1 Introduction
N-Heterocyclic compounds are the main building blocks in synthetic organic chemistry and they are often considered as privileged target molecules in pharmaceutical industries to develop some activity-specific lead molecules. As a result, the progress in the development of more efficient and versatile methods to synthesise N-heterocycles has always been interesting in organic synthesis. Especially, benzoxazole derivatives have attracted considerable attention because of their wide variety of applications in agrochemicals, natural products, functional materials and pharmaceutically active molecules [1]. The benzoxazole core has been found in a variety of cytotoxic natural products viz., natural antimycobacterial pseudopteroxazole [2], UK-1(bisbenzoxazole) [3], AJI9561 [4], and salvianen [5]. On the other hand, it has some medicinal chemistry applications consisting of the cathepsin S inhibitors [6], anticancer agents [7], 5-HT3 receptor agonists (Fig. 1) [8], non-nucleoside HIV reverse transcriptase inhibitors [9], oestrogen receptor-β agonists [10], selective peroxisome proliferator-activated receptor-γ antagonists [11], orexin-1 receptor antagonists [12], and elastase inhibitors [13]. In continuation, the benzoxazole core has some other applications such as herbicides, fenoxaprop and also used in fluorescent whitening agents such as bisbenzoxazolyl ethylenes and arenes (Fig. 1) [14]. In contrast, the core of interest has also exhibited remarkable applications in the fields of laser dyes [15], polymer production [16], organic light emitting diodes (OLEDs) [17] etc. Some of the benzoxazole derivatives, which are already booming inthe market, to quote a few, pseudopteroxazole (antimicrobial agent), UK-1(anticancer agent), AJI9561 (cytotoxic), benoxaprofen (non-steroidal anti-inflammatory drug, NSAID), flunoxaprofen (NSAID), tafamidis (hereditary amyloidosis), salvianen (cytotoxicity) fenoxaprop (herbicide) (Fig. 1).
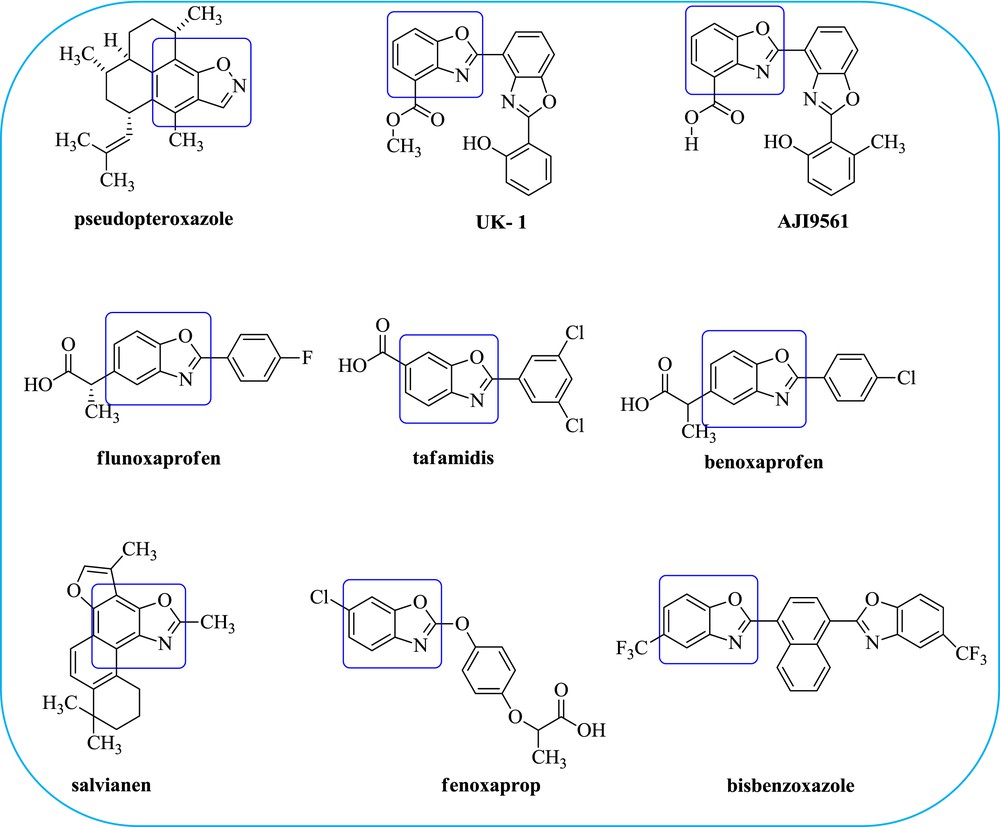
Structure of biologically and medicinally active benzoxazole derivatives.
