Every year, 3.8 billions cubic meters of wood harvested worldwide are dedicated to the building or furniture manufacturing, for particle-board and paper pulp production and also energetic applications [1]. Such exploitation generates large quantities of by-products such as bark, knots and stumps [2]. These residual plant materials are discarded because of their high content in lignins, polyphenols and resins, detrimental regarding the production of paper pulp and also because of their poor mechanical properties [3–6]. Barks discarded from paper pulp industries per year amounts, in average, to 125 000–250 000 t and knots represent around 5000–10 000 t [7,8]. Since several years, a global concern of the depletion of resources led to the urge of rethinking sustainable processes including wastes recycling. Currently, various agricultural industries already applied such a valorisation scheme, e.g. wine industry with grape pomace recovery [9], or orange industry with limonene and pectin extraction from peel [10], driven by the volumes of wastes to treat, and the value-added of the products generated. By-products from paper pulp industry present advantages such as availability throughout the year, low price, long-term chemical stability and ease of management [11].
Until now, wood by-products were used for the production of steam and energy [7]. However, these residues constitute a rich source of polyphenols and antiradical compounds, efficient against cancers, cardiovascular troubles and neurodegenerative diseases [12,13]. For example, tree knotwood extracts act as strong antioxidants [14,15], also showing antimicrobial and antitumoural activities [16,17]. Extracts from Pinus species, due to their high content in stilbenes, turned out to be particularly active. In the other hand, tree bark extracts are also well-known for their benefits for human health such as maritime pine bark extract, commercialized under the name Pycnogenol®, rich in proanthocyanidins and used against venous insufficiencies, hypertension, inflammation and erythma [18]. Aspen bark extract is an ingredient of a mix called Phytodolor®, for the treatment of rheumatic diseases [19].
Given the amounts of such by-products, an extracting process in a continuous mode at industrial-scale should be designed, to obtain bioactive products from wood industry waste. Twin-screw technology allows the extraction of substances from plant matrix such as oil and pectins from sunflower [20,21], oil from rapeseed [22,23] and from coriander [24], hemicelluloses from aspen [25]. This powerful tool in terms of transformation of the starting materials needs a fine optimization of its operating parameters according to the treated vegetal matter. A twin-screw extractor is based on successive elements allowing several actions in a single step: conveying, heating and cooling, shearing, mixing, solid/liquid extraction, separation and drying [26]. Forward pitch screws allow the conveying combined to a low mixing and shearing of the plant material. Bilobe paddles carry out a radial compression on the matter, shearing and mixing of the solid and the liquid phases. Reversed pitch screws also exert an intense radial compression and a strong shearing combined with mixing of the material and the extracting liquid. The sequence of the screw elements along the profile, the barrel temperature and the solvent-to-solid ratio are the parameters influencing the performance of the extracting process indicated by extraction yields, residence time in the extractor or mechanical energy input [27–30].
So far, no study has been undertaken concerning the extraction of compounds from tree knots, barks and stumps at industrial scale. For the first time, this study set out to evaluate the new application of twin-screw extractor for the recovery of polyphenols and antiradicals and fibres from wood by-products, in a continuous mode. Two representative species of French coniferous and broad-leaves trees exploited in paper pulp industries were studied: maritime pine (Pinus pinaster) and aspen (Populus tremula). The characterization of the extraction performance and the effects of the operating parameters such as the barrel temperature and solvent-to-solid ratio were observed by the determination of plant material breakdown (extrudate and particles yields), solid/liquid separation (dry matter of the extrudate and filtrate recovery rate), the polyphenols yield and the antiradical activity of the extracts.
1 Materials and methods
1.1 Materials
All trials were carried out using a single homogenous batch of wood by-products: maritime pine (Pinus pinaster) knots, supplied by Smurfit Kappa (France), maritime pine stumps, supplied by Tembec (France), aspen knots, and barks, supplied by Tembec/Asian Pulp and Paper (France). After drying in an oven and grounding in a hammer mill (Electra), the powdered material was sieved to select particles smaller than 6 mm. All solvents and chemicals were obtained from Sigma-Aldrich and VWR.
