1 Introduction
The rapid development of nanoscience and the application of nanotechnology have opened new approaches in quick and early diagnosis, timely treatment, and smart drug delivery and real-time assessments of therapeutic and surgical efficacy. Drug delivery is a rapidly growing area that takes advantages of nanotube technology. The systems being used currently for drug delivery include dendrimers [1], polymers [2], and liposomes [3], but carbon nanotubes present the opportunity to work with effective structures that possess high drug loading capacities and good cell penetration qualities [4]. Although biomedical applications of CNTs are not very appealing because of their toxicity and non-uniformity in dispersion in the solution, it has been confirmed experimentally that CNTs are pernicious to the survival of cells [5].
Among the most promising materials for bionanotechnology are boron nitride nanotubes (BNNTs). BNNTs are wide-band gap semiconductors whose electrical properties are independent of geometry, while CNTs may be metallic or semiconducting, depending on chirality and diameter. BNNTs are also more chemically inert, resistant to oxidation and corrosion and structurally stable than CNTs, thus the application of BNNTs is preferred, as far as nanotube-based device applications are expected at high temperatures and in hazardous environments [6–8].
On the other hand, a less reactive channel such as BNNTs may be less damaging to the biological molecules it carries and the cell at which it is targeted. Good biocompatibility [9,10] of BNNTs suggests that they are emerging as new materials for biomedical, therapeutic and diagnostic applications [11–15]. Therefore, it is important to realize the drug delivery properties of BNNTs and also their application in medicine. However, these applications often require a uniform and stable dispersion of BNNTs in solutions. Nanotubes such as CNTs and BNNTs are not soluble in any common solvents as they have a tendency to aggregate. Many applications of BNNTs are hampered due to the fact that pristine BNNTs are strongly hydrophobic and very difficult to disperse in aqueous environments. In the past several years, intensive research has been addressed to overcome the poor solubility of BNNTs in solvents. Non-covalent and covalent functionalization approaches have been used to functionalize and improve the in vitro biocompatibility and the solubility of BNNTs in aqueous and organic solutions [16–26]. For example, in Xie et al.’s work, an aqueous solution of BNNTs is obtained after a functionalization treatment with an amine-terminated oligomeric poly(ethyleneglycol). These reports demonstrate promising possibilities of homogeneous dispersion of BNNTs in various solutions [27]. Ciofani et al. proposed an innovative and simple strategy to obtain chemically functionalized boron nitride nanotubes. A strong oxidation with nitric acid, followed by the silanization of the surface by using APTES (3-aminopropyl-triethoxysilane), allowed amino groups to be chemically linked to the surface of the nanotubes. Ciofani et al. demonstrated the in vitro biocompatibility and cellular uptake of the functionalized BNNTs. This functionalization approach opens important perspectives to the exploitation of BNNTs as smart vectors for several nanomedicine applications [28]. While pristine BNNTs show no solubility in water, the functionalized nanotubes are easily soluble in aqueous solution.
In vitro studies have assessed BNNT–cell interactions in different cell lines, such as human neuroblastoma [12], muscle cells [29], embryonic kidney [30], murine alveolar macrophage and embryonic fibroblast cells [31], also showing contradictory results. Since the effects of nanomaterials vary depending on the cell type, localization and physiological role, it is crucial to assess them in various cell models. Thus, it is of utmost importance to further evaluate the biosafety in vitro and in vivo of BNNTs, particularly in vivo, before intensifying their use, especially in biomedical applications [32].
Nutrient-deficiency diseases are widespread among 2 billion people in developing and in developed countries. These are silent epidemics of vitamin and mineral deficiencies affecting people of both genders and all ages. Vitamin and mineral deficiencies not only cause specific diseases, but also they act as exacerbating factors in infectious and chronic diseases, they greatly influence morbidity, mortality, and quality of life [33].
Vitamins are organic compounds required by cells and organs to maintain their functions and development. They play a role in enzymic processes either as co-enzymes or their precursors and also in genetic regulatory processes. They may also act as antioxidants. Most vitamins cannot be synthesized by the body, thus must be taken through the diet. There are five vitamins associated with pandemic deficiency diseases. These are vitamin A, vitamin B1, vitamin C, vitamin B3, and vitamin D. Globally, vitamin A deficiency is a major public health problem and the most important preventable cause of blindness. Vitamin A has essential functions in vision, bone growth, reproduction, spermatogenesis and maintenance of healthy epithelia tissues [34]. Vitamin B1 plays a major role in the body health as well as in sound mental health. It is an essential part of converting carbohydrates for energy and also it is necessary for the proper functioning of the nervous system, the heart, and the musculature system. A deficiency of B1 in the diet results in the disease known as Beri Beri [35]. Vitamin C is a vital component of the human diet, it is also used for the prevention and treatment of the common cold, mental illnesses, infertility, cancers, and AIDS (acquired immune deficiency syndrome). Vitamin C is required in the process of collagen synthesis. Hydroxylation of lysines and prolines on the collagen propeptide by the enzymes prolyl hydroxylase and lysyl hydroxylase promote peptide crosslinking. This enzymatic step requires ascorbic acid as a cofactor. Reduction of vitamin C intake decreases the hydroxylation of prolines and lysines, and causes a looser triple helix result in scurvy [36,37]. Deficiencies of vitamin B3 result in avitaminose and lead to skin diseases and nervous disorders. Because it is vital for the production of energy, a vitamin B3 deficiency can lead to general fatigue, muscular weakness, and loss of appetite [38]. Vitamin D plays a major biologic function in maintaining the serum calcium and the phosphorous homeostasis. It promotes calcium absorption in the gut and reabsorption from the kidneys and inhibits the secretion of parathyroid hormone. With significant musculoskeletal consequences, vitamin D deficiency causes rickets in children and osteomalacia and osteoporosis in adults [39].
