1 Introduction
The traditional way of isolating volatile compounds as essential oils from lavender flowers (Lavandula hybribia L.) is distillation. During distillation, fragrant plants are exposed to boiling water or steam, releasing their essential oils. The recovery of the essential oil is facilitated by the density difference of water and essential oil at ambient temperature. Distillation is frequently done by prolonged heating for several minutes to hours, which can cause degradation of the thermo labile compounds present in the starting plant material and therefore odor deterioration. This conventional method has high consumption of energy (70% of total process energy) and time. Due to increasing energy prices and the drive to reduce CO2 emissions, academic and industrial scientists are challenged to find innovative technologies (Fig. 1) which can reduce energy consumption, achieve cost reduction and increased quality. Much attention has been devoted to the innovative processes such as the instant controlled pressure drop (DIC) technology, supercritical fluid extraction (SFE), ultrasound assisted extraction, microwave extraction, enzyme-assisted extraction, alternative solvents, for isolation essential oil from fragrant plants [1–13].
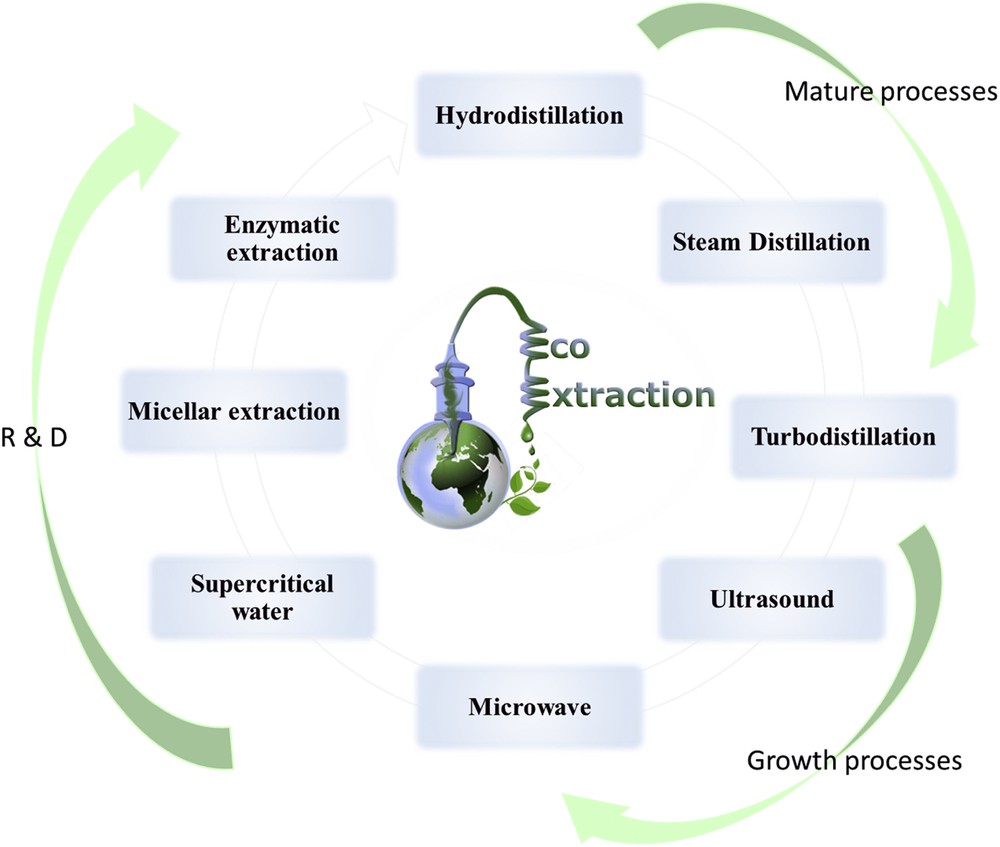
Processes for isolating essential oils from the plant material.
Besides, the use of water as a solvent has tremendous benefits as a green extraction solvent because water is not only inexpensive and environmentally benign; but it is also non-flammable, nontoxic, providing opportunities for clean processing and pollution prevention.
In addition to the environmental advantages of using water instead of organic solvents, purification of products is normally facilitated because, once cooled, the organic products are not soluble at ambient temperature water, which faster start-up, and simplification of process steps. However, the water is a polar solvent, with a density, a viscosity, and an activity which can impair solubility, the transfer or the contact with the matrix. Changing parameters and physical conditions of extraction, can overcome this problem.
The higher temperature of extraction can increase solvent-matrix interaction, but also transfer, solubility and diffusion. The macroscopic dielectric constant of a solvent (εr) characterizes the polarity of the medium and controls the ionic dissociation of salts. The dielectric constant of water decreases at high temperatures and pressures, which increases water diffusivity. Under these conditions, water is able to solubilize more non polar molecules [10].
Under soft conditions, it is possible to use water additives to assist extraction of the natural product. Another alternative approach to intensify essential oil release is the partial or complete hydrolysis of the cell walls by means of enzymes [14–16]. Surfactant molecules allow solubility enhancement of more hydrophobic molecules in water [17] and finally salt may be employed to enhance the concentration of certain components in the essential oil [18,19].
