1 Introduction
Recently, silver nanoparticles have been of great interest and were hugely investigated due to their unique properties and possible applications in different areas and in particular in medicine as effective antibacterial agents. There are many infections caused by some common existing bacteria, such as Staphylococcus aureus [1] and Escherichia coli [2] in human beings. These bacteria-transmitted contagions have an impact on human health and result in making heavier the economic burden. Therefore, the inhibition of bacteria is an essential subject. The traditional physical and chemical bactericidal methods, including high temperature, ultraviolet and bactericide, have been used for several decades. The use of nanoparticles is a new method that leads to an effective inhibition of bacterial growth, especially when silver nanoparticles (AgNps) have been applied. AgNps have a high specific surface area, a high fraction of surface atoms, and have been widely investigated for their antimicrobial activity. The control over the size and shape as well as the stability of silver nanoparticles is the key to success [3]. However, to realize their potentialities in technological and biological applications, they should be entrapped in a polymer matrix, which can form thin or scaffolded films [4]. There are many investigations reporting on the preparation of silver nanoparticles embedded into different polymer matrices [5–9]. The use of polyvinyl alcohol as a film-forming polymer to embed silver nanoparticles is an appropriate choice, since the PVA is a hydrophilic, biocompatible, and water-soluble polymer [10]. These characteristics provide PVA with a wide application field in medicine and biochemistry. According to the structure of PVA, the large numbers of hydroxyl groups originated from PVA chain tend to form intermolecular and intramolecular hydrogen bonds, which endows them with more properties, such as mechanical, barrier, film-forming ones [11]. The use of a sol–gel process is a promising way to improve some PVA properties such as its waterproofness. The preparation of PVA/AgNps/TEOS and PVA/AgNps/APTEOS hybrid films has been recently reported in the literature [3,12]. However, no data were found for the preparation of hybrid materials based on PVA and 3-mercaptopropyltriethoxysilane (MPTES) with embedded silver nanoparticles. MPTES is a kind of organosilane that possesses thiol groups (–SH) in its structure; these groups can provide additional adsorption sites to keep the AgNPs stabilized as well as to prevent them from coagulating, thus leading to increased AgNps efficiency.
In this study, we present the preparation of a novel hybrid material based on PVA/MPTES with embedded AgNps via a sol–gel process. The obtained novel hybrid materials are expected to present high antibacterial activity toward Gram-positive S. aureus and Gram-negative E. coli and could find possible application in the biomedical field as antibacterial coating materials.
2 Materials and method
2.1 Materials
Poly (vinyl alcohol) (Aladdin; 87.0-89 hydrolyzed); HNO3 (Sinopharm Chemical Reagent Co. Ltd, 2 mol/L); AgNO3 (Sinopharm Chemical Reagent Co. Ltd, AR); γ-mercaptopropyltriethoxysilane (MPTES) (Sinopharm Chemical Reagent Co. Ltd) were used as received without further purification.
2.2 Methods
The UV–vis absorption spectra of a solution of silver nanoparticles were recorded at room temperature in the range from 200 nm to 800 nm using a UV-vis 8453 (Agilent, USA) device. Transmission electron microscopy (TEM) images and energy-dispersive X-ray analysis (EDX) were recorded on a JSM-6700F (JEOL Ltd, Japan). The samples for TEM observations were prepared by coating the carbon-coated grids with final solution containing PVA/AgNPs and hydrolyzing MPTES, which were then dried at room temperature. The final hybrid film was obtained by further annealing the samples at 100 °C for 1 h.
Histograms of size distribution were calculated from the TEM images by measuring the diameter of at least 50 particles.
The FTIR spectra of the films were recorded in transmittance mode in the range from 400 to 4000 cm−1 by Fourier transform infrared (FTIR) spectroscopy using an AVATAR 370 (Nicolet, USA) device. X-ray diffraction experiments were performed on XRD, XD-3 (Persee, PR China). The hybrid films were measured by wide-angle X-ray diffraction between 10° and 90° with a step of 0.01° using Cu Kα radiation (λ = 1.5406 Å).