Thus, the design of new and effective vitamin delivery systems is a fundamental issue because of the importance of vitamins A, B1, C, B3 and D in the body and also, of maintaining biological and chemical properties of vitamins during their transit to the target area in the body. In the present study, the interaction of vitamins A, B1, C, B3 and D with (9, 0) and (5, 5) BNNTs is investigated theoretically based on analyses of electronic properties.
The objective of our calculations is, without recurring to costly experimental methods, to gain fundamental insights into the influence of adsorbed vitamins on the electronic properties of the nanotubes, and also to investigate the way these effects could be used to design drug delivery systems. Moreover, solubility under physiological conditions is a key prerequisite to make BNNT biocompatible [40,41], therefore stability and solubility of the BNNTs and (9, 0)/(5, 5) BNNT–vitamin complexes in solvent phase are investigated to realize the role of the solvent in the BNNTs and their complexes.
Theoretically, an interaction between BNNTs and metformin drug [42], pyrazinamide drug [43], amino acids [5] and nucleobases [44] has been reported. These studies on the functionalization of BNNTs provide a guidance to design BNNT-based devices for biomedical applications.
To the best of our knowledge, this is the first theoretical study that evaluates the possibility of using zigzag and armchair BNNTs as nanovectors for drug delivery of vitamins A, B1, C, B3 and D for the treatment of pandemic deficiency diseases.
2 Computational details
The interaction of vitamins A, B1, C, B3 and D with (9, 0) and (5, 5) BNNTs is studied by the density functional theory (DFT) as implemented in the DMol3 program code [45] at the LDA/DNP (fine) level of theory [46]. It has been verified that the numerical basis sets implemented in DMol3 can minimize or even eliminate basis set superposition error (BSSE).
In our calculations, (9, 0) and (5, 5) BNNTs comprised of 198 and 190 atoms and 0.699 and 0.673 nm in diameter and end terminated with hydrogen atoms (due to the absence of periodic boundary conditions in molecular calculations, it is necessary to saturate the B and N dangling bonds with hydrogen atoms) are considered as the model systems. Vitamins A, B1, C, B3, D, pristine (9, 0) and (5, 5) BNNTs and (9, 0)/(5, 5) BNNT–vitamin complexes are optimized, vibrational frequencies are calculated to insure the absence of imaginary frequencies. For solvation studies, water with a dielectric constant of 78.4 is taken as the solvating medium as it mimics the human biological system. The effect of water on the binding energy of (9, 0)/(5, 5) BNNT–vitamin complexes are determined using our Conductor-like Screening Model (COSMO) [47] method at the LDA/DNP (fine) level of theory. The solvation studies help us to understand the degree of dissolution of (9, 0)/(5, 5) BNNT–vitamin complexes in aqueous media, which is very fundamental to account for the release of BNNT and provides in-depth knowledge of the properties of BNNT loaded with vitamins A, B1, C, B3 and D within the body.
3 Results and discussion
3.1 Solvation and binding energies
Optimized structures of the vitamins A, B1, C, B3, D, (9, 0) and (5, 5) BNNTs and (9, 0)/(5, 5) BNNTs–vitamins complex are shown in Fig. 1.