This present study has been planned with the aim to design and optimize a new and green technique for the extraction of essential oils from lavender (L. hybribia L.) flowers and possible improvement of its yield. Comparisons were investigated between nine extraction techniques and conventional HD as well as in terms of extraction time, yield, aromatic composition, energy used and environmental impact of the process. Finally, using COSMO-RS software was proposed to understand the extraction mechanism.
2 Materials and methods
2.1 Plant and chemical material
The lavender (L. hybribia L.) flowers used were collected from the south of France in July 2013 (Alp'erbo SARL, Manosque, France). Analytical grade anhydrous sodium sulfate and Tween 40 were purchased from Fisher Scientific (Leicestershire, UK). Cellulase from Aspergillus aqueous solution was purchased from Sigma Aldrich (Steinheim, Germany).
2.2 Extraction procedures
Hydro-distillation is the fundamental method for extraction of the lavender essential oil. This present study has been planned with the aim to design and optimize a new and green technique for the extraction of essential oils from lavender (L. hybribia L.) flowers and possible improvement of its yield.
The extraction of the essential oils from lavender was performed using ten different methods; hydro-distillation (HD), steam distillation (SD), turbo hydro-distillation (THD), pre-treatment with salt (Salt-HD), enzyme (Enzyme-HD), micelle (Micelle-HD), ultrasound (US-HD) or supercritical water (WS-HD) followed by conventional hydro-distillation and finishing with microwave assisted extraction techniques such as solvent-free microwave extraction (SFME) and microwave steam distillation (MSD) (Fig. 2).
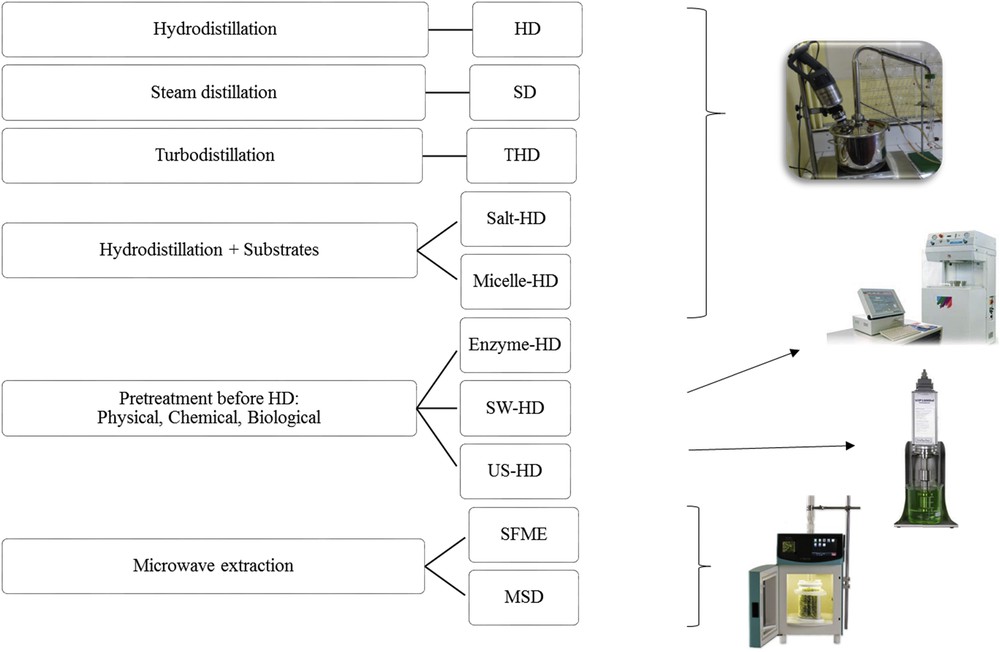
Extraction processes.
The appropriate experimental variables (amount of enzyme, surfactant, salt, and microwave power) for each process were optimized in order to maximize the essential oil yield.
2.2.1 Hydro-distillation (HD)
Two hundred fifty gram of lavender were mixed with 3 L of water. The mixture was submitted to hydro-distillation using a Clevenger-type apparatus [20] according to the European Pharmacopoeia for 2 h (until no more essential oil was obtained). The essential oil was collected, weighed, dried under anhydrous sodium sulfate and stored at 4 °C before analysis. Each extraction was performed at least three times.
2.2.2 Steam distillation (SD)
To facilitate rigorous comparison, the same glassware and the same operating conditions were used for conventional steam distillation. The specific glassware contained 3 L of distilled water and 250 g of lavender on a rack, so that there is no contact between water and lavender. The water was heated, the steam passed through the sample, evaporating and carrying the essential oil, and directed it toward the condenser and the Florentine flask. The oils obtained were recovered, weighed, dried over anhydrous sodium sulfate (Na2SO4) and stored in amber vials at 4 °C until required. Each extraction was performed at least three times.