Owing to such significance, the synthesis of benzoxazole and its derivatives has been an attractive goal for organic and medicinal chemists. From the literature, it is evident that several synthetic efforts have been made to prepare the benzoxazole scaffold. One of the traditional methods for the synthesis of benzoxazoles involves the construction of the O1C2 and N3C2 bonds via condensation of ortho-aminophenol with carboxylic acid or acyl chloride in the presence of a strong acid at elevated temperature [18], and the second widely followed method is through the aldehydes via oxidative cyclization of the phenolic Schiff bases with a wide variety of oxidizing agents viz., Mn(OAc)3 [19], DDQ [20], ThClO4 [21], NiO2 [22], ZrOCl2·8H2O [23], PCC-supported silica gel [24], Dess-Martin reagent [25], BaMnO4 [26], and Pb(OAc)4 [27]. On the other hand, a handful of approaches have been reported for the synthesis of benzoxazoles using diverse catalysts and other reagents viz., silica supported sodium hydrogen sulphate [28], Indion 190 resin [29], silica sulphuric acid [30], methane sulphonic acid [31], copper triflate [32], Indium triflate [33], SnCl2 [34], BF3·OEt2 [35], Shvo catalyst {[(η5-Ph4C4CO)]2HRu2(CO)4(μ-H)}, Co-salen complex, and 2,6-dimethoxybenzoquinone (DMBQ) as the terminal oxidant [36,37]. Yang et al., reported the synthesis of benzoxazole using mesoporous poly(melamine-formaldehyde) as a heterogeneous organocatalyst [38]. Lately, benzoxazole derivatives have also been prepared from 2-aminophenol and aldehydes with activated carbon [39], Pd/C in the presence of Hantzsch ester as a catalyst [40] and Al3+-exchanged K-10 clay as a heterogeneous catalyst [41].
Recently, the synthesis of 1,3-benzoxazoles using nanoparticles (NPs) was found to be an eye catching approach because of their large and reactive surfaces, high potential for selectivity, which may facilitate reaction under milder conditions, shorter reaction time, easier separation of the product and recyclability of the catalyst [42]. Several metal NPs as catalysts have been reported to synthesize the benzoxazole derivatives viz., CuO NPs supported on silica [43], ZnO NPs [44], nano CeO2 [45], KCN/MWCNTs (multi-walled carbon nanotubes) [46] and magnetic copper ferrite NPs [47]. Very recently, Maleki et al. reported the synthesis of benzoxazole derivatives using an Ag@TiO2 nano composite as a catalyst in aqueous media [48].
Most of the reported methods for the synthesis of benzoxazole suffer from the usage of harmful solvents, high reaction temperature, prolonged reaction times with low yields, tedious work-up procedures and the use of relatively expensive reagents. As a resultthere has been a continuous research interest on catalyst, there is search for much better catalysts to construct benzoxazoles with a simple work-up procedure, less toxicity, recovery and recyclability, environmental safety and atom economy. Many organic transformations have also been employed using metal mixed oxide and supported metal mixed oxide composites for the targeted synthesis. Especially, TiO2ZrO2 composite catalysts have been utilized in many organic reactions viz., dehydrogenation, isomerisation, epoxidation and dehydration [49]. Besides an array of new and innovative strategies has been developed that has made the synthesis of benzoxazole core a much simpler process than earlier. Therefore, herein we report a simple and efficient route for the synthesis of 2-aryl substituted benzoxazoles from 2-aminophenol and substituted aromatic aldehydes in the presence of TiO2ZrO2 composite metal oxide as a mesoporous heterogeneous catalyst (Scheme 1). Our model studies indicated that this reaction proceeds with a catalytic amount of mixed metal oxide. A short reaction time (15–25 min) and an easy work-up procedure are some of the advantages of this protocol.

Synthesis of benzoxazole derivatives using the TiO2ZrO2 catalyst.
2 Experimental section
2.1 Catalysts preparation
TiO2ZrO2 (1:1 mole ratio) mixed oxide was synthesized by a co-precipitation method from TiCl4 and ZrO(NO3)2·xH2O aqueous solutions by hydrolysis with ammonium hydroxide. In a typical experiment, the required quantities of ZrO(NO3)2·xH2O (SD Fine Chem. Ltd.) and TiCl4 (Loba Chemie) were dissolved separately in deionized water and mixed together. To this mixture, dilute aqueous ammonia (Emplura) was added dropwise with vigorous stirring until the precipitation was complete (pH = 9.5). The resultant precipitate was aged at room temperature under stirring for 12 h. The white precipitate thus formed was filtered and washed with deionized water until free from impurities. The obtained cake was oven dried at 120 °C for 12 h and finally calcined at 500 °C for 4 h in an air atmosphere. Similarly, individual metal oxides, TiO2 and ZrO2 were prepared for comparison.