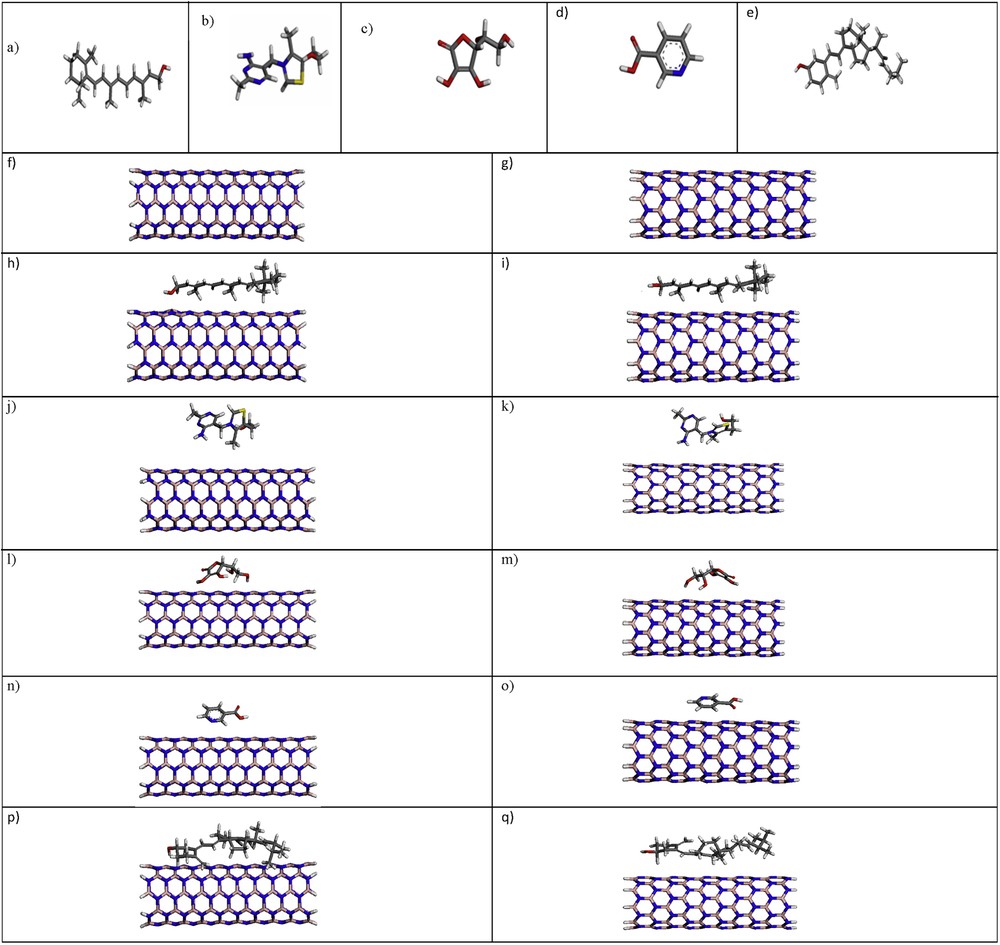
(Color online.) Optimized geometries of (a) vitamin A, (b) vitamin B1, (c) vitamin C, (d) vitamin B3, (e) vitamin D (f) (5, 5) BNNT, (g) (9, 0) BNNT, (h) (5, 5) BNNT–vitamin A complex, (i) (9, 0) BNNT–vitamin A complex, (j) (5, 5) BNNT–vitamin B1 complex, (k) (9, 0) BNNT–vitamin B1 complex, (l) (5, 5) BNNT–vitamin C complex, (m) (9, 0) BNNT–vitamin C complex, (o) (5, 5) BNNT–vitamin B3 complex, (p) (9, 0) BNNT–vitamin B3 complex (q) (5, 5) BNNT–vitamin D complex and (p) (9, 0) BNNT–vitamin D complex. Nitrogen atoms are colored blue, carbon gray, hydrogen white, boron pink, sulfur yellow and oxygen red. (For interpretation of the references to color in this figure, the reader is referred to the web version of this article).
To model the interaction between BNNTs and vitamins A, B1, C, B3 and D, these vitamins are located parallel to the BNNTs axis (to determine the stable adsorption structures, vitamin atoms are allowed to relax freely in all directions). The shortest distance between the (5, 5) and (9, 0) BNNTs with vitamins A, B1, C, B3 and D in the corresponding complexes are found to be about 1.823, 2.506, 2.645, 3.677, 2.363 and 2.668, 3.170, 2.048, 3.184, 2.539 Å, respectively.
The solvation energies of (9, 0) and (5, 5) BNNTs shown in Table 1 indicate that (9, 0) BNNT has a higher solubility compared to (5, 5) BNNT.
Solvation energies (in the presence of water) of pristine vitamins A, B1, C, B3, D, (9, 0), and (5, 5) BNNTs (kcal/mol).
Parameter | (9, 0) BNNT | (5, 5) BNNT | Vitamin A | Vitamin B1 | Vitamin C | Vitamin B3 | Vitamin D |
Solvation energy | –45.67 | –29.69 | –12.76 | –22.61 | –27.75 | –10.86 | –11.58 |
The binding energy of vitamins A, B1, C, B3 and D with (9, 0) and (5, 5) BNNTs is calculated using the equation:
Eb = E(BNNT/X) – [E(BNNT) + E(X)] | (1) |
From the calculated binding energies of (9, 0) BNNT–vitamins and (5, 5) BNNT–vitamins in Tables 2 and 3, the binding energies in both phases are negative, and we propose that adsorption is exothermic and experimentally possible from the energetic viewpoint, and that vitamins A, B1, C, B3 and D are stabilized by the BNNTs surface.
Solvation (in the presence of water) and binding energies (in both phases) of (5, 5) BNNT–vitamin A, (5, 5) BNNT–vitamin B1, (5, 5) BNNT–vitamin C, (5, 5) BNNT–vitamin B3, and (5, 5) BNNT–vitamin D complexes.