2.2.3 Turbohydro-distillation (THD)
In this method, the same glassware and same conditions were used as that for hydro-distillation. The mixture was continuously agitated with a stainless steel stirrer at 100 rpm, for 2 h. The oils obtained were recovered, weighed, dried over anhydrous sodium sulfate (Na2SO4) and stored in amber vials at 4 °C until required. Each extraction was performed at least three times
2.2.4 Salt-assisted extraction followed by conventional hydro-distillation (Salt-HD)
Two hundred fifty gram of lavender were mixed with 3 L-distilled water containing 150 g of NaCl (values determined after optimization). The mixtures were subjected to hydro-distillation for essential oil isolation. To facilitate rigorous comparison, the same glassware and the same operating conditions were employed. The oils obtained were recovered, weighed, dried over anhydrous sodium sulfate (Na2SO4) and stored in amber vials at 4 °C until required. Each extraction was performed at least three times.
2.2.5 Enzyme-assisted extraction followed by conventional hydro-distillation (Enzyme-HD)
Two hundred fifty gram of lavender were mixed with 3 L-distilled water containing 12 g of cellulase from Aspergillus aqueous solution (Sigma–Aldrich) [21–25]. The mixtures were stirred for 60 min at 40 °C and then subjected to hydro-distillation for essential oil isolation. To facilitate rigorous comparison, the same glassware and the same operating conditions were employed. The oils obtained were recovered, weighed, dried over anhydrous sodium sulfate (Na2SO4) and stored in amber vials at 4 °C until required. Each extraction was performed at least three times.
2.2.6 Micelle-mediated extraction followed by conventional hydro-distillation (Micelle-HD)
Two hundred fifty gram of lavender were mixed with an aqueous surfactant solution containing 10% Tween 40. To facilitate rigorous comparison, the same glassware and the same operating conditions were employed. The oils obtained were recovered, weighed, dried over anhydrous sodium sulfate (Na2SO4) and stored in amber vials at 4 °C until required. Each extraction was performed at least three times.
2.2.7 Ultrasound assisted extraction followed by hydro-distillation (US-SD)
The ultrasound-assisted extraction was performed using an ultrasound extraction reactor (BS2d34, Hielscher UIP 1000hd). Lavender was extracted with 3 L of distilled water with an ultrasound of 700 W. The extractor and water were regulated at 25 °C during sonication. After the pre-treatment of 1800 s, conventional hydro-distillation was performed to extract the essential oil with the same glassware. The oils obtained were recovered, weighed, dried over anhydrous sodium sulfate (Na2SO4) and stored in amber vials at 4 °C until required. Each extraction was performed at least three times
2.2.8 Subcritical water extraction followed by conventional hydro-distillation (WS-HD)
Ultraclave is a device of Milestone Company. This is a 2.45 GHz multimode microwave reactor with a maximum power of 1200 W delivered in 10 W increments. During experiments, the time, temperature, and power delivered by microwave and internal pressure were controlled by the software. A compressor connected to a nitrogen cylinder conditioned initial pressure. The ultraclave supports a maximum pressure of 200 bar and a temperature of 220°. A cooling system is coupled to the cavity. The opening system may be at atmospheric pressure with a temperature below 80 °C. The vessel containing 250 g lavender mixed with 3 L of water, after having been degassed by ultrasound, was placed in the cavity. The initial working pressure of nitrogen was 30 bar. The working temperature of 125 °C is reached with a heating power of 1000 W. The temperature increase is achieved in 15 min, and then it is regulated for 30 min. The pretreated lavender was subjected to conventional hydro-distillation to extract essential oils with the same glassware. The oils obtained were recovered, weighed, dried over anhydrous sodium sulfate (Na2SO4) and stored in amber vials at 4 °C until required. Each extraction was performed at least three times.
2.2.9 Solvent-free microwave extraction (SFME)
SFME was performed in a NEOS (Milestone, Italy) microwave laboratory oven. This is a 2.45 GHz multimode microwave reactor with a maximum power of 900 W delivered in 10 W increments. The time and power of microwaves are selected. The experimental SFME variables were optimized in order to maximize the essential oil yield. This procedure was performed at atmospheric pressure. A condenser placed outside a microwave was connected to specific glassware that contained 125 g of lavender after having been soaked in 500 mL of distilled water for 10 min. Lavender was heated using a fixed power of 500 W for 45 min. Condensed water was refluxed to the extraction vessel in order to provide uniform conditions of temperature and humidity for the extraction. Essential oil and aromatic water were simply separated by decantation in a Florentine flask. The oils obtained were recovered, weighed, dried over anhydrous sodium sulfate (Na2SO4) and stored in amber vials at 4 °C until required. Each extraction was performed at least three times.
2.2.10 Microwave steam distillation (MSD)
To facilitate rigorous comparison, the same equipment and the same conditions: time and power were used. The process is based on the conventional steam distillation principle in which microwave radiation is only applied on the extraction reactor. The use of water steam generated within the vessel requires that the lavender (125 g) be supported above some boiling water by a grid. The water was heated, the steam passed through the sample, evaporating and carrying the essential oil, and directed it toward the condenser and a Florentine flask. The essential oil was collected, weighed, dried under anhydrous sodium sulfate and stored at 4 °C until used. Each extraction was performed at least three times.