2.3 Synthesis of PVA/AgNps/MPTES hybrid materials
Five grams of polyvinyl alcohol (PVA) were dissolved in 95 mL of deionized water while heating for 20 min at 80 °C. Different amounts of silver nitrate (0.1 and 0.05 g dissolved in 0.75 mL of water) were added to 25 mL of PVA (5%). The silver nanoparticles were prepared via thermal reduction by heating the solution at 100 °C for 1 h. The silica sol was produced by hydrolyzing MPTES (200 μm) in acidified ethanol (2 mL) using HNO3 as a catalyst. The mixture was stirred until a clear solution is obtained and subsequently added dropwise to the PVA/AgNps solution. The final mixture was stirred for 12 h and then cast into a film into PS plates. The films were dried for three days at room temperature and peeled off. Further annealing of the films was performed during 1 h at 100 °C.
2.4 Antibacterial analysis
The antibacterial activity of PVA/MPTES/AgNps was evaluated against E. coli and S. aureus.
To evaluate the growth inhibition of the production, the optical density of S. aureus and E. coli in the mixture of nutrient broth (0.295% beef extract, 0.982% peptone, 0.491% NaCl and 98.2% H2O; pH = 7.4) and PVA/AgNps/MPTES solution was detected. Each bacterium was inoculated into the nutrient solution containing 60 mL of nutrient broth and 2 mL of a PVA/AgNps/MPTES solution. The optical density was detected every 2 h under the 595 nm wavelength using a UV-vis 8453 (Agilent, USA) device during 24 h.
2.4.1 Measurement of the antibacterial activity of the films by disk-diffusion method
The disk-diffusion method has been used to evidence antibacterial activity. The 6-mm-diameter disks were made of qualitative filter paper and were impregnated with 5 μL of the PVA/AgNps/MPTES mixture. The impregnated disks were allowed to dry at room temperature for 24 h. Then they were put into the oven and dried at 100 °C for 1 h. According to the reference of the Clinical Laboratory and Standards Institute (CLSI) methods, the disks were located on the surface of a beef extract–peptone agar medium. After 36 h, the diameter of the inhibition zone was measured.
3 Results and discussion
Novel hybrid materials on the basis of PVA and MPTES with embedded silver nanoparticles were synthesized for the first time via a sol–gel method. The chemical structure and the way of preparation of PVA/AgNps/MPTES hybrid films are shown in Schemes 1 and 2. Initially, AgNps were prepared by boiling the PVA solution at 100 °C for 60 min in the presence of AgNO3 as a precursor of silver ions. The transformation of silver ions into silver nanoparticles has been performed via thermal reduction in the presence of PVA, which acts as a stabilizer that protects silver nanoparticles from agglomeration and as a reducing agent that transferred Ag+ into Ag0 nanoparticles (Scheme 1). In comparison to other techniques for the preparation of silver nanoparticles, this method is preferable because of the lack of unwanted by-products obtained as a result of the reduction process [13].
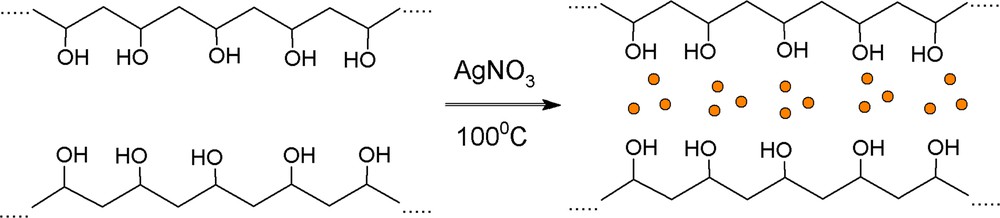
(Color online.) Preparation of silver nanoparticles stabilized by PVA.
