C6H4 | 10 | 86 | 23 | |
3 | 4-Br–C6H4 | 20 | 84 | 21 | |
4 | 4-OEt–C6H4 | 40 | 94 | – | |
5 | 2-OMe–C6H4 | 20 | 89 | 21 | |
6 | 4-OMe–C6H4 | 15 | 92 | 22 | |
7 | 3-OMe–C6H4 | 40 | 86 | 3 | |
8 | 4-NO2–C6H4 | 10 | 92 | 21 | |
9 | 4-iPr–C6H4 | 10 | 90 | 15 | |
10 | 4-OH–C6H4 | 25 | 83 | 21 | |
11 | 2-F–C6H4 | 30 | 90 | 15 | |
12 | 3-F–C6H4 | 20 | 88 | 23 | |
13 | C6H5 | 40 | 84 | 24 | |
14 | 4-Br–C6H4 | 30 | 87 | 25 | |
15 | 4-Cl–C6H4 | 30 | 92 | 24 | |
16 | 2-F–C6H4 | 50 | 78 | 26 |
a All reactions were carried out at room temperature using benzaldehyde (0.5 mmol), 2-aminobenzamide (0.5 mmol) and CAN·SiO2 (containing 5 mol% CAN) in acetonitrile at room temperature.
b Isolated yield.
c Compound reported in the literature.
A possible mechanism for this one-pot reaction has been postulated on the basis of the reported literature [15,39]. The reaction proceeds with the formation of imine with the amine of 2-aminobenzamide/2-aminobenzanilide and benzaldehydes promoted by CAN·SiO2. Intermediate 1 could be tautomerized in the presence of CAN·SiO2 to give intermediate 2. The formed intermediate 2 could be activated by CAN·SiO2, which will be further converted into intermediate 3 by intramolecular nucleophile attack of the nitrogen on the imine carbon. Finally, we obtained the desired product 2,3-dihydroquinazolin-4(1H)-ones by a simple 1,5-proton transfer (Scheme 2). All new compounds were completely characterized by their spectral properties, as 1H-, 13C-NMR and mass analysis were carried out.

(Color online.) A possible mechanism for the synthesis of 2,3-dihydroquinazolin-4(1H)-ones.
3 Conclusion
In conclusion, we have successfully developed an efficient protocol for the synthesis of 2,3-dihydroquinazolin-4(1H)-ones using CAN·SiO2 as an expeditious reusable catalyst at room temperature. Compared to the previously known methodologies, silica-supported CAN was conveniently prepared without any special precaution. A wide range of products bearing different functionalized groups is conveniently accessible in reasonable to excellent isolated yields. The method offers several advantages, including room-temperature conditions, high yield of products, short reaction times, recyclability of the catalyst, and the fact that the residue is crystallized from ethanol to give the pure product without further column purification.
4 Experimental
4.1 General
All chemicals were purchased from Aldrich and Alfa Aesar Chemicals. NMR spectra were recorded in ppm in DMSO-d6 on a Jeol JNM ECP 400 NMR instrument using TMS as an internal standard. EI/MS were recorded on a Jeol JMS-700 mass spectrometer. The melting points were taken in open capillaries and are uncorrected; an Electrothermal-9100 (Japan) instrument was used to determine the melting point of the compounds.
4.2 General procedure for the synthesis of the silica-supported ceric ammonium nitrate catalyst
A supported ceric ammonium nitrate catalyst was prepared by adopting the procedure in the literature [30]. Neutral silica gel (9.01 g, Merck Kieselgel 60, particle size 0.063–0.200 mm, 70–230 mesh) was mixed with a solution of CAN (1.02 g) in water (2.0 mL). Evaporation of water under reduced pressure gave a dry yellowish powder, which contained 10% (by weight) of CAN. According to Hwu et al., this reagent was found active for at least six months of storage in a well-capped bottle.