2.3 Computational methods: COSMO-RS software
The Conductor-like Screening Model for Real Solvents (COSMO-RS) developed by Klamt and co-workers is known as a powerful method for molecular description based on a quantum-chemical approach. COSMO-RS combines quantum chemical considerations (COSMO) and statistical thermodynamics (RS) to determine and predict thermodynamic properties without experimental data. COSMO-RS is a relatively new prediction method for solubility and other physical–chemical properties. The theory COSMO-RS is previously given in several papers [21–25]. A brief sketch of the practical course of a COSMO-RS is considered here. The calculation has been investigated for three molecules of interest (linalyl acetate, linalool, and 4-terpineol), using the cosmotherm program, (Version C30 Release 13.01). The COSMO model is applied to simulate a virtual conductor environment for the molecule of interest. In such an environment the molecule induces a polarization charge density σ on the interface between the molecules and the conductor, i.e. on the molecular surface. Thus, during the quantum calculation self-consistency algorithm the solute molecule is converged to its energetically optimal state in the conductor with respect to electron density and geometry. The standard quantum chemical method for COSMO-RS is density functional theory (DFT), which is used throughout this study. The output of a DFT/COSMO calculation is a file providing this optimized geometry of the molecule, the total energy of the molecule in its conductor environments, and complete three-dimensional information about the polarization charge density: σ-surface. These calculations act as molecular information input for used the model to real solvents. The σ-surface of each molecule X is converted into a distribution function (σ-profile). The full equations are given in Klamt [22,23]. Finally, the chemical potential of compound X in system S is calculated by integration, which gives histogram σ-potential. The chemical potential can now be used to calculate a wide variety of thermodynamic properties, such as the solubility and energy of the reaction. The solubility option allows for the automatic computation of the solubility of a liquid or solid compound j in a solvent i. Within the calculation all compounds are also considered solutes. This approach is optimized for the calculation of many solutes in a limited number of solvents, at a specific temperature or in a temperature range. Here, the values of the solubility of each interest molecules have been calculated in water in the temperature range: 25–150 °C (Fig. 6). It is important to note that the values obtained are relative, not absolute. The reaction panel basically allows calculating the equilibrium constant (Kreac) and the free energy (ΔGreac) of a given reaction in an arbitrary solvent. The Gibbs free energy of reaction is defined as difference of the total free energies of the product compounds and the reactant compounds.

Solubility as a function of water temperature.
For an example of reaction: linalool → 4-terpineol, the free energy is defined as:
The equilibrium constant of the reaction can be computed from the Gibbs free energy of the reaction:
In each calculation, the solvent, the temperature and both sides of the reaction have to be specified. The standard approach gives a prediction relative to a reaction energy.
2.4 GC and GC–MS identification
2.4.1 Gas chromatography by using a flame ionic detector (FID)
GC analysis was carried out using an Agilent 6850 gas chromatograph, under the following operation conditions: vector gas, helium; injector and detector temperatures, 250 °C; injected volume, 1 μl; split ration 1/100; HP1 column (J&W Scientific), polydimethylsiloxane (10 m × 1 mm i.d., film thickness × 0.4 μm; constant flow 0.3 mL/min). Temperature program 60–250 °C at 4 °C/min and 250 °C for 80 min. Retention indices were determined with C6 to C27 alkane standards as references. Relative amounts of individual components are based on peak areas obtained without FID response factor correction. Three replicates were performed for each sample. The average of these three values and the standard deviation were determined for each component identified.
2.4.2 Gas chromatography-mass spectrometry analysis
GC–MS analysis was carried out using an Agilent 6890N coupled to an Agilent 5973 MS (Agilent, Massy, France). Each sample has been diluted at 10% in dichloromethane. Samples were analyzed on a fused-silica capillary column HP-1MS™ (polydimethylsiloxane, 50 m × 0.25 mm i.d. × film thickness 0.25 μm; Interchim, Montluçon, France) and INNOWAX (polyethyleneglycol, 50 m × 0.20 mm i.d. × film thickness 0.4 μm; Interchim, Montluçon, France). Operation conditions: carrier gas, helium; constant flow 1 mL min−1; injector temperature, 250 °C; split ratio, 1:150; temperature program, 45 °C–250 °C or 230 °C, at 2 °C/min then held isothermal (20 min) at 250 °C (apolar column) or 230 °C (polar column); ion source temperature, 230 °C; transfer line temperature, 250 °C (apolar column) or 230 °C (polar column); ionization energy, 70 eV; electron ionization mass spectra were acquired over the mass range of 35–400 amu.
2.4.3 Identification of the components
Identification of the components was based on computer matching against commercial libraries (Wiley, MassFinder 2.1 Library, NIST98), laboratory mass spectra libraries built up from pure substances, and MS literature data [8,26,27] combined with a comparison of GC retention indices (RI) on apolar and polar columns reported in the literature. RIs were calculated with the help of a series of linear alkanes C6–C27 on apolar and polar columns (HP-1MS™ and HP-INNOWAX). Compounds available in the laboratory were confirmed by external standard compound co-injection.