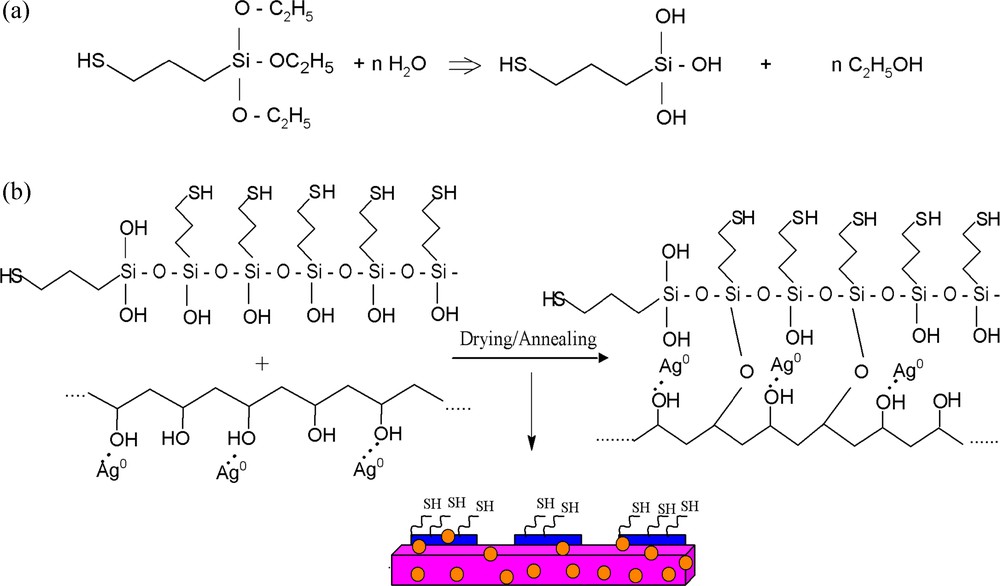
a: Hydrolysis of MPTES; b: preparation of PVA/AgNps/MPTES hybrid films.
To prepare PVA/AgNps/MPTES hybrid films, MPTES was first hydrolyzed in the presence of an acid catalyst (HNO3), which results in the formation of silanol groups (Scheme 2a). Thus the obtained hydrolyzed MPTES were further dispersed into PVA/AgNps solution where siloxane or Si-O-C bonds were formed through a dehydratation or dealcoholysis reaction during the polycondenzation process (Scheme 2b). Thus, film casting leads to the formation of silver nanoparticles embedded into a PVA/MPTES matrix.
The successful formation of thermally reduced silver nanoparticles stabilized by PVA was proven by UV-vis spectroscopy (Fig. 1). A strong absorption band at 420 nm for PVA/AgNps solutions at two different silver concentrations is observed, which is indicative of the formation of spherical silver nanoparticles [14,15].
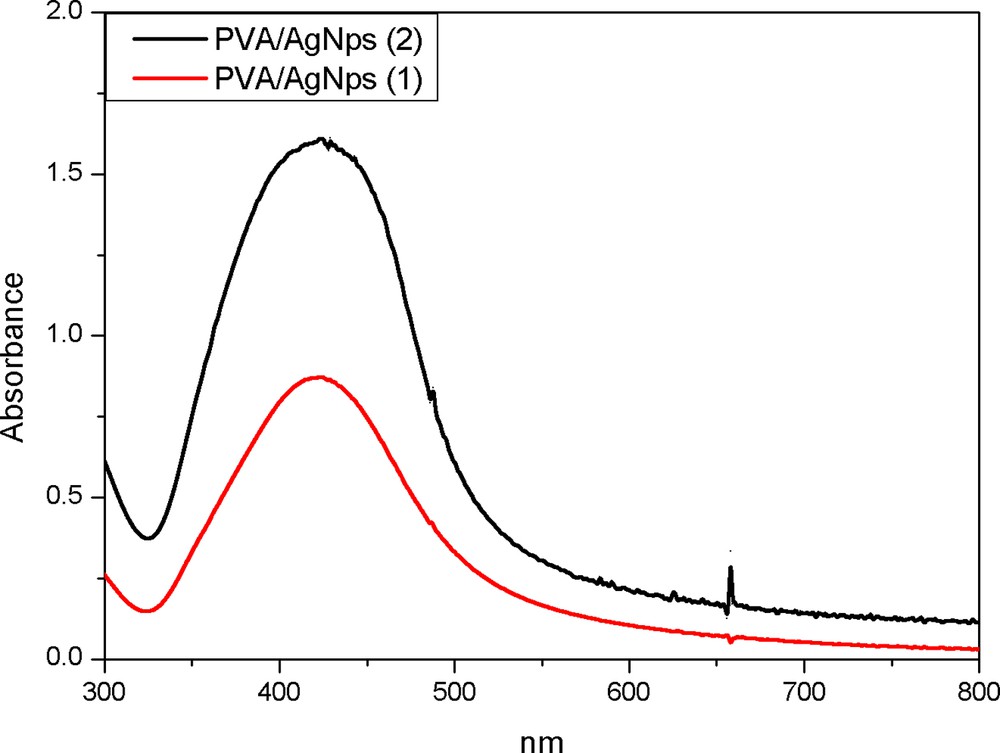
(Color online.) UV–vis spectroscopy for PVA/AgNps at two different silver concentrations: 1: 11.7 mM of silver precursor; 2: 23.5 mM of silver precursor.