4.3 General experimental procedure for the synthesis of 2,3-dihydroquinazolin-4(1H)-ones using CAN·SiO2
The standard procedure was followed by the use of 1 (3.6 mol), 2 (3.6 mol), CAN·SiO2 0.401 g (containing 0.100 g of CAN, 0.18 mol, 0.05 equiv.), and acetonitrile (5.0 mL). After the reaction mixture had been stirred for certain period as indicated in Table 3, and completion of the reaction as indicated by TLC, the reaction mixture was dissolved in acetone and the catalyst was recovered by filtration. The solvent was then evaporated under vacuum to afford the crude product. The obtained crude product was further recrystallized from ethanol to get the pure final product. All new compounds were completely characterized by 1H/13C NMR and EI/MS data of few selected new compounds are given below. The scanned spectra of all compounds are provided as supporting information (Appendix B).
4.3.1 2,3-Dihydro-2-phenylquinazolin-4(1H)-one (1, C14H12N2O)
Rf = 0.29 (hexane/ethyl acetate 70:30%), Pale yellow solid; Mp. 220–222 °C; yield 88%. 1H NMR (400 MHz, DMSO-d6) δ: 8.32 (br s, 1H), 7.65 (d, J = 7.6 Hz, 1H), 7.52 (d, J = 7.3 Hz, 2H), 7.41–7.33 (m, 3H), 7.26 (t, J = 7.5 Hz, 1H), 7.14 (br s, 1H), 6.78 (d, J = 8.0 Hz, 1H), 6.69 (t, J = 7.5 Hz, 1H), 5.79 (s, 1H) ppm; 13C NMR (100.5 MHz, DMSO-d6) δC: 163.6, 147.8, 141.6, 133.3, 128.4, 128.3, 127.3, 126.8, 117.1, 114.9, 114.4, 66.6 ppm.
4.3.2 2-(4-Chlorophenyl)-2,3-dihydroquinazolin-4(1H)-one (2, C14H11ClN2O)
Rf = 0.38 (hexane/ethyl acetate 70:30%), pale yellow solid; Mp. 203–205 °C; yield 86%. 1H NMR (400 MHz, DMSO-d6) δ: 8.35 (br s, 1H), 7.63 (d, J = 7.6 Hz, 1H), 7.49 (dd, J = 8.4, 26 Hz, 4H), 7.25 (t, J = 7.6 Hz, 1H), 7.15 (br s, 1H), 6.76 (d, J = 8.4 Hz, 1H), 6.69 (t, J = 7.5 Hz, 1H), 5.79 (s, 1H) ppm; 13C NMR (100.5 MHz, DMSO-d6) δC: 163.5, 147.6, 140.6, 133.3, 132.9, 128.7, 128.3, 127.3, 117.2, 114.9, 114.4, 65.7 ppm.
4.3.3 2-(4-Bromophenyl)-2,3-dihydroquinazolin-4(1H)-one (3, C14H11BrN2O)
Rf = 0.36 (hexane/ethyl acetate 70:30%), pale green solid; Mp. 193–195 °C; yield 84%. 1H NMR (400 MHz, DMSO-d6) δ: 8.35 (br s, 1H), 7.63–7.58 (m, 3H), 7.45 (d, J = 8.4 Hz, 2H), 7.25 (t, J = 7.7 Hz, 1H), 7.16 (br s, 1H), 6.76 (d, J = 8.0 Hz, 1H), 6.69 (t, J = 7.5 Hz, 1H), 5.77 (s, 1H) ppm; 13C NMR (100.5 MHz, DMSO-d6) δC: 163.4, 147.6, 141.1, 133.4, 131.2, 129.0, 127.3, 121.5, 117.2, 114.9, 114.4, 65.7 ppm.
4.3.4 2-(4-Ethoxyphenyl)-2,3-dihydroquinazolin-4(1H)-one (4, C16H16N2O2)
Rf = 0.38 (hexane/ethyl acetate 70:30%), yellow solid; Mp. 122–124 °C; yield 94%; EIMS m/z 268 [M]+. 1H NMR (400 MHz, DMSO-d6) δ: 8.17 (br s, 1H), 7.93 (br s, 1H), 7.39 (d, J = 8.4 Hz, 1H), 7.25–7.21 (m, 3H), 6.91 (d, J = 11.6 Hz, 1H), 6.74 (d, J = 8.0 Hz, 1H), 6.68–6.60 (m, 2H), 5.70 (s, 1H), 4.03–3.79 (q, 2H), 1.30 (t, J = 6.9, 3H) ppm; 13C NMR (100.5 MHz, DMSO-d6) δC: 163.0, 147.0, 133.1, 128.1, 127.3, 127.1, 116.4, 114.4, 114.2, 114.0, 66.8, 63.0, 14.6 ppm.