2.5 Sensory analysis
Sensory evaluation was used to discriminate the intensities of the main aromatic characteristics of lavender samples. Each essential oil was evaluated olfactorically by C. Louis (Perfurmer from Naturex, France). Ten different coded samples of essential oil of lavender obtained by different techniques have been classified in 12 odor attributes: floral lavender, herbaceous, camphoraceous, woody, spicy pepper, fruity, fatty/aldehydic, sweet/“candy”, soapy, off-note, intensity and persistence. Sample HD were used as standards. The individual products were scored for the intensity of different aroma attributes on a scale of 0–3, where:
- 0: not present
- 1: slight intensity
- 2: average intensity
- 3: high intensity
The essential oil of lavender has been prepared with 10% of ethanol, in plastic beakers with lids, which stood at room temperature for 30 min prior to analysis.
3 Results and discussion
3.1 Kinetics
Fig. 3 shows the variation in extraction yield according to extraction time. The yield is defined as the percentage of weight of the essential oil extracted from the initial mass of lavender flowers used. Hydro-distillation (4.55 ± 0.16%) was the reference method in essential oil isolation. The yields obtained from steam distillation (4.49 ± 0.06) and enzyme-HD (4.58 ± 0.14) were of the same order of magnitude as that of conventional hydro-distillation. Turbo-distillation (THD) and supercritical water pre-treatment (SW-HD) do not have a positive effect on extraction kinetics and give a maximum yield of 4.2 ± 0.36% and 3.89 ± 0.07% respectively, versus 4.55 ± 0.16% by HD. An increase of the extraction temperature to 100 °C at 125 °C by the SW-HD method shows a decrease of the extraction efficiency. Thus, a prolonged exposure time to each heating temperature appears to decrease terpene stability [28].

Yield profile of essential oil obtained via various extraction methods using water under different physical states (a) extraction methods with additivated water (b).
Otherwise, it is interesting to note that ultrasound pre-treatment of added salt or Tween 40 at hydro-distillation shows an improvement of the extraction. Indeed, US-HD, Salt-HD and Micelle-HD provide yields of 5.01 ± 0.45%, 5.06 ± 0.35% and 4.88 ± 0.07%, respectively, against 4.55 ± 0.16% by the conventional method. The cavitation process that occurs during sonication causes the rupture of cell walls, which enhances extraction essential oil. The use of salt in water allows improving the recovery of some volatile constituents from floral waters.
The presence of surfactant micelles of Tween 40 in water reduced the surface of tension between oil on the flowers and the distillation water; this explains the increase in the extraction yield.
One of the advantages of the extraction assisted by microwave is rapidity. The extraction process by microwave allows having 80% of final yield in 20 min. The end of the extraction process is reached after 30 min for the microwave process and 90 min using HD. Furthermore, the values obtained for essential oil yield are 3.9 ± 0.3% and 5.4 ± 0.5% for SFME and MSD respectively, versus 4.5 ± 0.2%. Based on these initial results, the MSD extraction appears to be promising.
3.2 Composition of essential oil
A total of 47 major compounds (in agreement with the literature) were identified in the lavender essential oil (Table 1). The lavender flower essential oils extracted using the different methods are all rather similar in their flavor profile. The principal volatile compounds are linalool and linalyl acetate followed by minor amounts of camphor, borneol, 1.8 cineole, lavandulyl acetate, 4-terpineol and α-terpineol; however, their proportions depend on the isolation technique used.
Chemical composition of lavender essential oils obtained by different techniques.