Parameter | (5, 5) BNNT–A | (5, 5) BNNT–B1 | (5, 5) BNNT–C | (5, 5) BNNT–B3 | (5, 5) BNNT–D |
Solvation energy (kcal/mol) | –37.28 | –49.77 | –48.45 | –38.40 | –36.93 |
Binding energies (eV) in gas phase | –1.40 | –0.06 | –0.84 | –0.14 | –0.24 |
Binding energies (eV) in solvent phase | –1.19 | –0.15 | –0.51 | –0.35 | –1.61 |
Solvation energies (in the presence of water) and binding energies (in both phases) of (9, 0) BNNT–vitamin A, (9, 0) BNNT–vitamin B1, (9, 0) BNNT–vitamin C, (9, 0) BNNT–vitamin B3, and (9, 0) BNNT–vitamin D complexes.
Parameter | (9, 0) BNNT–A | (9, 0) BNNT–B1 | (9, 0) BNNT–C | (9, 0) BNNT–B3 | (9, 0) BNNT–D |
Solvation energy (kcal/mol) | –52.18 | –66.74 | –68.72 | –45.26 | –53.21 |
Binding energies (eV) in gas phase | –0.54 | –1.50 | –0.69 | –0.49 | –0.82 |
Binding energies (eV) in solvent phase | –0.87 | –0.09 | –0.46 | –0.41 | –1.78 |
Also, the binding energies for (5, 5) BNNT–vitamin B1, (5, 5) BNNT–vitamin B3, (5, 5) BNNT–vitamin D, (9, 0) BNNT–vitamin A and (9, 0) BNNT–vitamin D in solvent phase are more than that of the gas phase, which shows an increase in the thermodynamic stability of these complexes and in the solubility of vitamins B1, B3 and D on (5, 5) BNNT support and vitamins A and D on (9, 0) BNNT support in the solvent phase. Tables 2 and 3 show that the values of the solvation energies for (9, 0)/(5, 5) BNNT–vitamin complexes are negative, indicating that the solvation of complexes is spontaneous.
In comparison to the solvation energies of pristine vitamins A, B1, C, B3 and D with (9, 0)/(5, 5) BNNT–vitamin complexes, it is observed that on loading vitamins A, B1, C, B3 and D on BNNT support, solvation energy gets a further reduction, indicating that the solubility of pristine vitamins A, B1, C, B3 and D is increased in the presence of nanotubes. These results denoted that by the functionalization of BNNTs with vitamins A, B1, C, B3 and D as a nanovector vitamin delivery, the solubility of vitamins A, B1, C, B3 and D in solvent is increased. Moreover, the solvation energies of (9, 0) BNNT–vitamin complexes in solvent phase are more than the solvation energies of (5, 5) BNNT–vitamin complexes that display increased degrees of dissolution of vitamins A, B1, C, B3 and D attached to (9, 0) BNNT in the solvent phase.
3.2 Molecular orbital
The structures and positions of the molecules’ frontier orbitals (HOMO, the highest occupied molecular orbital, and LUMO, the lowest unoccupied molecular orbital) play a major role in governing many chemical reactions and determining electronic band gaps in solids; they are also responsible for the formation of many charge-transfer complexes. Therefore, the spatial distributions of the molecular orbitals (MOs), especially the frontier MOs including HOMO and LUMO are studied for further describing the nature of the interaction between (5, 5) and (9, 0) BNNTs and vitamins A, B1, C, B3 and D. HOMOs and LUMOs of pristine vitamins A, B1, C, B3 and D, (5, 5) and (9, 0) BNNTs, (5, 5) BNNT–vitamin and (9, 0) BNNT–vitamin complexes, are shown in Figs. 2 and 3.