The addition of MPTES to the PVA/AgNps leads to the formation of PVA/AgNps/MPTES hybrid materials originating from acid catalyzed hydrolysis and polycondensation of MPTES, the chemical structure of which was characterized by FTIR spectroscopy. Fig. 2 presents the FTIR spectra of pure PVA and PVA/AgNps/MPTES films. In both cases, a broad absorption peak centered at around 3300 cm−1 is attributed to the OH stretching vibration of hydrogen-bonded hydroxyl groups present in the samples as well as of uncondensed silanols (SiOH). The peaks at 1430 cm−1 and 1326 cm−1 are characteristic of the OH groups and CH deformation vibration, respectively, in PVA, and are present in all spectra. The absorption peak at 1000–1100 cm−1 can be assigned to CO stretching and OH bending vibrations arising from the PVA chain. In the same region, the asymmetric stretching vibration due to the SiOSi linkage as a result of the condensation reaction between hydrolyzed silanol SiOH groups is also presented. The SiOC bonds that can be formed as a result of chemical crosslinking of silanol groups (SiOH) and OH groups arising from PVA during the thermal annealing of the films appeared in the range from 1120 to 1080 cm−1, which overlaps the absorption interval of SiOSi bonds. In comparison to the pure PVA film, for PVA/AgNps/MPTES film, an absorption peak at 2353 cm−1, which is characteristic for MPTES, associated with the thiol (SH) vibration band, is observed.
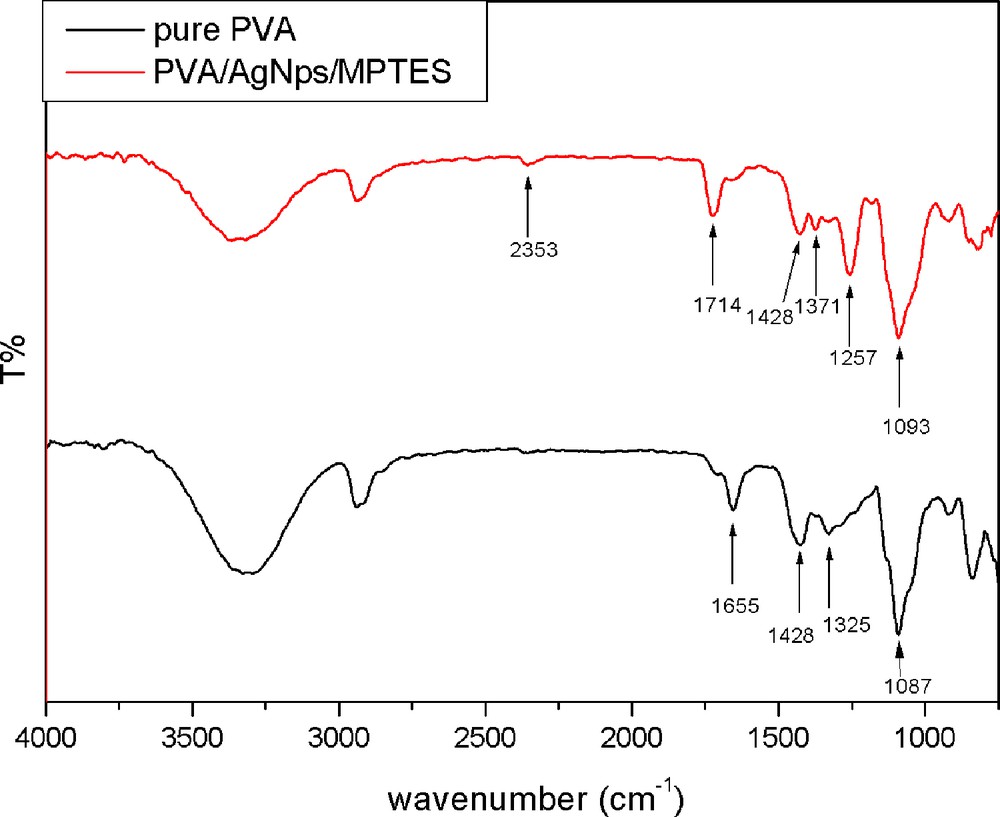
(Color online.) FTIR spectra of pure PVA film; PVA/MPTES matrix and PVA/AgNps/MPTES matrix.