4.3.5 2,3-Dihydro-2-(2-methoxyphenyl)quinazolin-4(1H)-one (5, C15H14N2O2)
Rf = 0.35 (hexane/ethyl acetate 70:30%), yellow solid; Mp. 123–125 °C; yield 89%. 1H NMR (400 MHz, DMSO-d6) δ: 8.04 (br s, 1H), 7.65 (d, J = 7.3 Hz, 1H), 7.42 (d, J = 7.3 Hz, 1H), 7.33–7.19 (m, 3H), 7.03 (t, J = 8.0 Hz, 1H), 6.94 (t, J = 7.5, 1H), 6.79 (d, J = 8.0 Hz, 1H), 6.67 (t, J = 7.5 Hz, 1H), 6.05 (s, 1H), 3.82 (s, 3H) ppm; 13C NMR (100.5 MHz, DMSO-d6) δC: 163.8, 156.3, 147.9, 133.2, 129.6, 128.9, 126.8, 120.1, 117.0, 114.5, 111.0, 61.0, 55.51 ppm.
4.3.6 2,3-Dihydro-2-(4-methoxyphenyl)quinazolin-4(1H)-one (6, C15H14N2O2)
Rf = 0.38 (hexane/ethyl acetate 70:30%), pale yellow solid; Mp178–180 °C; yield 92%. 1H NMR (400 MHz, DMSO-d6) δ: 8.19 (s, 1H), 7.62 (d, J = 8.0 Hz, 1H), 7.42 (d, J = 8.8 Hz, 2H), 7.24 (br s, 1H), 6.94 (d, J = 8.8 Hz, 3H), 6.75 (d, J = 8.01 Hz, 1H), 6.67 (t, J = 7.5 Hz, 1H), 5.71 (s, 1H), 3.74 (s, 3H) ppm; 13C NMR (100.5 MHz, DMSO-d6) δC: 163.7, 159.4, 148.0, 133.2, 128.2, 127.3, 117.0, 114.9, 114.4, 113.6, 66.3, 55.1 ppm.
4.3.7 2,3-Dihydro-2-(3-methoxyphenyl)quinazolin-4(1H)-one (7, C15H14N2O2)
Rf = 0.28 (hexane/ethyl acetate 70:30%), yellow solid; Mp. 142–144 °C; yield 86%. 1H NMR (400 MHz, DMSO-d6) δ: 8.30 (s, 1H), 7.62 (unresolved dd, 1H), 7.30–7.22 (m, 2H), 7.12 (br s, 1H), 7.07–7.06 (m, 2H), 6.90 (unresolved dd, 1H), 6.76 (d, J = 8.0 Hz, 1H), 6.67 (t, J = 7.5 Hz, 1H), 5.73 (d, J = 1.8 Hz, 1H), 3.74 (s, 3H) ppm; 13C NMR (100.5 MHz, DMSO-d6) δC: 163.5, 159.2, 147.7, 143.3, 133.2, 129.4, 127.3, 118.9, 117.0, 114.3, 113.6, 112.5, 66.2, 55.0 ppm.
4.3.8 2,3-Dihydro-2-(4-nitrophenyl)quinazolin-4(1H)-one (8, C14H11N3O3)
Rf = 0.27 (hexane/ethyl acetate 70:30%), yellow solid; Mp. 183–185 °C; yield 92%. 1H NMR (400 MHz, DMSO-d6) δ: 8.53 (br s, 1H), 8.24 (d, J = 9.6 Hz, 2H), 7.75 (d, J = 8.8 Hz, 2H), 7.62 (dd, J = 1.4, 8.0 Hz, 1H), 7.33 (br s, 1H), 7.28–7.24 (m, 1H), 6.78 (d, J = 8.0 Hz, 1H), 6.70–6.66 (m, 1H), 5.92 (s, 1H) ppm; 13C NMR (100.5 MHz, DMSO-d6) δC: 163.3, 149.3, 147.2, 133.5, 128.0, 127.4, 123.5, 117.4, 114.9, 114.5, 65.3 ppm.