1.2 Characterization of the wood
Dry matter was determined according to the French standard NF V 03-903. The protein content was determined by the Kjeldahl method [31], according to the French standard NF V18-100, applying a multiplying factor of 6.25 to the nitrogen content [32]. The mineral content was determined according to the French standard NF V 03-322. The fibres content (cellulose, hemicelluloses and lignins) was determined according to the ADF–NDF method [33]. The hydrophilic extractives content was determined with aqueous extractions performed with a Tecator Fibertec M1017 (FOSS), during 1 h at 100 °C. Lipophilic extractives content was determined with cyclohexane extractions performed with a Soxhlet, during 6 h at boiling point. Extractions and analyses were performed in triplicate.
1.3 Extracts analysis
The amount of total phenolics was assayed by means of the Folin–Ciocalteu method adapted from Singleton and Rossi (1965) by mixing Folin–Ciocalteu reagent (0.5 mL), sodium carbonate 20% (1 mL), completed with purified water (7.5 mL) and the extracts solution (1 mL) at 70 °C for 10 min and measuring the absorbance of the mixtures at 700 nm at 25 °C [34]. The results are expressed in grams GAE in the initial dry matter.
The hydrogen-donating ability of a crude extract was performed with a 2,2-diphenyl-1-picrylhydrazyl (DPPH) radical-scavenging assay adapted from the procedure described by Brand-Williams, Cuvelier and Berset (1995), by mixing DPPH reagent with the extracts and measuring the absorbance of the mixtures at 516 nm [35]. A reaction curve was plotted as follows:
Analyses were performed in triplicate.
1.4 Twin-screw extractions
Experiments were conducted with a co-rotating and co-penetrating Clextral BC 45 (Firminy, France) twin-screw extruder made of seven modular barrels. Plant material (milled knots, barks and stumps) was fed into the twin-screw extractor inlet port on module 1 by a volumetric screw feeder (Clextral 40, France) at a flow rate of 15 kg/h. Water was injected on module 3, at a flow rate of 15 L/h, and on module 5, at a flow rate from 30 to 75 L/h, (depending on the solvent-to-solid ratio) using piston pumps (Clextral DKM K20-2-PP16 and DKM K20-2-P32, France). After optimizing the experimental extraction processes on theses wood by-products (e.g. forming a dynamic plug able the generate a filtrate), the screws speed was set up at 206 rpm. The modules 3, 4, 5 and 7 were heated by thermal induction and cooled by water circulation. Module 6 was outfitted with a filter consisting of six hemispherical dishes with perforations of 1 mm in diameter, to separate the liquid and the solid phases. The schematic modular barrels of the twin-screw extractor and the screw profile tested were represented on the Fig. 1.
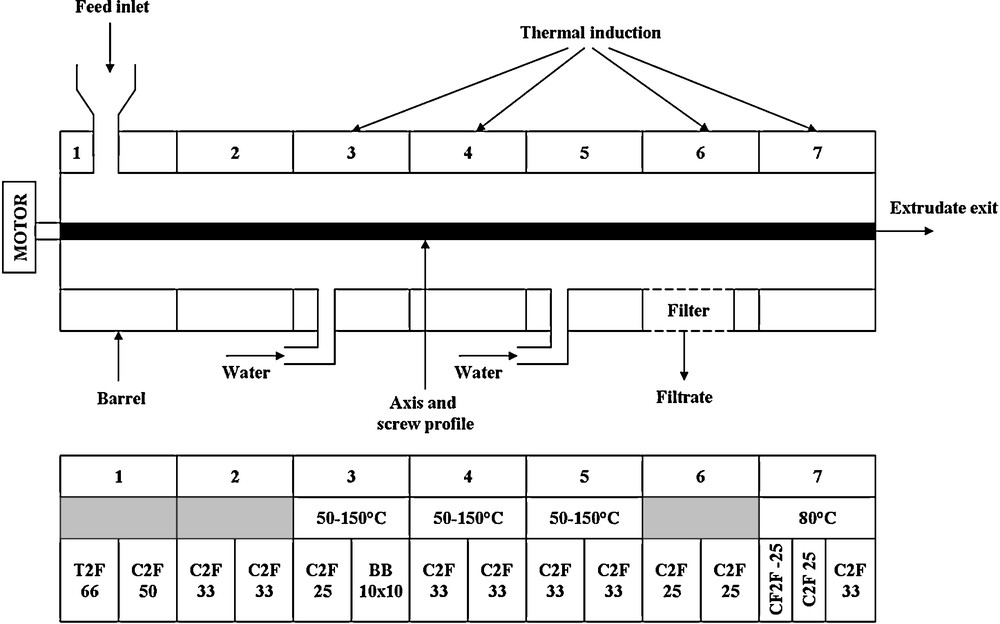
Schematic modular barrel and screw profile of twin-screw extractor BC 45 used for thermo-mechanical fractionation of wood by-products. T2F = trapezoidal double-thread screw; C2F = conveyed double-thread screw; BB = bilobe paddle screw; CF2F = reversed screw. The numbers following the type of the screw indicate the pitch of T2F, C2F and CF2F screws and the length of the BB screws.