The morphology and the size of AgNps embedded into PVA/MPTES matrices were observed by TEM. Fig. 3 shows TEM images of silver nanoparticles embedded into a PVA/MPTES matrix where spherical silver nanoparticles were detected. For a PVA/AgNps/MPTES matrix with a silver concentration of 11.7 mM, silver nanoparticles with an average diameter of 5.0 nm have been measured by TEM pictures (Fig. 3a). The TEM picture of PVA/AgNps/MPTES matrix with higher silver concentration (23.5 mM) showed the presence of individual silver nanoparticles as well as of a number of aggregates (Fig. 3c). The particle size histograms of silver nanoparticles illustrate the relatively narrow size distribution and an increase in the average diameter of the particles with increasing silver concentration (Fig. 3b,d).
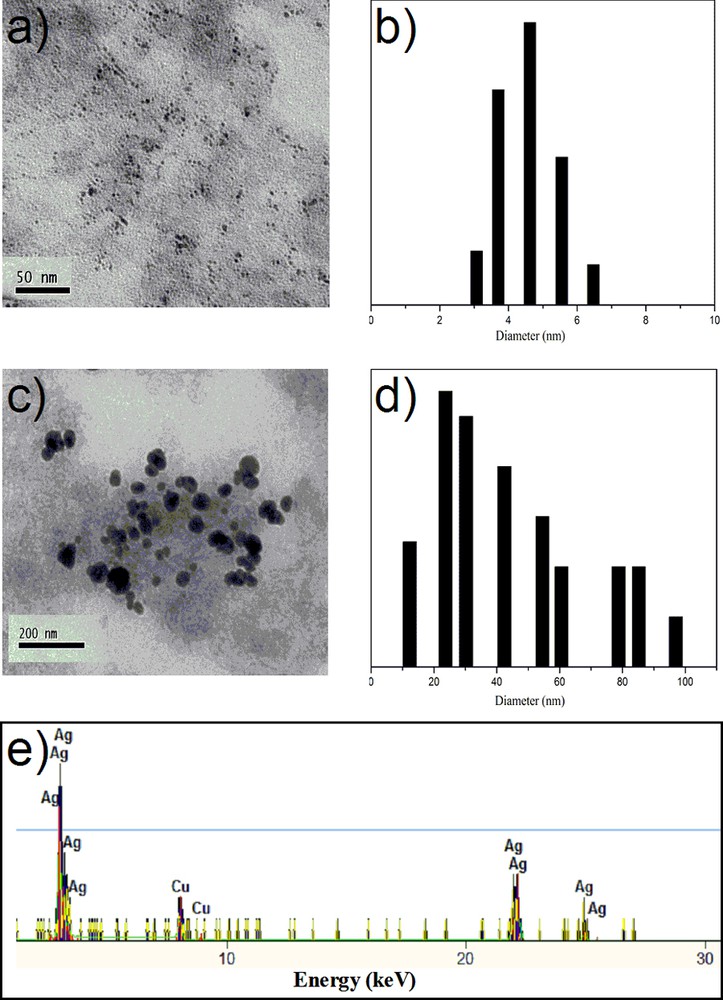
(Color online.) TEM and particle size distribution histograms of PVA/AgNps/MPTES films: a–b: silver concentration of 11.7 mM; c–d: silver concentration of 23.5 mM; e: EDX of PVA/AgNps/MPTES films (silver concentration of 23.5 mM).
To confirm the formation of silver nanoparticles, the elemental analysis was performed using EDX on the TEM (Fig. 3e). The figure shows the presence of peaks at around 3.40 keV, 22 keV and 25 keV, which correspond to the binding energies of Ag L, Ag Kα1 and Ag Kα2 [16]. The copper peaks correspond to the copper grids used for TEM analysis. No peaks of other impurities have been detected. A similar EDX spectrum was obtained for each analyzed sample.
