1 Introduction
The Henry reaction or nitroaldol reaction, which was first discovered and named by Louis Henry [1] in 1895, is one of the most important C–C-bond-forming reactions in organic synthesis. The corresponding products of this reaction, a coupling between nitro alkanes and carbonyl groups, can be conveniently converted into a variety of valuable building blocks depending on the different requirements in the synthesis of natural products and other pharmacologically active compounds [2–4]. There has been a lot of interest in developing highly enantioselective syntheses of β-nitro alcohols in recent years, and this reaction has attracted much interest from many excellent chemical workers [5–15], since Shibasaki [16] reported the first catalytic asymmetric Henry reaction in 1992.
Tridentate chiral Schiff-base is one of the promising ligands in asymmetric catalysis, which has frequently been used in many catalytic asymmetric syntheses. Although chiral amino alcohols have frequently been used in catalytic asymmetric synthesis, only a few studies use tridentate chiral Schiff-base ligands in catalytic asymmetric Henry reaction. Recently, Zhiyong Wang [17] reported that a series of novel copper(II) Schiff-base complexes, which could be easily prepared from a natural amino acid, had been used to catalyze asymmetric Henry reactions under mild conditions. Besides, Rui Wang [18] reported that four polymer-supported Schiff-base of optically active amino alcohols were used as chiral ligands in the enantioselective addition of alkynylzinc to simple ketones with moderate to good ee values (ee% up to 89%). However, there has not been a lot of research about the reuse of excellent catalysts for the asymmetric Henry reaction. The initial aim that motivated our study was to improve the recyclability of the catalyst, and we would like to synthesize some excellent polymer-supported chiral Schiff-bases that could be reused several times for the asymmetric Henry reaction.
In this paper, a series of tridentate chiral Schiff-base ligands and their corresponding polymer-supported ligands (Fig. 1) have been prepared and applied to the catalytic asymmetric Henry reaction (Fig. 2). In order to promote these results and acquire some information on the relationship between the structure of a complex and its enantioselectivity in the Henry reaction, we have been making continuous efforts to modify the ligands for the asymmetric Henry reaction. The experimental results showed that the corresponding β-nitro alcohols were obtained in moderate to high yields (up to 98%) with up to 98% ee in accordance with the table under mild conditions. Polymer-supported ligands could be recycled by a simple filtration and the recovered catalyst could be reused 6 times at least with similar good catalytic effect (about 94% yields and 90% ee).
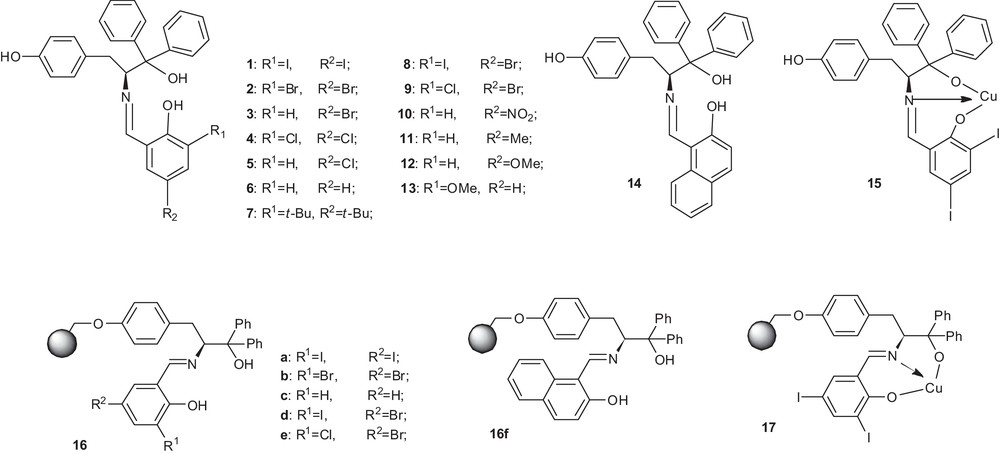
The structure of the chiral catalytic ligands.

Model reaction used as asymmetric Henry reaction.