The profile of the screw tested consisted in forward pitch screws (T2F and C2F) with a decreasing pitch from 66 to 25 mm from module 1 to module 3 followed by a series of 10 bilobe paddles (BB) disposed with an angle of 90° at the end of module 3, to exert shearing and intimate mixing of the solid and liquid phases. A second section of forward pitch screws with a decreasing pitch from 33 to 25 mm was positioned in modules 4, 5 and 6. The reversed pitch screws, having a negative pitch of –25 mm, were placed at the end of module 6, in the pressing zone, immediately after the filter section. The end of the screw profile consisted in forward pitch screws (CF2F) with an increasing pitch from 25 to 33 mm to expulse the extrudate (defibrated plant material).
The twin-screw extractor was let to function for 15–20 min before sampling to ensure the stabilization of the operating conditions such as feed flow rate of plant material and water, temperature of the modules and current feeding the motor. The filtrate and the extrudate were collected over a period of 15 min to avoid any variability of the outlet flow rates. For each extraction, samples collection was carried out once. A centrifugal filtration was applied to the filtrate to withdraw the fine particles, namely the foot, due to the solid breakdown, at 2000 rpm with a filter porosity of 10 μm. The liquid extracts, the feet and the extrudates were weighted. The total extractives yield YEXT (g per 100 g dry matter) was calculated according to the following formula:
2 Results and discussion
2.1 Characterization of wood by-products
The chemical composition of maritime pine and aspen wood by-products has been studied (Table 1). The extractives content of the different by-products varied depending upon their nature. Indeed, it appears that the knots contained more hydrophilic extractives than stumps for maritime pine, whereas for aspen, bark extractives content was higher than the one of knots. Moreover, hydrophilic extractives content of maritime pine by-products was higher than the one of aspen by-products. In maritime pine, knot's hydrophilic extractives were higher than in stemwood, for which the content reaches 3% dry matter, according to Valette and De Choudens (1992). The bark content was similar to the one previously published by Nunes et al. [36,37]. The hydrophilic extractives content of aspen by-products approached the one of aspen stemwood, determined by Guidi et al. (2009), which is around 5 to 10% dry matter [38]. Lipophilic content of maritime pine knotwood was remarkably high, which was expected, regarding the peculiar environmental stresses to which these parts in coniferous trees are subjected [39]. Willför et al. (2003) found an amount of lipophilic substances in Pinus knots from 4 to 32% dry matter [40]. The marked presence of proteins and minerals in maritime pine stumps is partially due to the sand remaining on the surface of the stumps, but also to oligo-elements present in the zone of exchange between the roots and the soil [41]. Maritime pine and aspen by-products’ fibres content was highly cellulosic and weakly lignified, compared to the stemwood [36,38,42]. Reaction wood, formed in response to mechanical stresses in knots contains gelatinous fibres, with slight or no lignin in coniferous and broad-leaves trees [43,44]. But this weak lignin content given by the Van Soest and Wine dosage can also be due to the presence of polyphenols in the plant material, that can interfere with the reagent used in the assay [45]. Maritime pine knots and aspen barks were the richer by-products in terms of hydrophilic polyphenols, especially the aspen barks, for which polyphenolic content amounted to 24% of the extractable content. In terms of antiradical activity, the evaluation of the extraction processes with DPPH radical appeared as an additional information operating with similar mechanisms of sequential electron transfer (SET) and hydrogen atom transfer (HAT) as it has been described for the Folin–Ciocalteu test [46]. These two methods acted as probes of efficiency of extraction processes. Consequently, the extracts obtained from maritime pine knots or aspen barks exhibited a high antiradical activity, respectively 22.06 and 18.76 mg/L. In the same conditions, the single reference Trolox showed an IC50 value of 3.66 mg/L. Consequently, it appears that water turned out to be an efficient solvent for the extraction of polyphenols and antiradicals from these wood by-products.