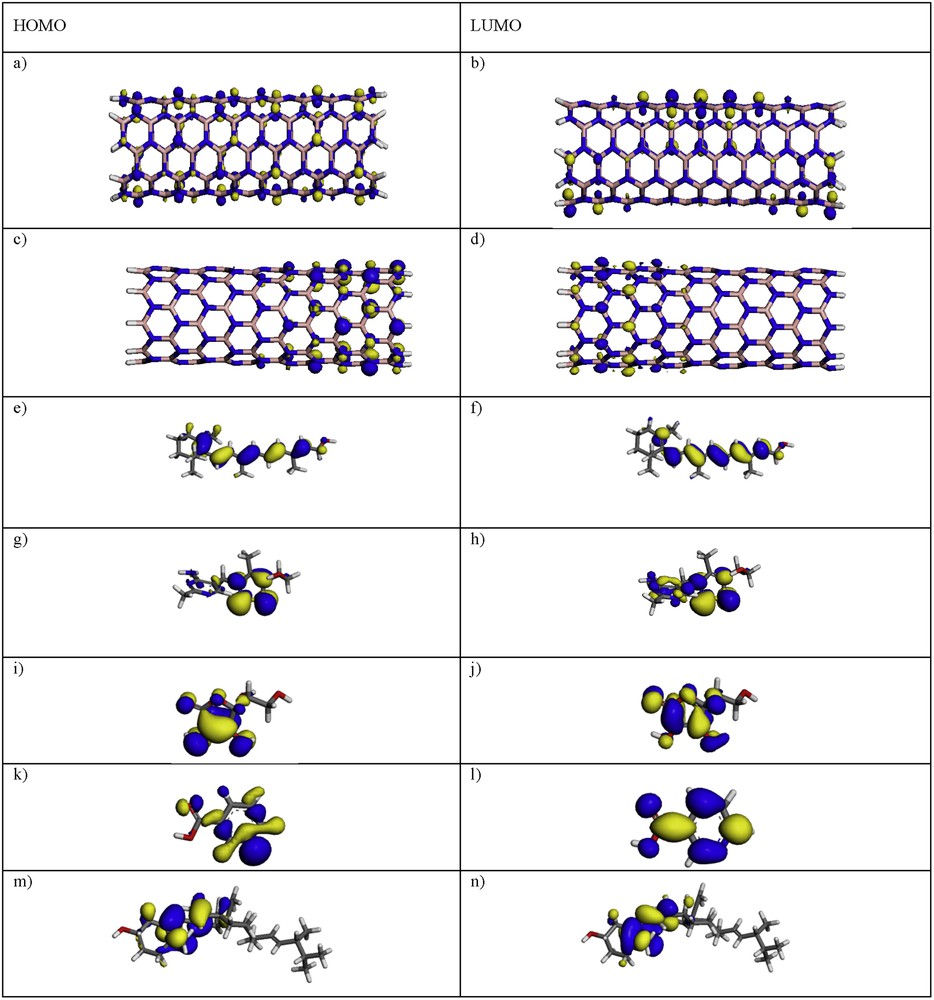
(Color online.) Orbital description of HOMOs and LUMOs (in the gas phase) in (a, b) (5, 5) BNNT, (c, d) (9, 0) BNNT, (e, f) vitamin A, (g, h) vitamin B1, (i, j) vitamin C, (k, l) vitamin B3, and (m, n) vitamin D, optimized at the DFT–LDA/DNP level of theory.
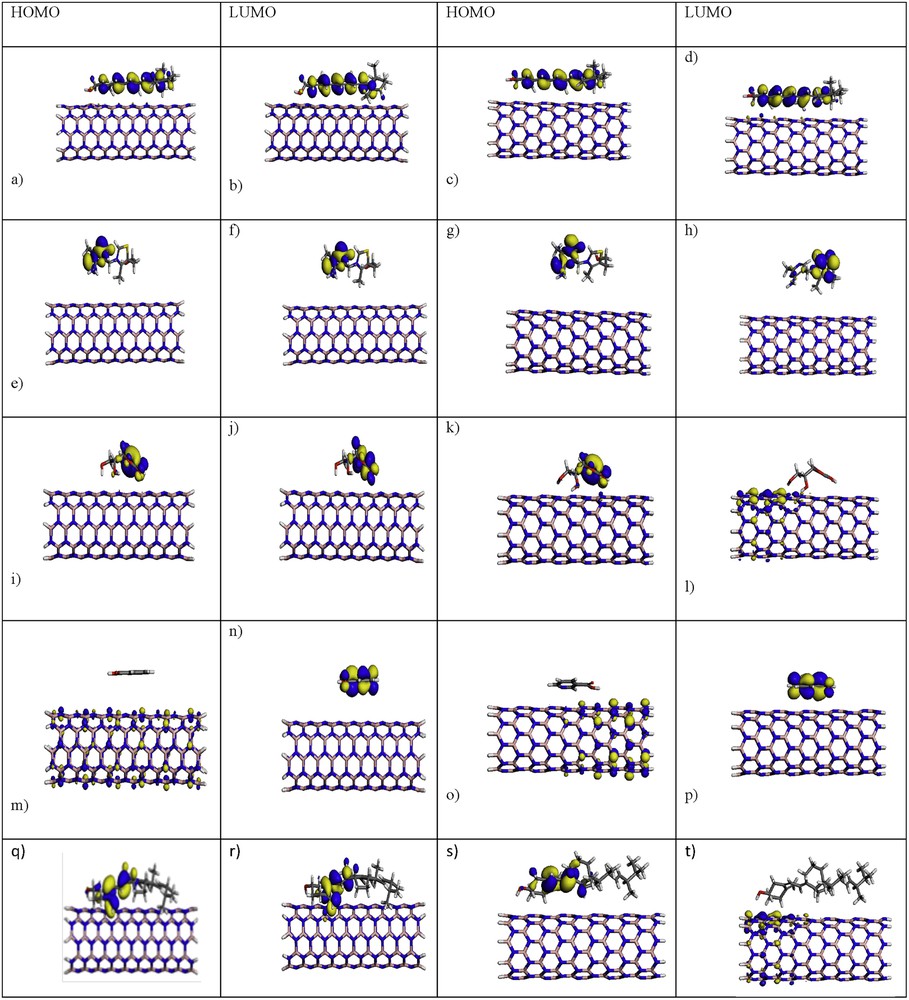
(Color online.) Orbital description of HOMO and LUMO (gas phase) in (a, b) (5, 5) BNNT–vitamin A, (c, d) (9, 0) BNNT–vitamin A, (e, f) (5, 5) BNNT–vitamin B1, (g, h) (9, 0) BNNT–vitamin B1, (i, j) (5, 5) BNNT–vitamin C, (k, j) (9, 0) BNNT–vitamin C, (m, n) (5, 5) BNNT–vitamin B3, (o, p) (9, 0) BNNT–vitamin B3, (q, r) (5, 5) BNNT–vitamin D, and (s, t) (9, 0) BNNT–vitamin D optimized at DFT–LDA/DNP level of theory.