2 Results and discussion
2.1 Results and discussion about ligands 1–15
At first, in order to get the optimized ligand, ligands 1–14 with different substituents on the phenol ring were synthesized and employed in the asymmetric Henry reaction. As shown in Table 1, ligand 1 (Table 1, entry 1, 98% yield, 93% ee) was the optimized ligand and ligands 1–14 with the substituents on the phenol ring had a great influence on the ee values, but little influence on the reaction yield. The experimental results showed that ligand 6 (entry 6), without any substituent on the phenol ring, can give 95% yield and 87% ee value. When the iodine atom (or the bromine atom) was located in both ortho-(R1) and para-(R2) positions to the phenol ring, the higher ee value 93% (entry 1) or 90% (entry 2) could be obtained. However, the chlorine atom or the tert-butyl group led to a significant decrease in ee values, which was only 80% (entry 4) or 74% (entry 7), respectively. In addition, when the chlorine atom was located in ortho position (R1) and the bromine atom was located in para position (R2) to the phenol ring, a 92% ee value (entry 9) can be obtained. But when the iodine atom was located in ortho position (R1) and the bromine atom was located in para position (R2) to the phenol ring, the ee value was decreased by only 73% (entry 8). Thus, this showed that the substituent located in ortho-(R1) and para-(R2) positions to the phenol ring, which are strong electron-withdrawing groups, can improve the catalytic activity of the reaction, especially when the substituent was in ortho-position (R1). However, the tert-butyl group gave a low ee value because of its great hindrance. If the substituent group (bromine, chlorine, nitryl, methyl, and methoxyl groups) was only located in para position (R2) to the phenol ring, the ee values were all decreased (entries 3, 5, 10–12). A strong electron-withdrawing substituent like nitryl group (entry 10) had a little higher ee value compared to those of weak electron-withdrawing (entries 3, 5) and electron-donating (entries 11, 12) substituents. When the methoxyl group was only located in ortho position (R1) to the phenol ring, the ee value also decreased. But the ortho-position (R1) product had a little higher ee value than the para-position (R2) product. It indicated that the substituent group with a strong electron-donating effect will make the effect of the catalyst fall sharply. Finally, we exploited naphthyl phenol as a substitute for salicylaldehyde to get ligand 14. What was surprising was that the obtained ligand 14 increased the ee value (entry 14, 98% yield, 90% ee) a little more than ligand 6. This suggested that the substituent with great steric hindrance had little influence on this reaction because of its obvious space effect. In addition, it is worth noting that the copper complex ligand 15 (entry 15) gave the same yield as ligand 14 and similar ee values.
Screening of the ligands for the asymmetric Henry reactiona.
Entry | Ligand | Time (h) | Yieldb (%) | eec (%) | Configd |
1 | 1 | 48 | 98 | 93 | S |
2 | 2 | 48 | 95 | 90 | S |
3 | 3 | 48 | 94 | 76 | S |
4 | 4 | 48 | 98 | 80 | S |
5 | 5 | 48 | 99 | 75 | S |
6 | 6 | 48 | 95 | 87 | S |
7 | 7 | 48 | 99 | 74 | S |
8 | 8 | 48 | 95 | 73 | S |
9 | 9 | 48 | 93 | 92 | S |
10 | 10 | 48 | 99 | 79 | S |
11 | 11 | 48 | 98 | 73 | S |
12 | 12 | 48 | 95 | 78 | S |
13 | 13 | 48 | 99 | 76 | S |
14 | 14 | 48 | 98 | 90 | S |
15e | 15 | 48 | 98 | 92 | S |
a Conditions: 0.2 mmol of 4-nitrobenzaldehyde, 6 mmol (30 equiv.) of nitromethane, 2 mL of EtOH, 0.005 mmol of ligand 1–14, 0.0055 mmol of Cu(OAc)2·2H2O, r.t.
b Isolated yield.
c Determined by HPLC with chiral column (AD–H).
d The absolute configurations of the products were determined by comparison with values from the literature [19].
e No Cu(OAc)2·2H2O was added.