Chemical composition of the wood by-products (% of dry matter).
Maritime pine | Aspen | |||
Knots | Stumps | Knots | Barks | |
Minerals | 0.31 ± 0.05 | 7.92 ± 0.19 | 1.20 ± 0.18 | 4.75 ± 0.50 |
Proteins | 0.38 ± 0.02 | 3.63 ± 0.03 | 1.00 ± 0.03 | 2.69 ± 0.13 |
Cellulose | 50.86 ± 1.32 | 57.55 ± 1.42 | 63.03 ± 1.01 | 54.78 ± 0.74 |
Hemicellulose | 24.58 ± 0.42 | 9.51 ± 1.25 | 14.60 ± 0.23 | 12.64 ± 0.86 |
Lignins | 15.44 ± 1.22 | 15.47 ± 0.08 | 15.38 ± 0.81 | 12.63 ± 0.28 |
Lipophilic extractives | 16.74 ± 0.17 | – | 3.80 ± 0.19 | 2.57 ± 0.16 |
Hydrophilic extractives | 12.94 ± 0.97 | 8.30 ± 0.33 | 4.49 ± 0.35 | 7.33 ± 0.27 |
Polyphenolic content | 1.68 ± 0.04 | 0.59 ± 0.02 | 0.43 ± 0.03 | 1.74 ± 0.02 |
Polyphenolic content of the hydrophilic extract (mg GAE/g dry extract) | 132 ± 6 | 72 ± 2 | 88 ± 6 | 23 3 ± 5 |
Antiradical ativity of the hydrophilic extracts (IC50 mg/L) | 22.1 ± 2.9 | 63.6 ± 2.6 | 31 ± 4.1 | 18.8 ± 2.5 |
2.2 Influence of operating conditions on twin-screw extractions of wood by-products
The first observation is that in the experimental field studied, the extractions were feasible without clogging or the impossibility to form a fibrous dynamic plug by the reverse pitch screws.
From the inlet and outlet flow rates obtained for each trial, the extraction yields were calculated and the specific mechanical energy, the polyphenolic content and the antiradical activity of the extracts were determined for maritime pine knots (Table 2), maritime pine stumps (Table 3), aspen knots (Table 4) and aspen barks (Table 5).
Solid and liquid flow rates and results of maritime pine knots conducted with a Clextral BC 45 twin-screw extractor.
Trial | ||||||
1 | 2 | 3 | 4 | 5 | 6 | |
Operating conditions solvent-to-solid ratio | 3.29 | 3.23 | 5.59 | 4.57 | 5.47 | 3.32 |
Temperature (°C) | 50 | 100 | 100 | 125 | 150 | 150 |
QI (kg/h) | 13.4 | 14.2 | 13.5 | 13.2 | 13.8 | 13.7 |
QW (L/h) | 45.0 | 45.0 | 75.0 | 60.0 | 75.0 | 45.0 |
Extrudate | ||||||
QS (kg/h) | 11.8 | 12.6 | 11.2 | 11.4 | 11.5 | 11.9 |
DMS (%a) | 64.1 | 67.4 | 65.9 | 67.2 | 66.9 | 66.7 |
YS (%b) | 88.1 | 88.7 | 83.0 | 86.9 | 83.3 | 86.4 |
Foot | ||||||
QFO (kg/h) | 1.4 | 1.35 | 2.09 | 1.58 | 2.04 | 1.59 |
YFO (%b) | 10.4 | 9.5 | 15.5 | 11.6 | 14.8 | 12.0 |
Filtrate | ||||||
QFIL (kg/h) | 32.6 | 31.6 | 57.3 | 44.2 | 56.3 | 31.5 |
RFIL (%c) | 71.8 | 69.1 | 76.0 | 69.4 | 74.7 | 73.2 |
QEXT (kg/h) | 0.19 | 0.2 | 0.24 | 0.21 | 0.23 | 0.2 |
YEXT (%b) | 1.4 | 1.4 | 1.8 | 1.5 | 1.7 | 1.6 |
QPP (kg/h) | 0.015 | 0.023 | 0.029 | 0.023 | 0.029 | 0.018 |
YPP (%b) | 0.11 | 0.16 | 0.21 | 0.13 | 0.21 | 0.17 |
SME (W.h/kg) | 280 | 359 | 261 | 266 | 268 | 289 |
IC50 (mg/L) | 90 | 65 | 56 | 80 | 57 | 64 |
a g per 100 g of extrudate.
b g per 100 g of dry plant material.
c g per 100 g of water injected.