In (5, 5) BNNT, HOMO is distributed on the nitrogen atoms throughout the nanotube and corresponds to the lone pair of electrons on nitrogen atoms, whereas LUMO is uniformly distributed throughout the nanotube. In (9, 0) BNNT, HOMO is located in the nitrogen atoms at the end of the N-terminated, in contrast to LUMO, which is distributed on the boron atoms at the ends of the B-terminated. For the (5, 5) BNNT–vitamin A, (5, 5) BNNT–vitamin B1, (5, 5) BNNT–vitamin C, (5, 5) BNNT–vitamin D, (9, 0) BNNT–vitamin B1 and (9, 0) BNNT–vitamin D, HOMO and LUMO are gathered in the vitamins. Also Figs. 2 and 3 show that, for (9, 0) BNNT–vitamin C and (9, 0) BNNT–vitamin D complexes, HOMO is localized in the vitamins, and LUMO is distributed in the nanotube, in contrast to the case of (5, 5) BNNT–vitamin B3 and (9, 0) BNNT–vitamin B3HOMO, in which HOMO is distributed in the tube, and LUMO is localized in the vitamins. These results indicated that electron conduction occurs chiefly through vitamins A, B1, C, B3 and D.
3.3 Quantum molecular descriptors
Quantum molecular descriptors for popular qualitative chemical concepts with rigorous definitions within the purview of conceptual DFT [48–51] have been provided. Conceptual DFT is a subfield of DFT in which one tries to extract the electronic density relevant concepts and principles. This helps us to understand and predict the chemical behavior of a molecule. The conceptual density functional theory has been quite successful in providing quantitative definitions of popular qualitative chemical concepts like the chemical potential (μ) [52], the global hardness (η) [53], the electrophilicity index (ω) [54,55], etc. From the optimized geometries obtained from gas and solvent phases, global molecular descriptors including μ, η, ω and ΔN [53] are calculated.
The chemical potential, μ, which measures the escape tendency of an electron from equilibrium, is defined as:
μ = –(I + A)/2 | (2) |
The global hardness, η, is a measure of the resistance of a chemical species to a change in its electronic configuration; a global hardness increase leads an increase in the stability and a decrease in the reactivity of the species:
η = (I – A)/2 | (3) |
The electrophilicity index ω represents the stabilization energy of the systems when it gets saturated by electrons that come from the surroundings and also provides information about the structural stability, the reactivity and the toxicity of a chemical species:
ω = μ2/2η | (4) |
Interactions between vitamins A, B1, C, B3 and D with the (5, 5) and (9, 0) BNNTs can be evidenced through parameter ΔN, which determines the partial number of electrons transferred from the vitamins to the (5, 5) and (9, 0) BNNTs.
ΔN = μB – μA/2(ηA – ηB) | (5) |
Tables 4–6 display the values of the quantum molecular descriptors calculated for pristine vitamins A, B1, C, B3 and D, (5, 5) and (9, 0) BNNTs, (5, 5) BNNT–vitamins and (9, 0) BNNT–vitamins complex in the gas and solvent phases.
Quantum molecular descriptors for optimized geometries of (5, 5) BNNT, (9, 0) BNNT, vitamins A, B1, C, B3 and D in gas and solvent phases (eV).