Compounda | RIb | HD (%) | SD (%) | THD (%) | SFME (%) | MSD (%) | US-HD (%) | SW-HD (%) | Salt-HD (%) | Enzyme-HD (%) | Micellar-HD (%) |
α-Thujene* | 923 | 0.1 | 0.1 | 0.1 | 0.1 | ||||||
α-Pinene* | 930 | 0.2 | 0.2 | 0.4 | 0.2 | 0.1 | 0.2 | 0.5 | 0.5 | 0.4 | 0.4 |
Camphene* | 942 | 0.1 | 0.2 | 0.2 | 0.1 | 0.1 | 0.2 | 0.4 | 0.4 | 0.4 | 0.4 |
1 Octen-3-ol* | 964 | 0.2 | 0.2 | 0.1 | 0.3 | 0.3 | 0.2 | 0.2 | 0.2 | 0.2 | 0.2 |
Sabinene* | 965 | 0.1 | 0.1 | 0.1 | 0.1 | 0.2 | 0.2 | 0.1 | |||
β-Pinene* | 969 | 0.2 | 0.2 | 0.2 | 0.1 | 0.1 | 0.2 | 0.4 | 0.4 | 0.3 | 0.3 |
β-Myrcene* | 983 | 1.1 | 1.1 | 1.0 | 0.7 | 0.5 | 1.0 | 1.5 | 1.3 | 1.4 | 1.4 |
n-Hexylacetate* | 995 | 0.2 | 0.2 | 0.1 | 0.1 | 0.1 | 0.1 | 0.2 | 0.2 | 0.2 | |
α-Terpinene* | 1008 | 0.1 | 0.1 | 0.1 | |||||||
Pcymene* | 1011 | 0.1 | 0.2 | 0.3 | 0.2 | 0.2 | 0.3 | 0.5 | 0.1 | 0.1 | 0.1 |
1.8 Cineol* | 1020 | 3.8 | 4.5 | 4.3 | 4.0 | 3.5 | 4.9 | 4.6 | 5.5 | 5.6 | 5.4 |
Limonene* | 1021 | 0.5 | 0.5 | 0.6 | 0.3 | 0.2 | 0.5 | 0.7 | 0.7 | 0.7 | 0.7 |
(Z) β-Ocimene* | 1027 | 0.8 | 0.8 | 0.4 | 0.3 | 0.2 | 0.5 | 0.4 | 0.9 | 0.9 | 0.9 |
(E)β-Ocimene* | 1038 | 0.8 | 0.7 | 0.4 | 0.3 | 0.2 | 0.6 | 0.4 | 0.8 | 0.9 | 0.9 |
γ-Terpinene* | 1049 | 0.1 | 0.1 | 0.2 | 0.3 | 0.2 | 0.2 | 0.2 | |||
(Z) Linalool oxyde* | 1058 | 0.5 | 0.6 | 0.5 | 0.5 | 0.5 | 0.5 | 0.6 | 0.6 | 0.7 | 0.7 |
(E)Linalool oxyde* | 1072 | 0.3 | 0.4 | 0.3 | 0.5 | 0.5 | 0.4 | 0.5 | 0.5 | 0.5 | 0.5 |
Terpinolene* | 1078 | 0.3 | 0.3 | 0.4 | 0.2 | 0.5 | 0.5 | 0.5 | |||
Linalol* | 1094 | 30.0 | 28.1 | 30.9 | 27.1 | 24.0 | 33.6 | 27.5 | 29.6 | 32.7 | 31.5 |
1-Octenyl-3-acetate* | 1097 | 0.4 | 0.4 | 0.3 | 0.4 | 0.4 | 0.4 | 0.3 | 0.4 | 0.4 | 0.4 |
Camphor* | 1124 | 7.2 | 7.5 | 7.3 | 7.8 | 7.0 | 8.2 | 7.4 | 7.6 | 8.3 | 8.0 |
n-Hexylisobutyrate* | 1134 | 0.2 | 0.2 | 0.2 | 0.1 | 0.2 | 0.2 | 0.3 | 0.3 | 0.3 | |
Borneol* | 1151 | 4.5 | 4.2 | 3.9 | 4.2 | 4.1 | 1.5 | 4.5 | 4.0 | 4.3 | 4.4 |
Lavandulol* | 1152 | 0.8 | 0.7 | 0.8 | 0.8 | 0.8 | 0.9 | 4.8 | 0.7 | 0.7 | 0.8 |
4-Terpineol* | 1163 | 4.5 | 4.5 | 4.2 | 4.4 | 4.2 | 4.7 | 5.2 | 4.5 | 4.8 | 4.7 |
α-Terpineol* | 1174 | 6.2 | 4.7 | 4.8 | 1.8 | 1.8 | 6.2 | 10.2 | 5.3 | 6.4 | 6.5 |
Nerol* | 1212 | 1.0 | 0.6 | 0.4 | 0.4 | 0.1 | 0.8 | 1.8 | 0.8 | 0.9 | 1.0 |
Methyl-2-butyrate hexyl * | 1224 | 0.2 | 0.2 | 0.6 | 0.2 | 0.1 | 0.2 | 0.2 | 0.1 | ||
Methyl-3-butyrate hexyl | 1230 | 3.4 | 1.5 | 0.2 | 0.2 | 0.2 | 2.5 | 0.2 | |||
Geraniol* | 1240 | 2.1 | 5.2 | 2.7 | 3.2 | ||||||
Linalylacetate* | 1244 | 13.7 | 21.8 | 19.4 | 30.1 | 29.6 | 16.1 | 1.2 | 15.3 | 12.3 | 11.3 |
Lavandulylacetate* | 1273 | 3.8 | 3.7 | 3.4 | 3.3 | 3.5 | 3.6 | 3.7 | 3.5 | 3.3 | 3.2 |
Tiglatehexyle* | 1309 | 0.2 | 0.2 | 0.2 | 0.2 | 0.3 | 0.2 | 0.2 | 0.2 | 0.2 | 0.2 |
Nerylacetate* | 1342 | 1.5 | 1.1 | 1.1 | 0.4 | 0.3 | 1.4 | 2.1 | 1.2 | 1.4 | 1.4 |
Geranylacetate* | 1361 | 2.8 | 1.9 | 2.1 | 0.8 | 0.8 | 2.6 | 3.9 | 2.3 | 2.6 | 2.5 |
β-Funebrene* | 1406 | 0.1 | 0.1 | 0.1 | 0.1 | 0.1 | 0.1 | 0.1 | |||
β-Caryophyllene* | 1416 | 1.6 | 1.9 | 2.2 | 1.6 | 2.3 | 0.8 | 1.4 | 1.1 | 0.9 | 1.2 |
β-Bergamotene* | 1431 | 0.1 | 0.2 | 0.2 | 0.2 | 0.3 | 1.0 | 0.1 | 0.1 | ||
(E,E)-Farnesene* | 1446 | 1.2 | 1.4 | 1.8 | 1.4 | 2.0 | 0.6 | 0.7 | 0.8 | 0.6 | 0.9 |
Germacrene D* | 1474 | 0.6 | 0.6 | 0.3 | 0.2 | 0.3 | 0.1 | 0.4 | 0.3 | 0.4 | |
Linalylisovalerate | 1493 | 0.7 | 0.7 | 0.8 | 0.6 | 0.9 | 0.4 | 0.5 | 0.4 | 0.4 | |
β-Bisabolene* | 1499 | 0.2 | 0.2 | 0.3 | 0.3 | 0.4 | 0.1 | 0.1 | 0.2 | ||
γ-Cadinene* | 1504 | 0.3 | 0.3 | 0.4 | 0.4 | 0.6 | 0.1 | 0.4 | 0.3 | 0.2 | 0.2 |
β-Sesquiphellandrene | 1513 | 0.2 | 0.3 | 0.2 | 0.4 | 0.2 | 0.1 | 0.2 | 0.1 | 0.2 | |
Caryophylleneoxide* | 1568 | 0.4 | 0.3 | 0.6 | 0.7 | 1.1 | 0.4 | 0.7 | 0.4 | 0.3 | 0.3 |
α-Cadinol* | 1634 | 1.4 | 1.0 | 1.1 | 0.9 | 1.3 | 1.0 | 1.5 | 1.4 | 0.8 | 0.9 |
α-Bisabolol* | 1666 | 3.0 | 2.2 | 2.3 | 2.0 | 2.9 | 2.1 | 3.1 | 3.0 | 1.6 | 1.9 |
a Essential oil compounds sorted by chemical families.