Solid and liquid flow rates and results of the extractions of maritime pine stumps conducted with a Clextral BC 45 twin-screw extractor.
Trial | ||||||
1 | 2 | 3 | 4 | 5 | 6 | |
Operating conditions solvent-to-solid ratio | 3.19 | 3.21 | 6.28 | 4.62 | 6.76 | 3.42 |
Temperature (°C) | 50 | 100 | 100 | 125 | 150 | 150 |
QI (kg/h) | 13.9 | 13.8 | 13.5 | 13.9 | 12.5 | 12.9 |
QW (L/h) | 44.3 | 44.3 | 84.1 | 63.4 | 84.1 | 43.8 |
Extrudate QS (kg/h) | 12.9 | 12.9 | 11.7 | 12.2 | 10.6 | 11.8 |
DMS (%a) | 62.0 | 67.2 | 68.0 | 70.2 | 67.9 | 69.3 |
YS (%b) | 92.8 | 93.5 | 86.7 | 91.5 | 84.8 | 87.8 |
Foot | ||||||
QFO (kg/h) | 0.72 | 0.62 | 1.35 | 1.26 | 1.52 | 0.85 |
YFO (%b) | 5.2 | 4.5 | 10.0 | 6.6 | 12.2 | 9.1 |
Filtrate | ||||||
QFIL (kg/h) | 32.2 | 31.8 | 73.9 | 51.9 | 72.2 | 28.7 |
RFIL (%c) | 71.7 | 70.8 | 87.2 | 64.6 | 85.2 | 81.1 |
QEXT (kg/h) | 0.27 | 0.29 | 0.39 | 0.37 | 0.39 | 0.27 |
YEXT (%b) | 1.94 | 2.1 | 2.9 | 2.1 | 3.1 | 2.7 |
QPP (kg/h) | 0.016 | 0.019 | 0.026 | 0.025 | 0.025 | 0.021 |
YPP (%b) | 0.12 | 0.14 | 0.19 | 0.16 | 0.20 | 0.18 |
SME (W.h/kg) | 515 | 471 | 546 | 499 | 499 | 460 |
IC50 (mg/L) | 253 | 245 | 220 | 218 | 202 | 199 |
a g per 100 g of extrudate.
b g per 100 g of dry plant material.
c g per 100 g of water injected.
Solid and liquid flow rates and results of the extractions of aspen knots conducted with a Clextral BC 45 twin-screw extractor.
Trial | ||||||
1 | 2 | 3 | 4 | 5 | 6 | |
Operating conditions solvent-to-solid ratio | 3.27 | 3.35 | 6.22 | 4.28 | 5.77 | 2.98 |
Temperature (°C) | 50 | 100 | 100 | 125 | 150 | 150 |
QI (kg/h) | 14 | 13.6 | 14.6 | 16 | 15.7 | 15.3 |
QW (L/h) | 44.5 | 44.5 | 90 | 67.5 | 90 | 45 |
Extrudate | ||||||
QS (kg/h) | 13.4 | 13.1 | 13.5 | 15.1 | 14.7 | 14.9 |
DMS (%a) | 56.5 | 57.5 | 60.3 | 63.1 | 59.3 | 61.9 |
YS (%b) | 95.7 | 96.3 | 92.5 | 97.4 | 93.6 | 94.4 |
Foot | ||||||
QFO (kg/h) | 0.32 | 0.32 | 0.75 | 0.58 | 0.73 | 0.25 |
YFO (%b) | 2.3 | 2.4 | 5.1 | 1.6 | 4.6 | 3.6 |
Filtrate | ||||||
QFIL (kg/h) | 29.4 | 28.3 | 68 | 44.8 | 66.8 | 26 |
RFIL (%c) | 65.2 | 62.7 | 75.1 | 56.9 | 73.6 | 65.7 |
QEXT (kg/h) | 0.21 | 0.23 | 0.3 | 0.25 | 0.3 | 0.22 |
YEXT (%b) | 1.50 | 1.7 | 2.1 | 1.4 | 1.9 | 1.6 |
QPP (kg/h) | 0.013 | 0.016 | 0.024 | 0.018 | 0.022 | 0.014 |
YPP (%b) | 0.09 | 0.12 | 0.16 | 0.09 | 0.14 | 0.11 |
SME (W.h/kg) | 621 | 637 | 606 | 566 | 619 | 535 |
IC50 (mg/L) | 178 | 118 | 107 | 133 | 120 | 132 |
a g per 100 g of extrudate.