Further optimization of the reaction conditions of ligand 1 was tested in the asymmetric Henry reaction between 4-nitrobenzaldehyde and nitromethane. The results are summarized in Tables 2–4. The data clearly showed that the protonic solvents were superior to the non-protonic ones (Table 2). It is important to note that the protonic solvents had more advantages than the only chiral inductive effect for this catalytic system, especially ethanol (Table 2, entry 1, 98% yield, 93% ee). Then, in order to optimize the loading of ligand, raising the loading of ligand 1 (Table 3, entries 1–2) from 1 mol% to 2.5 mol% would led to an increase in yield from 90% to 93% and in ee value from 88% to 93%. But if one continued to raise the amount of ligand 1 from 2.5 mol% to 15 mol% (Table 3, entries 3–7), the reaction gave almost the same yield and ee value. Raising the loading of nitromethane (Table 3, entries 8–12) from 1 to 30 equiv led to a significant increase in yield from 76% to 98% and in ee value from 71% to 93%. If the loading of nitromethane was further raised from 30 to 40 equiv (Table 3, entry 13), the reaction gave almost the same yield, but with a little decrease in ee value. Moreover, among different kinds of metal salts (Table 4), copper salts were superior to other metal salts such as Fe, Co, Zn salts for this reaction system (Table 4, entry 1, 98% yield, 93% ee), Cu(OAc)2·2H2O was established as the best for this reaction system. Since Cu(II) can accept the lone pair of electrons from the ligand to form a stable complex and acetate as a Brønsted base, it activated nitromethane to promote the reaction smoothly and had more advantages over the chiral inductive effect for this catalytic system. For strong acid salts such as copper chloride and copper sulfate, chloride and sulfate ions acting as Brønsted bases could not make the nucleophilic reagent deprotonate effectively due to a lack of alkalinity.
Effect of the solvents on the enantioselective Henry reactiona.
Entry | Solvent | Time (h) | Yieldb (%) | eec (%) |
1 | EtOH | 48 | 98 | 93 |
2 | MeOH | 48 | 96 | 90 |
3 | i-PrOH | 48 | 88 | 89 |
4 | CH2Cl2 | 60 | 86 | 78 |
5 | THF | 48 | 95 | 86 |
6 | Acetone | 48 | 95 | 89 |
7 | DMF | 48 | 92 | 87 |
8 | Toluene | 48 | 94 | 90 |
9 | Et2O | 48 | 98 | 88 |
10 | CH3CN | 48 | 91 | 81 |
11 | Hexane | 48 | 89 | 82 |
a Conditions: 0.2 mmol of 4-nitrobenzaldehyde, 6 mmol (30 equiv) of nitromethane, 2 mL of EtOH, 0.005 mmol ligand 1, 0.0055 mmol of Cu(OAc)2 2H2O, r.t.
b Isolated yield.
c Determined by HPLC with a chiral column (AD-H).
Screening of the amount of ligand 1 and nitromethane for the Henry reactiona.
Entry | Ligand 1 (mol%) | Cu(OAc)2 2H2Ob (mol%) | CH3NO2 (equiv) | Time (h) | Yieldc (%) | eed (%) |
1 | 1 | 1.1 | 30 | 72 | 90 | 88 |
2 | 2.5 | 2.75 | 30 | 48 | 98 | 93 |
3 | 5 | 5.5 | 30 | 48 | 98 | 93 |
4 | 7.5 | 8.25 | 30 | 48 | 98 | 93 |
5 | 10 | 11 | 30 | 48 | 98 | 92 |
6 | 12.5 | 13.75 | 30 | 48 | 97 | 91 |
7 | 15 | 16.5 | 30 | 48 | 98 | 92 |
8 | 2.5 | 2.75 | 1 | 48 | 95 | 71 |
9 | 2.5 | 2.75 | 5 | 48 | 93 | 82 |
10 | 2.5 | 2.75 | 10 | 48 | 99 | 87 |
11 | 2.5 | 2.75 | 20 | 48 | 98 | 88 |
12 | 2.5 | 2.75 | 30 | 48 | 95 | 91 |
13 | 2.5 | 2.75 | 40 | 48 | 99 | 90 |
a Conditions: 0.2 mmol of 4-nitrobenzaldehyde, 0.2–8 mmol (1–40 equiv.) of nitromethane, 2 mL of EtOH, r.t.
b The amount of Cu(OAc)2·2H2O changes with ligand 1 and it is equal to 1.1 times the amount of ligand 1 that would lead to the background reaction.
c Isolated yield.
d Determined by HPLC with chiral column (AD-H).
Screening of metal salts for the Henry reactiona.
Entry | Metal salt | Yieldb (mol%) | eec (%) |
1 | Cu(OAc)2·2H2O | 98 | 93 |
2 | CuSO4·5H2O | 55 | 25 |
3 | Cu(NO3)2·3H2O | 20 | 10 |
4 | CuCl | 80 | 81 |
5 | CuBr | 87 | 84 |
6 | CuBr2 | 85 | 78 |
7 | Cu(OTf)2 | 88 | 87 |
8 | FeCl3 | 45 | 47 |
9 | FeCl2 | 57 | 56 |
10 | ZnCl2 | 55 | 59 |
11 | Co(OAc)2·4H2O | 68 | 42 |
a Conditions: 0.2 mmol of 4-nitrobenzaldehyde, 6 mmol (30 equiv.) of nitromethane, 2 mL of EtOH, 0.005 mmol ligand 1, 0.0055 mmol of metal salt, r.t., 48 h.
b Isolated yield.
c Determined by HPLC with chiral column (AD-H).