b Retention indices relative to C6–C27 n-alkanes calculated on non-polar HP1MS™ capillary column.
As Table 1 shows, even if these samples seem to be quite similar, they are different considering only their mean in some oxygenated compounds such as linalyl acetate, linalool and 4-terpineol. The mean percentage of linalyl acetate is slightly higher than that of linalool for the extraction assisted by microwave and steam distillation but the contrary is significantly observed in all other techniques (Fig. 4). These differences are probably due to the degradation of linalyl acetate (when in contact with water) into linalool (Fig. 5). In SD, SFME and MSD, lavender is not directly in contact with water like in HD, consequently, the degradation of linalyl acetate is less marked than in HD [29]. The essential oil obtained by microwave shows higher values of linalyl acetate and lower values than by steam distillation of linalool.

Main compounds of the essential oil.

Formation of linalool and 4-terpineol.
For extraction Micelle-HD the mean percentage of linalool was high and the percentage to 4-terpineol was slightly above the hydro-distillation. The linalool molecule is unstable and it can transformed into 4-terpineol (Fig. 5). The calculation of the free enthalpy (Greac) and the constant (Kreac) of the reaction has been performed at 100 °C with COSMOtherm program. As shown in Fig. 4b, linalool rearrangement of 4-terpineol presents a negative value of Greac and a greater value of Kreac. At 100 °C, the reaction is spontaneous and total. Theory data confirm rearrangement hypothesis.
The same observations can be made for results found with THD, enzyme-HD and US-HD.
Furthermore, SW-HD shows a mean percentage of linalool higher than linalyl acetate, which can be due to the degradation, as seen previously. This extract presented also a significant amount of 4-terpineol. This percentage may be explained by the rearrangement of linalool in 4-terpineol but also by the increase extraction temperature. Indeed, calculation of solubilities with Cosmotherm program, of three interest molecules, at temperatures ranging from 25 to 150 °C (in step of 25 °C) shows a better solubilization (Fig. 6). The influence of the temperature on aqueous solution solubility has a significant effect on 4-terpineol, followed by linalool and a less effect on linalyl acetate. This may also explain the levels of these molecules in the extracts of SW-HD.
3.3 Environment impact, cost and upscaling
Extractions assisted by microwave are clearly advantageous in terms of time and energy (Fig. 7). The energy required to perform the two extraction methods are 3.452 kW h−1 per gram of essential oil for HD and 0.298 kW h−1 per gram of essential oil for microwave. The power consumption was determined with a Wattmeter at a microwave generator entrance and an electrical heater power supply. Hydro-distillation requires an extraction time of 120 min to heat the water and plant material to the extraction temperature, while the microwave methods require only 30 min of lavender flower heating (SFME) or to heat the water (MSD).

Environmental analysis.
Regarding environmental impact, the calculated quantity of carbon dioxide released into the atmosphere is dramatically higher in HD (2762 g CO2/g of essential oil) than by microwave extraction (238 g CO2/g of essential oil). These calculations have been made accordingly to obtain 1 kWh from coal or fuel, 800 g of CO2 will be rejected in the atmosphere during combustion of fossil fuel [30].
For the extraction, US-HD and SW-HD add an energy consumption at HD of 0.16 kW h and 1.28 kW h, respectively. In other terms, ultrasound rejected 128 g of CO2, ultraclave pre-treatment rejected 1024 g of CO2. The use of the enzyme or surfactant decreases energy consumption of 5.05% and 13.05%, respectively, versus the conventional method.