b g per 100 g of dry plant material.
c g per 100 g of water injected.
Solid and liquid flow rates and results of the extractions of aspen barks conducted with a Clextral BC 45 twin-screw extractor.
Trial | ||||||
1 | 2 | 3 | 4 | 5 | 6 | |
Operating conditions solvent-to-solid ratio | 4.66 | 4.22 | 9.69 | 8.21 | 8.75 | 4.53 |
Temperature (°C) | 50 | 100 | 100 | 125 | 150 | 150 |
QI (kg/h) | 9.5 | 10.5 | 8.7 | 7.8 | 9.7 | 9.8 |
QW (L/h) | 44.3 | 43.8 | 84.1 | 63.4 | 84.1 | 43.8 |
Extrudate | ||||||
QS (kg/h) | 7.8 | 8 | 5.9 | 5.7 | 6.5 | 7.4 |
DMS (%a) | 40.6 | 57.1 | 56.7 | 59.7 | 58.0 | 57.0 |
YS (%b) | 82.1 | 76.2 | 67.8 | 75.5 | 67.0 | 73.1 |
Foot | ||||||
QFO (kg/h) | 1.44 | 2.07 | 2.3 | 1.53 | 2.5 | 1.76 |
YFO (%b) | 15.2 | 19.7 | 26.4 | 18.0 | 25.8 | 19.6 |
Filtrate | ||||||
QFIL (kg/h) | 28.3 | 30.3 | 74.9 | 56.4 | 74.9 | 33.1 |
RFIL (%c) | 62.9 | 68.1 | 88.4 | 74.4 | 88.4 | 88.3 |
QEXT (kg/h) | 0.27 | 0.46 | 0.53 | 0.53 | 0.67 | 0.63 |
YEXT (%b) | 2.84 | 4.4 | 6.1 | 6.4 | 6.9 | 6.8 |
QPP (kg/h) | 0.025 | 0.051 | 0.059 | 0.076 | 0.076 | 0.069 |
YPP (%b) | 0.26 | 0.49 | 0.68 | 0.70 | 0.78 | 0.97 |
SME (W.h/kg) | 294 | 358 | 437 | 361 | 350 | 405 |
IC50 (mg/L) | 181 | 65 | 74 | 60 | 42 | 82 |
a g per 100 g of extrudate.
b g per 100 g of dry plant material.
c g per 100 g of water injected.
The effect of solvent-to-solid ratio and the barrel temperature on the extraction has been studied using the extrudate yield and the foot yield (fine particles due to the breakdown of the plant material) indicating the level of defibrating of the plant matrix, the filtrate recovery rate and the dry matter of the extrudate indicating the efficacy of pressing the solid/liquid mixture, the specific mechanical energy giving information on the energy necessary to defibrate the woody material and the extractives and polyphenols yields, combined to the antiradical activity of the extracts, which represent the aim of the extractions. First, the effect of the solvent-to-solid ratio on the extrudate yield, the foot yield and the filtrate recovery rate dominated the effect of the barrel temperature, for all the wood by-products. These results suggested that an increase of the solvent-to-solid ratio led to a higher breakdown of the material and a better solid/liquid separation. In the screw profile configuration and the flow rates chosen, an elevation of the barrel temperature from 100 to 150 °C did not have any effect on the breakdown, the shredding and the pressing of the materials studied. However, in the case of aspen barks, and for a solvent-to-solid ratio of 3, an increase of the barrel temperature from 50 to 100 °C, the extrudate yield decreased from 82 to 68%, the foot yield increased from 15 to 26%, the dry matter of the extrudate increased from 41 to 57% and the filtrate recovery rate increased from 63 to 88%, showing an effect of the barrel temperature on the breakdown of the material and solid/liquid separation at low solvent-to-solid ratios.