Descriptors | (5, 5) BNNT | (9, 0) BNNT | Vitamin A | Vitamin B1 | Vitamin C | Vitamin B3 | Vitamin D | |||||||
Gas | Solvent | Gas | Solvent | Gas | Solvent | Gas | Solvent | Gas | Solvent | Gas | Solvent | Gas | Solvent | |
EHOMO | –6.02 | –6.02 | –5.59 | –5.89 | –4.53 | –4.74 | –2.70 | –2.82 | –5.56 | –5.80 | –6.02 | –6.44 | –4.79 | –4.98 |
ELUMO | –1.46 | –1.45 | –2.25 | –2.21 | –2.48 | –2.74 | –2.04 | –2.18 | –1.85 | –2.12 | –2.93 | –2.99 | –1.82 | –2.14 |
I = –EHOMO | 6.02 | 6.02 | 5.95 | 5.89 | 4.53 | 4.74 | 2.70 | 2.82 | 5.56 | 5.80 | 6.02 | 5.44 | 4.79 | 4.98 |
A = –ELUMO | 1.46 | 1.44 | 2.25 | 2.21 | 2.48 | 2.74 | 2.04 | 2.18 | 1.85 | 2.12 | 2.93 | 1.50 | 1.89 | 2.14 |
η = (I – A)/2 | 2.27 | 2.28 | 1.84 | 1.83 | 1.02 | 1.00 | 0.33 | 0.32 | 1.85 | 1.83 | 1.64 | 1.72 | 1.48 | 1.42 |
μ = –(I + A)/2 | –3.74 | –3.73 | –4.10 | –4.05 | –3.51 | –3.74 | –2.37 | –2.50 | –3.71 | –3.96 | –4.57 | –4.42 | –3.30 | –3.56 |
ω = μ2/2η | 3.07 | 3.05 | 4.55 | 4.47 | 6.00 | 7.00 | 8.49 | 9.83 | 3.71 | 4.27 | 6.38 | 6.45 | 3.97 | 4.47 |
Quantum molecular descriptors for optimized geometries of (5, 5) BNNT–Vitamin A, (5, 5) BNNT–Vitamin B1, (5, 5) BNNT–Vitamin C, (5, 5) BNNT–Vitamin B3, and (5, 5) BNNT–Vitamin D in gas and solvent phases (eV).
Descriptors | (5, 5) BNNT–Vitamin A | (5, 5) BNNT–Vitamin B1 | (5, 5) BNNT–Vitamin C | (5, 5) BNNT–Vitamin B3 | (5, 5) BNNT–Vitamin D | |||||
Gas | Solvent | Gas | Solvent | Gas | Solvent | Gas | Solvent | Gas | Solvent | |
EHOMO | –4.80 | –4.77 | –5.49 | –5.89 | –5.72 | –5.81 | –6.02 | –6.02 | –4.78 | –4.94 |
ELUMO | –2.86 | –2.82 | –2.09 | –2.16 | –2.17 | –2.25 | –2.82 | –2.99 | –2.03 | –2.16 |
I = –EHOMO | 4.80 | 4.77 | 5.49 | 5.89 | 5.72 | 5.81 | 6.02 | 6.02 | 4.78 | 4.94 |
A = –ELUMO | 2.86 | 2.82 | 2.09 | 2.16 | 2.17 | 2.25 | 2.82 | 2.99 | 2.03 | 2.03 |
η = (I – A)/2 | 0.96 | 0.97 | 1.70 | 1.75 | 1.77 | 1.78 | 1.59 | 1.51 | 1.37 | 1.39 |
μ = –(I + A)/2 | –3.83 | –3.80 | –3.79 | –4.14 | –3.95 | –4.03 | –4.42 | –4.50 | –3.40 | –3.55 |
ω = μ2/2η | 7.59 | 7.43 | 4.23 | 4.88 | 4.39 | 4.58 | 6.13 | 6.70 | 4.22 | 4.54 |
△N = μB – μA/2(ηA – ηB) | 0.09 | –0.01 | 0.04 | 0.31 | –0.01 | 0.03 | –0.65 | 0.87 | 0.27 | 0.09 |
Quantum molecular descriptors for optimized geometries of (9, 0) BNNT–Vitamin A, (9, 0) BNNT–Vitamin B1, (9, 0) BNNT–Vitamin C, (9, 0) BNNT–Vitamin B3 and (9, 0) BNNT–Vitamin D in gas and solvent phases (eV).
Descriptors | (9, 0) BNNT–Vitamin A | (9, 0) BNNT–Vitamin B1 | (9, 0) BNNT–Vitamin C | (9, 0) BNNT–Vitamin B3 | (9, 0) BNNT–Vitamin D | |||||
Gas | Solvent | Gas | Solvent | Gas | Solvent | Gas | Solvent | Gas | Solvent | |
EHOMO | –4.44 | –4.55 | –5.72 | –5.83 | –5.71 | –5.49 | –5.94 | –5.88 | –4.69 | –4.95 |
ELUMO | –2.54 | –2.64 | –2.40 | –2.35 | –2.24 | –2.35 | –2.96 | –3.06 | –2.31 | –2.24 |
I = –EHOMO | 4.44 | 4.55 | 5.72 | 5.83 | 5.71 | 5.49 | 5.94 | 5.88 | 4.69 | 4.95 |
A = –ELUMO | 2.54 | 2.64 | 2.40 | 2.35 | 2.24 | 2.35 | 3.06 | 3.06 | 2.31 | 2.24 |
η = (I – A)/2 | 0.95 | 0.95 | 1.66 | 1.73 | 1.73 | 1.57 | 1.49 | 1.41 | 1.19 | 1.35 |
μ = –(I + A)/2 | –3.49 | –3.60 | –4.06 | –4.09 | –3.98 | –3.92 | –4.45 | –4.47 | –3.50 | –3.60 |
ω = μ2/2η | 6.43 | 6.78 | 4.97 | 4.83 | 4.57 | 4.90 | 6.65 | 7.08 | 5.14 | 6.78 |
△N = μB – μA/2(ηA – ηB) | –0.30 | 0.18 | 0.35 | 0.51 | 0.50 | 0.09 | –1.07 | –3.00 | 0.50 | 0.58 |
Based on Table 4, the global hardness and the ionization potential of (5, 5) BNNT in the gas and solvent phases are higher than (9, 0) BNNT, which signifies that the stability of (9, 0) BNNT is higher than that of (5, 5) BNNT.