In a word, the asymmetric Henry reaction was effectively catalyzed in a high yield and high enantioselectivity fashion from content values of 2.5 mol% of ligand 1, 30 equiv of nitromethane and 2.75 mol% of metal salt Cu(OAc)2·2H2O in ethanol.
After screening the optimized conditions for ligand 1, the scope of the substrate was extended. As listed in Table 5, different kinds of aromatic aldehydes, including those with electron-withdrawing and electron-donating groups were employed as substrates to react with nitromethane, giving the corresponding products with moderate to good yields (78–98%) and ee values (54–98%). Obviously, the electronic effect of the substituent on aromatic aldehydes had much influence on the enantioselectivities in this reaction. As shown in Table 5, aldehydes with electron-withdrawing substituents such as –NO2, –Br, –Cl, –F, and –CF3 (Table 5, entries 1–6) gave similar high ee values; especially that observed with 2-chlorobenzaldehyde could reach up to 98%. However, aldehydes with electron-donating substituents such as –OMe and –Me led to a significant decrease in both yield and ee value (Table 5, entries 8–10). Benzaldehyde as the simplest aromatic aldehyde can give a 80% yield with 89% ee (Table 5, entries 7), Moreover, other aromatic aldehydes (Table 5, entries 11–12) and aliphatic aldehyde (Table 5, entry 13) could also give yields as high as 85–88% and ee values of 89–90%, regardless of the branch on the chain. Therefore, ligand 1 could be applied with a wide scope of substrates for the asymmetric Henry reaction.
Enantioselective Henry reactions of various aldehydesa.
Entry | R | Yieldb (%) | eec (%) | Configd |
1 | 4-NO2C6H4 | 98 | 93 | S |
2 | 2-NO2C6H4 | 95 | 89 | S |
3 | 3-NO2C6H4 | 96 | 87 | S |
4 | 4-BrC6H4 | 97 | 94 | S |
5 | 2-ClC6H4 | 98 | 98 | S |
6 | 4-CF3C6H4 | 97 | 94 | S |
7 | C6H5 | 86 | 88 | S |
8 | 4-MeC6H4 | 78 | 54 | S |
9 | 4-MeOC6H4 | 78 | 64 | S |
10 | 2-MeOC6H4 | 83 | 78 | S |
11 | (E)-PhCH = CH | 88 | 90 | R |
12 | 87 | 89 | S | |
13 | CH3CH2 | 85 | 90 | S |
a Conditions: 0.2 mmol of 4-nitrobenzaldehyde, 6 mmol (30 equiv) of nitromethane, 2 mL of EtOH, 0.005 mmol of ligand 1, 0.0055 mmol of Cu(OAc)2·2H2O, r.t., 48 h.
b Isolated yield.
c Determined by HPLC with chiral column (AD-H or OD-H).
d The absolute configuration of the products was based on the measurement of the optical rotation and comparison with the literature values [20–23].
At last, the recyclability of catalyst 15 has been tested in the catalytic asymmetric Henry reaction. As shown in Table 6, catalyst 15 had a bad recyclability. Both the declined yield and the ee value had been obtained with increasing the reaction times. It indicated that catalyst 15 had a poor recyclability because of its serious loss.
The recyclability of catalyst 15 for the Henry reactiona.
Entry | Cycle index | Time (h) | Recovery ratio (%) | Yieldb (%) | eec (%)/S |
1 | Cycle 1 | 48 | 95 | 98 | 93 |
2 | Cycle 2 | 48 | 93 | 97 | 90 |
3 | Cycle 3 | 48 | 92 | 96 | 88 |
4 | Cycle 4 | 48 | 89 | 92 | 85 |
5 | Cycle 5 | 48 | 87 | 90 | 84 |
6 | Cycle 6 | 48 | 85 | 91 | 84 |
7 | Cycle 7 | 48 | 84 | 90 | 83 |
8 | Cycle 8 | 48 | 80 | 89 | 81 |
a Conditions: 0.2 mmol of 4-nitrobenzaldehyde, 6 mmol (30 equiv) of nitromethane, 2 mL of EtOH, 0.005 mmol catalyst 15, r.t.
b Isolated yield.
c Determined by HPLC with chiral column (AD-H).