Usually, X-ray scattering has been used as a technique for the investigation of the crystallinity of materials (crystalline phases, orientations, planes, and unit cell structure) [17]. Therefore, PVA/AgNps/MPTES hybrid materials have been characterized by XRD analysis. X-ray diffraction patterns (Fig. 4) of the studied hybrid materials show the appearance of a strong diffraction peak centered at 2θ = 19° of, which is attributed to the PVA crystalline phase [18]. A weak diffraction peak at 2θ = 38° is observed and is assigned to the (111) plane of the face-centered cubic (fcc) crystal structure of metallic silver, which confirms the formation of metallic silver nanoparticles [19]. The small size of the metallic particles can explain the weakness of the signal and the absence of other well-defined diffraction peaks characteristic of silver [20].
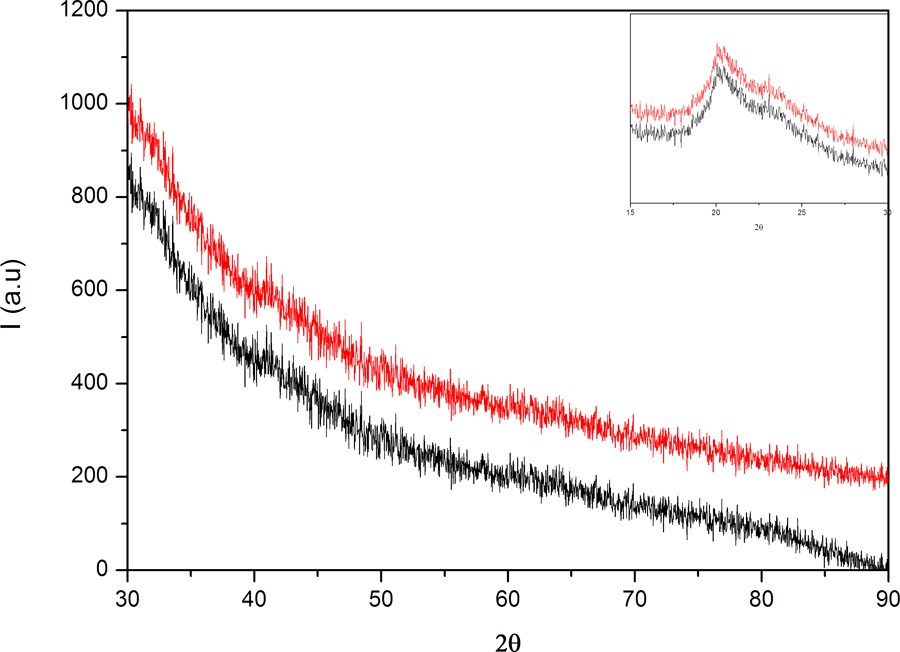
(Color online.) XRD patterns of PVA/AgNps/MPTES for different silver nanoparticle concentrations.
The crystalline nature of AgNps was further confirmed by selected-area electron diffraction (SAED) analysis. Fig. 5 illustrates SAED patterns of silver nanoparticles. The ring-like diffraction patterns indicate that the particles are crystalline. The diffraction rings corresponding to the {111} {200}, {220}, {311} and {331} planes of the face-centered cubic (fcc) silver structure were detected.
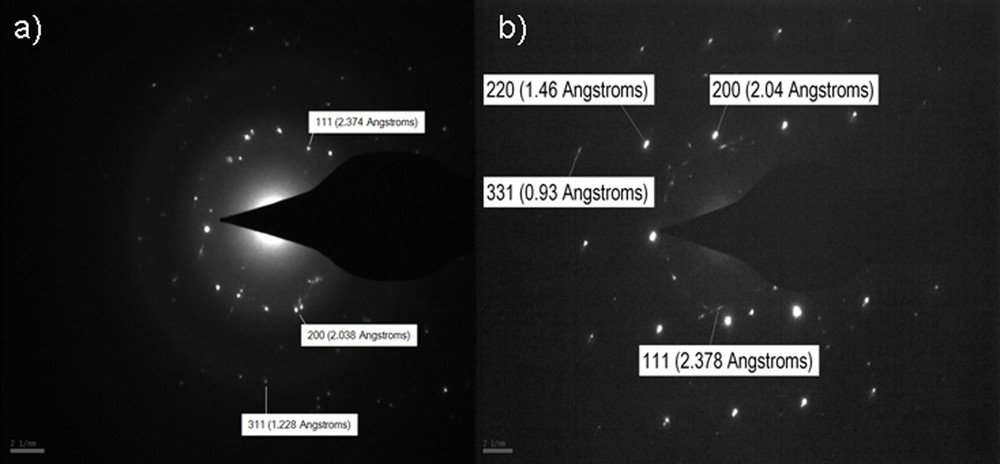
SAED patterns of PVA/AgNps/MPTES films: a: 11.7 mM silver concentration; b: 23.5 mM silver concentration.