Besides, a major positive effect of the solvent-to-solid ratio on the total extractives and the polyphenols yields had been established for maritime pine knots and stumps, and for aspen knots. Between a ratio of 3 and 6, the extractives yields respectively increased from 1.4 to 1.8% for maritime pine knots, from 1.9 to 3.1% for maritime pine stumps and from 1.5 and 2.1% for aspen knots. The polyphenols yields increased by 0.05% for the three materials, which represented a gain of 25% in extracted polyphenols. Hence, the breakdown of the material and the better pressing occurring at high solvent-to-solid ratio enhanced the extractions of desired compounds, due to the higher release of extractives when the fibrous network of plant cell wall is disrupted. This effect can also be explained by a larger diffusion of extractives to a more diluted solvent at high solvent-to-solid ratios, leading to the exhaustion of the plant material. The effect of the barrel temperature on extractives and polyphenols yields seemed non-significant. The influence of solvent-to-solid ratio has already been established by Evon et al., for the twin-screw extraction of oil from sunflower press cake [21,47]. Extractives yields beyond 1.7% for maritime pine knot, and beyond 2 and 6% respectively for aspen knots and barks were in the same range as the one performed at high temperature and high pressure conditions with an accelerated solvent extractor, using an acetone/water mixture 95:5 (v/v) on knots from Pinus species, and knots and barks from Populus tremula, that led to respective extractives yields of 3–19%, 6.3% and 18% dry matter [14,15].
Regarding aspen barks, the solvent-to-solid ratio and the barrel temperature showed a similar influence on the extractives yields and the polyphenols yields. The comparison of the extractions carried out at a solvent-to-liquid ratio of 3 and a barrel temperature of 50 °C (YEXT = 2.8% and YPP = 0.26%) showed that the recovery of polyphenols was multiplied by 3 at a ratio of 6 and a barrel temperature of 150 °C (YEXT = 6.9% and YPP = 0.78%). Temperature is known for its effect on cell walls breakdown, which facilitates extractives recovery. In addition, the variations of SME observed in the field of solvent-to-solid ratio from 3 to 6 and of the barrel temperature from 100 to 150 °C, are relatively slight. In the case of aspen knots, an increase of the barrel temperature tended to decrease the SME. The variation of SME did not seem to be correlated to the breakdown of the plant material. Nevertheless, the twin-screw extraction of maritime pine knots and aspen bark required 1.5 to 2 less energy than the one of maritime pine stumps and aspen knots. This result may be due to the presence of lipophilic compounds (detected thanks to Soxhlet extractions with cyclohexane) acting as lubricant inside the extractor.
The antiradical activity of the extracts, allowing one to evaluate the efficiency of such extraction, did not varied much in the experimental field studied and no clear dependence on the solvent-to-solid ratio and the barrel temperature can be detected. Overall, the extracts obtained from all the by-products at a ratio higher than 3 and at a barrel temperature higher than 50 °C were stronger radical scavengers, especially the extracts of maritime pine knots and aspen bark, exhibiting an antiradical activity respectively close to IC50 values of 60 and 40 mg/L.
2.3 Efficacy and processability of twin-screw extraction of wood by-products
Regarding maritime pine knots, at a solvent-to-solid ratio of 6 and a barrel temperature of 100 °C, twin-screw extractives yield was of 1.8%, or 13% of the extractable potential determined with macerations in water at 100 °C during 1 h, and polyphenols yield was of 0.21%, corresponding to 12.5% of the polyphenolic potential of the material. Yet, these yields were obtained for a shorter residence time, from one to several minutes for woody material, in such operating conditions, explaining the non-exhaustion of the plant material [25]. As the extraction yields showed a clear dependence on the solvent-to-solid ratio, macerations performed at a ratio of 100 exhausted obviously more compounds from plant matrix than a ratio from 3 to 6. These quite modest polyphenols yields can also be due to the use of high barrel temperatures that may denature thermo-sensitive compounds [48]. Nevertheless, the content of polyphenols in the maritime pine knots extracts (from 78 to 145 mg GAE per g dry extract) and their antiradical activity were the higher among the four by-products, obtained for a low SME. Maritime pine knots were an interesting material to illustrate the efficacy of twin-screw extractor for the recovery of polyphenolic and antiradical fractions from wood by-products.