2.2 Results and discussion about ligands 16a–f, 17
Although some small molecular ligands exhibited excellent solubility and inconvenient recovery properties, they appeared to have good catalytic effects. Based on its merit, the corresponding polymer-supported ligands have been synthesized immediately. Then these polymer-supported ligands were applied to research in the field of the asymmetric Henry reaction. The results are shown in Tables 7–11. As shown in Table 7, ligand 16a (Table 7, entry 1, 95% yield, 90% ee) was the optimized ligand among the polymer-supported ones. Then, further optimization of the reaction conditions of ligand 16a were tested in the asymmetric Henry reaction between 4-nitrobenzaldehyde and nitromethane. The results were summarized in Tables 8 and 9 The results clearly showed that protonic solvents were superior to non-protonic ones (Table 8). It was important to note that the protonic solvents behaved better than aprotic solvents in this reaction, especially ethanol. Raising the loading of ligand 16a (Table 9, entries 1–2) from 1 mol% to 2.5 mol% led to an increase in both of yields and the ee values. But, by raising further the loading of ligand 16a from 2.5 mol% to 12.5 mol% (Table 9, entries 3–6), the reaction gave almost the same yields and ee values. In a word, the optimized solvent was ethanol, the optimized amount of ligand was 2.5 mol%.
Screening of the ligands for the asymmetric Henry reactiona.
Entry | Ligand | Time (h) | Yieldb (%) | eec (%)/S |
1 | 16a | 48 | 95 | 90 |
2 | 16b | 48 | 92 | 87 |
3 | 16c | 48 | 91 | 88 |
4 | 16d | 48 | 91 | 89 |
5 | 16e | 48 | 90 | 88 |
6 | 16f | 48 | 92 | 85 |
a Conditions: 0.3 mmol of 4-nitrobenzaldehyde, 9 mmol (30 equiv) of nitromethane, 2 mL of EtOH, 2.5 mol% of ligands 16a–f, 2.75 mol% Cu(OAc)2·2H2O, r.t.
b Isolated yield.
c Determined by HPLC with chiral column (AD-H). The absolute configurations of the products were determined by comparison with literature [19,24] data.
Effect of the solvents of ligand 16a for the Henry reactiona.
Entry | Solvent | Time (h) | Yieldb (%) | eec (%) |
1 | EtOH | 48 | 95 | 90 |
2 | MeOH | 48 | 94 | 89 |
3 | i-PrOH | 48 | 92 | 87 |
4 | CH2Cl2 | 60 | 81 | 76 |
5 | THF | 48 | 85 | 82 |
6 | PhMe | 48 | 85 | 86 |
7 | CH3COOC2H5 | 48 | 82 | 85 |
8 | CH3CN | 48 | 80 | 81 |
a Conditions: 0.3 mmol of 4-nitrobenzaldehyde, 9 mmol (30 equiv) of nitromethane, 2 mL of solvent, 2.5 mol% ligand 16a, 2.75 mol% Cu(OAc)2·2H2O, r.t.
b Isolated yield.
c Determined by HPLC with chiral column (AD-H).
Screening of the amount of ligand 16a for the Henry reactiona.
Entry | Ligand 16a (mol%) | Cu(OAc)2·2H2Ob (mol%) | Time (h) | Yieldc (%) | eed (%)/S |
1 | 1 | 1.1 | 48 | 88 | 80 |
2 | 2.5 | 2.75 | 48 | 94 | 89 |
3 | 5 | 5.5 | 48 | 94 | 89 |
4 | 7.5 | 8.25 | 48 | 95 | 88 |
5 | 10 | 11 | 48 | 95 | 78 |
6 | 12.5 | 13.75 | 48 | 95 | 77 |
a Conditions: 0.3 mmol of 4-nitrobenzaldehyde, 9 mmol (30 equiv) of nitromethane, 2 mL of EtOH, r.t.
b The amount of Cu(OAc)2·2H2O changes with ligand 16a and it represents 1.1 times the amount of ligand 16a.
c Isolated yield.
d Determined by HPLC with chiral column (AD-H). The absolute configurations of the products were determined by comparison with literature [19,24] data.
Enantioselective Henry reactions of various aldehydesa.