The hybrid PVA/AgNps/MPTES/materials are tested for bactericidal activity against Gram-positive bacteria (S. aureus) and Gram-negative enteric bacteria (E. coli). It is well known that the membrane of the bacteria consists of many sulfur-containing proteins. Thus, the thiol-modified PVA hybrid films might ensure some specific mechanism of interaction with sulfur-containing proteins of bacteria through sulfursulfur interactions. On the other hand, these sulfur-containing proteins might be preferential sites for the silver nanoparticles. It is reported that the AgNps could combine with the (SH) groups of protein, then cause inactivity [21].
Initially, the antibacterial activity toward E. coli and S. aureus was tested by the disk diffusion method (DDM) by monitoring the zone of inhibition. It was found that PVA/AgNps/MPTES films exhibit bactericidal activity by the appearance of an inhibition zone against gram-positive bacteria (S. aureus) and gram-negative bacteria (E. coli); these zones are enhanced with increasing silver concentration (Fig. 6a–f).
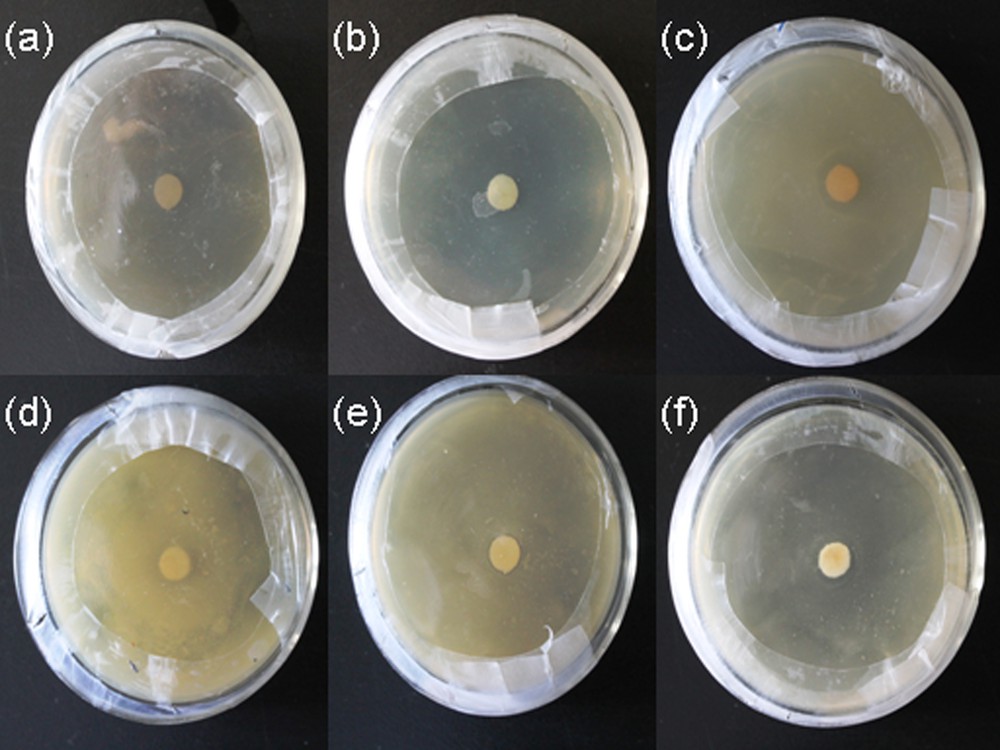
(Color online.) Disk diffusion method of PVA/AgNps/MPTES hybrid films against: a: E. coli – control sample PVA/MPTES film; b: E. coli–PVA/AgNps/MPTES film (11.7 mM of Ag precursor); c: E. coli–PVA/AgNps/MPTES film (23.5 mM); d: S. aureus – control PVA/MPTES sample; e: S. aureus – PVA/AgNps/MPTES film (11.7 mM of Ag precursor); f: S. aureus – PVA/AgNps/MPTES film (23.5 mM of Ag precursor).