4.3.9 2,3-Dihydro-2-(4-isopropylphenyl)quinazolin-4(1H)-one (9, C17H18N2O)
Rf = 0.29 (hexane/ethyl acetate 70:30%), pale yellow solid; Mp. 159–161 °C; yield 90%. 1H NMR (400 MHz, DMSO-d6) δ: 8.21 (br s, 1H), 7.61 (d, J = 7.6 Hz, 1H), 7.42 (d, J = 8.4 Hz, 2H), 7.27-7.21 (m, 3H), 7.02 (br s, 1H), 6.73 (d, J = 8.0 Hz, 1H), 6.67 (t, J = 7.7 Hz, 1H), 5.71 (s, 1H), 2.93–2.83 (m, 1H), 1.18 (d, J = 6.9 Hz, 6H) ppm; 13C NMR (100.5 MHz, DMSO-d6) δC: 163.6, 148.8, 147.9, 138.9, 133.2, 127.3, 126.9, 126.2, 117.0, 114.9, 114.3, 66.5, 33.2, 23.8 ppm.
4.3.10 2,3-Dihydro-2-(4-hydroxyphenyl)quinazolin-4(1H)-one (10, C14H12N2O2)
Rf = 0.28 (hexane/ethyl acetate 70:30%), yellow solid; Mp. 192–194 °C; yield 83%. 1H NMR (400 MHz, DMSO-d6) δ: 9.54 (br s, 1H), 8.12 (s, 1H), 7.63 (d, J = 7.6 Hz, 1H), 7.32 (d, J = 8.0 Hz, 2H), 7.24 (t, J = 7.5 Hz, 1H), 6.94 (br s, 1H), 6.79–6.74 (m, 3H), 6.58 (t, J = 7.5 Hz, 1H), 5.67 (s, 1H) ppm; 13C NMR (100.5 MHz, DMSO-d6) δC: 163.8, 157.7, 148.1, 133.2, 131.6, 128.3, 127.3, 117.0, 114.9, 114.4, 66.7 ppm.
4.3.11 2-(2-Fluorophenyl)-2,3-dihydroquinazolin-4(1H)-one (11, C14H11FN2O)
Rf = 0.28 (hexane/ethyl acetate 70:30%), pale yellow solid; Mp. 185–187 °C; yield 90%. 1H NMR (400 MHz, DMSO-d6) δ: 8.26 (s, 1H), 7.62 (unresolved dd, 1H), 7.57–7.53 (m, 1H), 7.43–7.38 (m, 1H), 7.27–7.20 (m, 3H), 7.64 (s, 1H), 6.76 (d, J = 8.0 Hz, 1H), 6.72–6.68 (m, 1H), 6.06 (s, 1H) ppm; 13C NMR (100.5 MHz, DMSO-d6) δC: 163.5, 147.6, 133.4, 130.6, 128.3, 127.3, 124.3, 117.3, 115.7, 115.4, 114.6, 114.4, 60.8 ppm.
4.3.12 2-(3-Fluorophenyl)-2,3-dihydroquinazolin-4(1H)-one (12, C14H11FN2O)
Rf = 0.27 (hexane/ethyl acetate 70:30%), pale yellow solid; Mp. 258–260 °C; yield 88%. 1H NMR (400 MHz, DMSO-d6) δ: 8.41 (s, 1H), 7.60 (unresolved dd, 1H), 7.43–7.16 (m, 6H), 6.78 (d, J = 8.0 Hz, 1H), 6.68 (t, J = 7.8 Hz, 1H), 5.80 (s, 1H) ppm; 13C NMR (100.5 MHz, DMSO-d6) δC: 163.4, 147.5, 144.6, 133.4, 130.3, 127.3, 122.7, 117.3, 115.2, 114.9, 114.4, 113.6,113.4, 65.6 ppm.