Entry | R | Yieldb (%) | eec (%) | Config d |
1 | 4-NO2C6H4 | 94 | 89 | S |
2 | 2-NO2C6H4 | 86 | 76 | S |
3 | 3-NO2C6H4 | 87 | 78 | S |
4 | 4-BrC6H4 | 87 | 86 | S |
5 | 2-ClC6H4 | 89 | 88 | S |
6 | C6H5 | 76 | 78 | S |
7 | 4-MeC6H4 | 70 | 60 | S |
8 | 4-MeOC6H4 | 68 | 67 | S |
a Conditions: 0.3 mmol of arylaldehyde, 9 mmol (30 equiv) of nitromethane, 2 mL of EtOH, 2.5 mol% ligand 16a, 2.75 mol% Cu(OAc)2·2H2O, r.t., 48 h.
b Isolated yield.
c Determined by HPLC with chiral column (AD-H or OD-H).
d The absolute configuration of the products was based on measurements of the optical rotation and comparison with literature [20] data.
The recyclability of catalyst 17 for the Henry reactiona.
Entry | Cycle index | Time (h) | Recovery ratio (%) | Yieldb (%) | eec (%)/S |
1 | Cycle 1 | 48 | 98 | 94 | 90 |
2 | Cycle 2 | 48 | 98 | 93 | 90 |
3 | Cycle 3 | 48 | 98 | 94 | 89 |
4 | Cycle 4 | 48 | 97 | 92 | 88 |
5 | Cycle 5 | 48 | 97 | 91 | 89 |
6 | Cycle 6 | 48 | 96 | 91 | 88 |
7 | Cycle 7 | 48 | 95 | 90 | 87 |
8 | Cycle 8 | 48 | 95 | 90 | 86 |
a Conditions: 0.2 mmol of 4-nitrobenzaldehyde, 6 mmol (30 equiv) of nitromethane, 2 mL of EtOH, 2.5 mol% of 17, r.t.
b Isolated yield.
c Determined by HPLC with chiral column (AD-H). The absolute configurations of the products were determined by comparison with literature [19,24] values.
Next, with the above optimized conditions, the scope was extended to a larger domain of substrates, as shown in Table 10. Different kinds of aromatic aldehydes, including those with electron-withdrawing and electron-donating groups, were employed as substrates in the reaction with nitromethane, affording the corresponding products with excellent yields (68–98%) and ee values (54–98%). Obviously, the electronic effect of the substituent on aromatic aldehydes had much influence on enantioselectivities in this reaction. In addition, although the catalytic effect of the small molecule ligand 1 was a little better than that of the corresponding polymer-supported ligand 16a, both ligands 1 and 16a could be applied with a wide scope of substrates for the asymmetric Henry reaction. And copper(II) chiral tridentate Schiff-base polymer-supported complex catalyst 17 has been obtained by coordinating ligand 16a with Cu(OAc)2·2H2O.
At last, we had tested the recyclability of catalyst 17 in catalytic asymmetric Henry reaction. As listed in Table 11, catalyst 17 had a good recyclability. It could be reused six times at least with similar good yields and high ee values.
3 Conclusion
In conclusion, the results of the recycling experiment showed that in our optimized reaction conditions, (2.5 mol% ligand 1, 2.75 mol% Cu(OAc)2·2H2O, EtOH, room temperature, reaction duration 48 h), most kinds of aromatic aldehydes could give a higher yield and the corresponding selectivity. They evidenced also that this catalyst works with a wide scope of substrates. On the other hand, the yield and enantioselectivity of an asymmetric Henry reaction were enhanced by a modification of the substituent on the phenol ring of the Schiff base. It could be very useful for designing new effective catalysts for the Henry reaction. Thus, we chose ligand 1, 2, 6, 9, 10 and 14 to prepare six kinds of polymer-supported tridentate chiral Schiff-base ligands 16a–f. In addition, in order to compare the advantages and disadvantages of the small molecule catalysts and those of the polymer-supported ones, we synthesized the small-molecule copper complex catalyst 15. They were applied to the asymmetric Henry reaction with good yields and ee values. Non-polymer-supported ligands had better catalytic effect than polymer-supported ones, but the latter could be recycled by a simple filtration and the recovered catalyst could be reused. Copper complex catalyst 15 gave good yields and high ee values, but had a poor recovery rate because of its serious loss. However, catalyst 17 could be reused six times at least with similar high yield and ee values in the asymmetric Henry reaction. It is showed that this kind of catalyst may have a potential value for industrial application.
Acknowledgements
This work was financially supported by the Natural Science Foundation of Zhejiang Province (LY12B02016) and the State Key Laboratory Breeding Base of Green Chemistry–Synthesis Technology, Zhejiang University of Technology (China).