Microwave extraction is a very clean method, which allows essential oil extraction without being followed by hydro-distillation. SFME or MSD generate fresh aromatic flower “without essential oil” which could be used as food for ruminants. The SFME method has an additional advantage versus MSD: step of wastewater treatment is not needful.
Furthermore, microwave and ultrasound become mature and accepted technologies for industrial applications. Microwave and ultrasound extractions could be used to produce larger quantities of essential oils by using existing large-scale extraction reactors treating for 10–100 kg of aromatic herbs and spices [31–37].
3.4 Sensory analysis
The sensory analysis results are presented in Fig. 8. The main notes of aromatic lavender namely “floral lavender”, “herbaceous”, “camphoraceous”, “fruity citrus/bergamot” are generally well represented in all samples. It is interesting to note that SW-HD, SFME, MSD and Micelle-HD essential oils give the most typical profiles: “fruity citrus/bergamot” with sides “fatty/aldehyde” and “sweet/candy” that can to be associated at linalyl acetate amount. Besides, the extraction microwave technology offers a similar odor of the plant. SW-HD sample gives a special typical “peppery spicy” & “citrus bergamot” interesting, that are characteristic notes of 4-terpineol and linalool [38], respectively. Besides, it is observed that the pre-treatment of the material by grinding (THD) or ultrasound (US-HD) before the hydro-distillation increases typicities “herbaceous” and “camphoraceous” while maintaining the “floral lavender” side. The pre-treatment with the salt (NaCl-HD) or enzyme (enzyme-HD) presented atypical off-notes. Besides, these extracts give lower intensity and persistence products. In conclusion, pre-treatment of the material with grinding (THD), ultrasound (US-HD), micelle-mediated (Micelle-HD) before hydro-distillation conventional and extraction by microwave technology (SFME and MSD) provides an excellent alternative to the traditional technologies such as hydro-distillation or steam distillation.
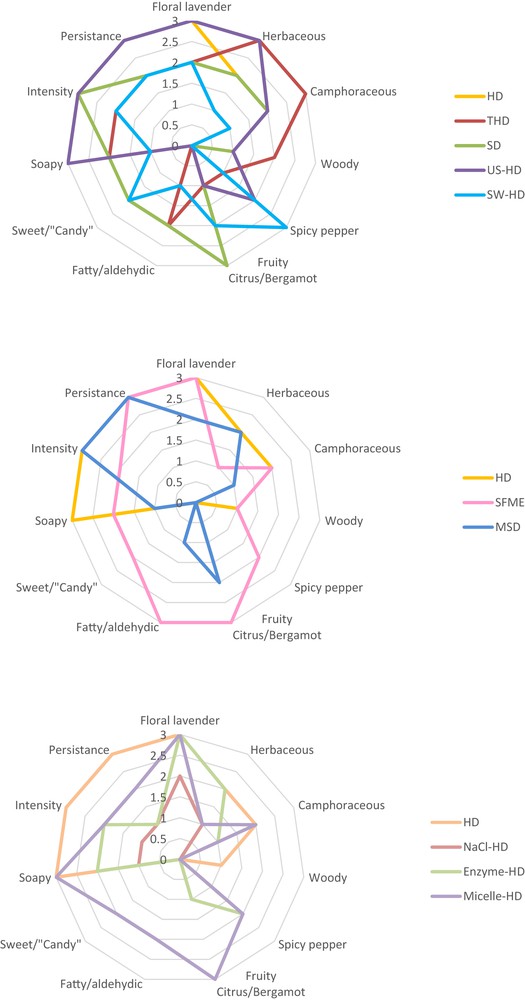
Sensorial analysis.
4 Conclusion
Using water as an alternative solvent for extraction includes: reduced environmental impact, selective extraction, use of simple equipment, no hazards, faster start-up, and simplification of process steps. Advantages and drawbacks of using water as a solvent with variable geometry have been compared to the hydro-distillation (Table 2).
Summarized advantages and drawbacks of using water as a solvent with variable geometry have been compared to the hydro-distillation.
The values extraction time, extraction yield, degradation, environmental impact of Hydro-distillation are considered as the reference. A better extraction yield, shorter extraction time compared to reference, gives positive impact (green). In contrast, longer extraction times, lower extraction efficiency, greater degradation of aromas or a significant environmental impact assessment will be considered as negative effects (red).
The results identified the optimal extraction technique as being the steam distillation assisted by microwave (MSD). Indeed, MSD offers important advantages over traditional hydro-distillation. It gave a yield of 5.4% in 30 min, versus 4.6% in 120 min for hydro-distillation and consumed 0.298 kWh against 3.452 kWh by the conventional method. The essential oil obtained was of excellent quality (linalyl acetate amount and a similar odor of the plant). MSD is a promising tool for the extraction of essential oil from lavender flowers.
Acknowledgments
Aurore Filly thanks “Région PACA” (Provence–Alpes– Côte d'Azur) and competitivity cluster PASS (Pôle Arômes Senteurs et Saveurs) for her doctoral grant.