When vitamins A, B1, C, B3, and D are adsorbed on the (5, 5) and (9, 0) BNNTs, ELUMO and EHOMO are changed for all systems, so the hardness, the electronic chemical potentials and the electrophilicity of bare BNNTs are altered. On the other hand, the chemical activity of BNNTs can be characterized by the hardness, which is a significant parameter relying on the HOMO and LUMO energy levels. The increase in the global hardness leads to an increase in the stability and a decrease in the reactivity of the species. The results demonstrate that the hardness of pristine (5, 5) and (9, 0) BNNTs in the gas and solvent phases is higher than those of the (5, 5) BNNT–vitamin and (9, 0) BNNT–vitamin complexes in both phases, the global hardness decreasing with the adsorption of vitamins A, B1, C, B3 and D on the (5, 5) and (9, 0) BNNTs due to the increase in chemical reactivity, which also leads to the lowering of the stability of the complexes. Therefore, we can predict that the (5, 5) and (9, 0) BNNTs are relatively stable and that vitamins’ adsorption process is a conqueror. These results are consistent with the results of the binding and solvation energies of the (5, 5) BNNT–vitamins and (9, 0) BNNT–vitamins.
The calculated ω values of pristine BNNTs, of (5, 5) BNNT–vitamins of (9, 0) BNNT–vitamins are reported in Tables 4–6, which suggests that electrophilicity increases in gas and solvent phases are linked with an increase of the charge transfer between vitamins and (5, 5) and (9, 0) BNNTs. These results show that the electronic transport properties of (5, 5) and (9, 0) BNNTs could be significantly changed upon the adsorption of vitamins A, B1, C, B3, and D. The amount of charge transfer between vitamins A, B1, C, B3 and D and the (5, 5) and (9, 0) BNNTs (ΔN) method is given in Tables 5 and 6. A positive value of ΔN indicates that vitamins A, B1, C, B3, and D act as electron acceptors, while a negative value of ΔN indicates that vitamins A, B1, C, B3, and D act as electron donors. In (5, 5) BNNT–vitamins and (9, 0) BNNT–vitamins (except (5, 5) BNNT–vitamin A in the solvent phase, (5, 5) BNNT–vitamin C, (5, 5) BNNT–vitamin B1 and (9, 0) BNNT–vitamin A in the gas phase) in the solvent and gas phases, ΔN is positive, indicating that (5, 5) and (9, 0) BNNTs act as electron donors; in (9, 0) BNNT–vitamin B3 in the solvent and gas phases, ΔN is negative, which means that vitamin B3 acts as an electron donor [57–59].
4 Conclusions
We studied the effect of the adsorption of vitamins A, B1, C, B3, and D as a drug in gas and solvent phases on the electronic structure properties and the quantum molecular descriptors (5, 5)/(9, 0) BNNTs by means of density functional theory (DFT) calculations.
Our results showed that binding energies of the (5, 5) BNNT–vitamin and (9, 0) BNNT–vitamin complexes are negative and also that the functionalization of (5, 5)/(9, 0) BNNT with vitamins A, B1, C, B3 and D is energetically favorable, whereas (5, 5)/(9, 0) BNNT–vitamin complexes are thermodynamically stable. The fact that solvation energies are lower than functionalization energies (in the gas phase) indicated that, in the presence of water, (5, 5)/(9, 0) BNNT–vitamin complexes are stabilized, and also that the solubility of vitamins A, B1, C, B3, and D increased in the presence of BNNTs. From these calculations, we can evidence that the solubilities of (9, 0) BNNT–vitamin complexes are higher than those of (5, 5) BNNT–vitamin complexes, though both solubilities in solvent are thermodynamically favorable. The decrease in the global hardness because of functionalized (5, 5) and (9, 0) BNNTs with vitamins A, B1, C, B3 and D suggests a stability lowering of a reactivity increase of (5, 5)/(9, 0) BNNT–vitamin complexes in both phases.
In summary, our results show that vitamins A, B1, C, B3 and D can be adsorbed on the surface of the BNNTs, a statement that can provide new insight into the development of functionalized boron nitride nanotubes as drug delivery systems within biological environments.
Acknowledgment
The supports of the University of Mazandaran (Islamic Republic of Iran) for research facilities are gratefully acknowledged.