4.3.13 2,3-Dihydro-2,3-diphenylquinazolin-4(1H)-one (13, C20H16N2O)
Rf = 0.28 (hexane/ethyl acetate 70:30%), blackish green solid; Mp. 215–217 °C; yield 84%. 1H NMR (400 MHz, DMSO-d6) δ: 7.75 (dd, J = 1.4, 7.7 Hz, 1H), 7.66 (d, J = 2.5 Hz, 1H), 7.40–7.16 (m, 11H), 6.78 (d, J = 8.0 Hz, 1H), 6.74–6.70 (m, 1H), 6.29 (d, J = 2.6 Hz, 1H) ppm; 13C NMR (100.5 MHz, DMSO-d6) δC: 162.2, 146.5, 140.6, 133.7, 128.5, 128.3, 128.2, 127.9, 126.5, 126.2, 125.9, 117.4, 114.7, 72.5 ppm.
4.3.14 2-(4-Bromophenyl)-2,3-dihydro-3-phenylquinazolin-4(1H)-one (14, C20H15BrN2O)
Rf = 0.27 (hexane/ethyl acetate 70:30%), pale yellow solid; Mp. 221–223 °C; yield 87%. 1H NMR (400 MHz, DMSO-d6) δ: 7.74 (dd, J = 1.0, 7.7 Hz, 1H), 7.65 (br s, 1H), 7.51 (d, J = 8.4 Hz, 2H), 7.35–7.17 (m, 8H), 6.78–6.71 (m, 2H), 6.31 (s, 1H) ppm; 13C NMR (100.5 MHz, DMSO-d6) δC: 162.1, 146.3, 140.5, 140.0, 133.8, 131.2, 128.8, 128.6, 127.9, 126.1, 126.0, 121.5, 117.6, 114.8, 71.9 ppm.
4.3.15 2-(4-Chlorophenyl)-2,3-dihydro-3-phenylquinazolin-4(1H)-one (15, C20H15ClN2O)
Rf = 0.28 (hexane/ethyl acetate 70:30%), pale pink solid; Mp. 219–221 °C; yield 92%. 1H NMR (400 MHz, DMSO-d6) δ: 7.75 (d, J = 7.7 Hz, 1H), 7.66 (d, J = 2.5 Hz, 1H), 7.41–7.25 (m, 9H), 7.19 (t, J = 7.1 Hz, 1H), 6.78 (d, J = 8.0 Hz, 1H), 6.73 (t, J = 7.7 Hz, 1H), 6.32 (d, J = 2.5 Hz, 1H) ppm; 13C NMR (100.5 MHz, DMSO-d6) δC: 162.1, 146.4, 140.6, 139.6, 133.8, 132.9, 128.6, 128.5, 128.3, 127.9, 126.2, 126.0, 117.6, 114.8, 71.9 ppm.
4.3.16 2-(2-Fluorophenyl)-2,3-dihydro-3-phenylquinazolin-4(1H)-one (16, C20H15FN2O)
Rf = 0.30 (hexane/ethyl acetate 70:30%), blackish green solid; Mp. 195–197 °C; yield 78%. 1H NMR (400 MHz, DMSO-d6) δ: 7.74 (unresolved dd 1H), 7.70 (d, J = 2.56 Hz, 1H) 7.37–7.18 (m, 9H), 7.12–7.07 (m, 1H), 6.79 (d, J = 8.0 Hz, 1H), 6.74 (t, J = 7.8 Hz, 1H), 6.35 (d, J = 2.9 Hz, 1H) ppm; 13C NMR (100.5 MHz, DMSO-d6) δC: 162.1, 146.4, 143.3, 140.6, 133.8, 130.3, 128.6, 128.5, 127.9, 126.1, 122.6, 117.7, 115.3, 115.0, 114.8, 113.6, 114.4, 71.8 ppm.
Acknowledgements
This research work was supported by the Industrial Technology Development Program, which was conducted by the Ministry of Knowledge Economy of the Korean Government.