However, the zones of inhibition of impregnated PVA/AgNps/MPTES disks against E. coli and S. aureus were relatively small. Therefore, the antibacterial activity against E. coli and S. aureus of the so-prepared PVA/AgNps/MPTES hybrid materials was studied via the growth curve method by measuring the optical density at 595 nm using a UV–vis spectrophotometer. Growth inhibition effects of PVA/AgNps/MPTES hybrid materials with two different silver concentrations were tested in nutrient solution for 24 h at 37 °C (Fig. 6a, b). The results showed that the PVA/AgNps/MPTES hybrid materials with lower silver concentration (11.7 mM) lead to an increase in the lag phase, as this effect was more pronounced for S. aureus population. However, further increasing silver concentration up to 23.5 mM in PVA/MPTES matrix leads to a significant reduction of the final population of E. coli and S. aureus within 24 h.
The results showed that in both cases the PVA/AgNps/MPTES films inhibit the bacterial growth of E. coli and S. aureus (Fig. 7) by decreasing the optical density, which means a reduced bacterial growth of E. coli and S. aureus.
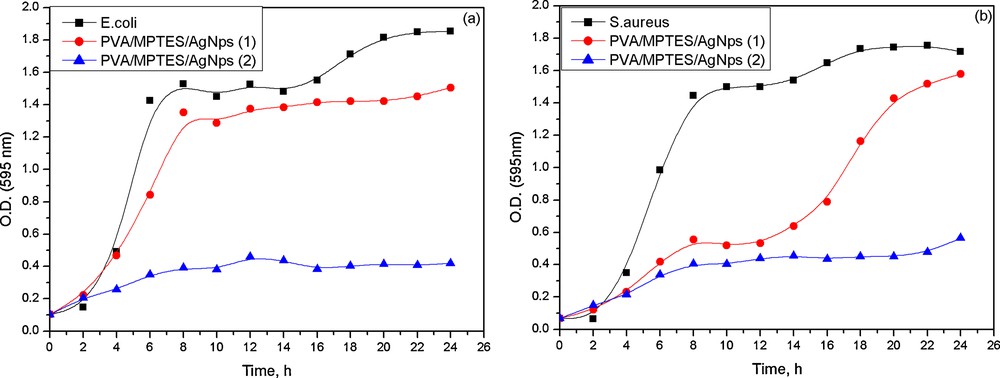
(Color online.) Growth curves of PVA/AgNps/MPTES hybrid materials against E. coli and S. aureus.
In PVA/AgNps/MPTES films with the higher silver concentration, there was almost no bacterial growth of E. coli and S. aureus, as the optical absorption was insignificant, which confirms the complete inhibition of bacterial growth. The results of the study of the antibacterial effects suggest that the obtained PVA/AgNps/MPTES hybrid materials can find potential applications in the biomedical field as effective antibacterial coatings.
4 Conclusion
Silver nanoparticles embedded in a PVA/MPTES matrix have been successfully synthesized for the first time via a sol–gel method. This has been achieved by the preliminary preparation of silver nanoparticles using PVA as a reducing agent via simple thermal reduction followed by addition of a silica precursor as MPTES. The formation of silver nanoparticles embedded into a PVA/MPTES matrix was proven by UV–vis, TEM, and XRD analysis. The obtained hybrid films showed high bactericidal activity against two different groups of bacteria — S. aureus (gram-positive bacteria) and E. coli (gram-negative bacteria) — whose effect enhances with increasing the silver content into the PVA/MPTES matrix. These results demonstrated that such hybrid materials could find potential applications in the biomedical area. Moreover, the presence of hydroxyl and thiol groups originating from PVA and MPTES would give a possibility to produce materials for biomolecular interactions having different applications.