The extraction of the polyphenols from maritime pine stumps was also efficient in twin-screw extractor since at 100 °C and at the solvent-to-solid ratio of 3, the polyphenols yield was of 0.15%, corresponding to an extraction of 25% of the polyphenolic content of the material determined at 100 °C during 1 h, for a solvent-to-solid ratio of 100. An increase of this ratio from 3 to 6 and, in a lesser extend, an increase of the barrel temperature from 100 to 150 °C, improved the extraction until recovering 40% of the hydrophilic extractives content and 35% of the polyphenolic content of this material. However, the presence of a significant part of minerals, remaining despite the washing of the material, was responsible of the abrasion of the screws, which decreased the accuracy of a twin-screw treatment for stumps.
Aspen knots were the harder and the less shredded material among the four by-products subjected the twin-screw extraction. The generation of fine particles due to the knots breakdown and present in the filtrate was limited at 5% of the dry mass introduced. At a solvent-to-solid ratio of 6 and a barrel temperature of 150 °C, extractives and polyphenols yields respectively reached 3% and 0.15%, which represent 66% and 35% of potentials determined with water at 100 °C during 1 h. The antiradical activity of the extracts was the fifth of the potential determined with extractions in water at 100 °C during 1 h: it appears that an increase of the barrel temperature beyond 150 °C could be detrimental for the radical scavenging activity of the extracts.
Aspen barks led to the higher extractives and polyphenols yields in twin-screw extractions. Thus, for a solvent-to-solid ratio of 3, these extractions allowed to reach 60% of the extractives yield obtained with maceration in water at 100 °C during 1 h, with a solvent-to-solid ratio of 100. An increase of the barrel temperature and the solvent-to-solid ratio improved these yields: at a solvent-to-solid ratio of 4.5 and a barrel temperature of 125 °C, polyphenols yields was of 1%, representing 50% of the extractable polyphenolic content of the material. Hence, aspen barks are interesting for the recovery of fractions rich in polyphenols and highly active antiradicals, which illustrates the efficacy of the twin-screw extraction of wood by-products.
3 Conclusion
Aqueous extraction of wood by-products was performed using twin-screw technology. This technology allows a thermo-mechanical and a chemical treatment of the plant material using water as extracting solvent, in a single step and in a continuous mode. The Clextral BC 45 twin-screw extractor, with the described screw profile and at 15 kg/h of solid flow rate, in an experimental field of a solvent-to-solid ratio between 3 and 6 and a barrel temperature between 50 and 150 °C, turned out to be efficient for the recovery of polyphenols and antiradicals from wood by-products. A positive effect of solvent-to-solid ratio on the efficacy of the extraction, e.g. the breakdown of the material and the pressing of the solid/liquid mix, was noted for all the plant materials. For aspen barks, extraction yields also showed a dependence on the barrel temperature.
Maritime pine and aspen are representatives of the coniferous and the broad-leaves trees usually treated by European paper industries, suggesting that this flexible process can be applied to a larger woody biomass directly available as discarded residues. The use of a twin-screw extractor Clextral BC 45 type would allow the valorisation of 108 tons of by-products, at a solid flow rate of 15 kg/h for 24 h for 300 days, which would absorb a large part of the by-products yearly generated.
The extracts, thanks to their polyphenolic content and their high antiradical activity, can be used as mass products (preservatives for woody materials, antioxidant for paints) or as fine value-added substances (for food and health applications). Moreover, despites an energetic demand higher for the single extraction step compared to a batch extraction, the extrudate, a defibrated plant material obtained in the end of the extraction at high dry matter rates, can also be reused in the paper pulp process but also for the production of agromaterials [49]. A valorisation of the extrudate as raw material for the thermopressing of particle-boards is currently under study. Therefore, such a full process scheme can be considered in an environmentally comprehensive approach. The thermo-mechanical treatment of wood by-products permits to expose this biomass to a range of disruptive conditions to make it more available for its conversion to biofuels [50].
Acknowledgements
The authors are grateful for the facilities provided by the “Institut national polytechnique de Toulouse”. This work is part of the activities of the “Laboratoire de chimie agro-industrielle”, ENSIACET and was supported by the “Fonds unitaire interministériel”, program BIOEXTRA.