2.2 General procedure for the synthesis of 2-aryl substituted benzoxazoles
In a 25 mL round-bottom flask equipped with a condenser, substituted aromatic aldehyde (1 mmol), 2-aminophenol (1 mmol) and TiO2ZrO2 (10 mol %) were taken and the mass was stirred in an oil bath at 60 °C for the appropriate time mentioned (Table 2). The progress of the reaction was monitored by TLC (eluent phase = n-hexane:EtOAc). After the completion of the reaction, the solvent was evaporated under vacuum and the reaction mixture was added to a beaker containing ethyl acetate to recover the catalyst by filtration and once again the solvent was removed under reduced pressure. The crude product was then subjected to column chromatography to give the pure product. The melting points and spectral data of the known compounds are found to be identical to the values reported in the literature.
Temperature optimization for the synthesis of 2-phenylbenzo[d]oxazole (3a).
Entry | Temperature (°C) | Timea (min) | Yieldb (%) |
1 | RT | 180 | 30 |
2 | 40 | 45 | 75 |
3 | 50 | 45 | 82 |
4 | 60 | 20 | 90 |
5 | 70 | 20 | 90 |
6 | 80 | 20 | 88 |
a Reaction time is monitored by TLC.
b Reaction condition: 2-Aminophenol (1 mmol), benzaldehyde (1 mmol), and catalyst (10 mol %) in acetonitrile at different temperatures.
2.3 Characterization techniques
The melting points of the products were determined by open capillaries and are uncorrected. The 1H and 13C NMR spectra were recorded on Bruker AVANCE III spectrometers at 300 and 400 MHz, respectively. Chemical shifts (δ) are reported in ppm from the standard internal reference tetramethylsilane (TMS). The following abbreviations are used: s = singlet, d = doublet, t = triplet, m = multiplet in the spectral interpretation. Mass spectra were recorded on a Micromass Quattro II using Electrospray Ionization (ESI) technique, showing the (M+H) peak as a prominent base peak. The homogeneity of the compounds was described by TLC on aluminium silica gel 60 F254 (Merck) detected by UV light (254 nm) and iodine vapours. The powder X-ray diffraction patterns of the catalysts were recorded on a Ultima-IV X-ray diffractometer (M/s. Rigaku Corporation, Japan) using Ni-filtered Cu Kα radiation (l = 1.5406 Å) with a 2θ scan speed of 2°/min and a 2θ scan range of 10–80° at 40 kV and 30 mA. Crystalline phases were identified by comparison with the reference data from International Center for Diffraction Data (ICDD) files. The crystallite size was calculated using Debye–Scherrer equation (D = Kλ/β Cosθ).
The BET Surface area and pore size distribution studies were conducted with N2 at liquid N2 temperature (−196 °C) on a Quadrasorb-SI (M/s. Quantachrome Instruments, USA) for getting the multipoint surface area using the Brunauer–Emmett–Teller (BET) analysis method and total pore volume by the Barrett–Joyner–Halenda (BJH) method. Prior to the measurement, the sample was pre-heated at 100 °C for 2 h in order to expel the interlayer moisture under vacuum control. The scanning electron microscopy (SEM) measurements were made using a Carl Zeiss Ultra 55 FESEM system.
3 Results & discussion
3.1 Characterization studies
Table 1 depicts the BET surface area to verify the textural characteristics of all the metal oxides. As can be noted from the table, the TiO2ZrO2 mixed oxide exhibits a high specific surface area of 171 m2 g−1. The surface area of the mixed metal oxides is remarkably higher than their individual component oxides, TiO2 (59 m2 g−1) and ZrO2 (26 m2 g−1). The increase in the surface area of the mixed oxide can be due to the strong interaction between the TiO2 and ZrO2 as explained by Reddy et al. [49]. Further, it is noteworthy that all the adsorption–desorption isotherms follow type IV BET isotherm which is characteristic of mesoporous materials as depicted in Fig. 2. The steep curves at relative pressure 0.5 for mixed oxide, 0.77 for TiO2, and 0.73 for ZrO2 can be attributed to the capillary condensation in pores [50]. During co-precipitation of TiO2ZrO2 the interaction between these oxides increases, which subsides their individual crystallization. Further, the BJH pore size distribution also supported the mesoporous nature of the catalysts [51]. In the mixed oxide catalyst TiO2ZrO2, the pore volume increased with the increase in pore diameter as depicted in Table 1. Thus, the strong interaction between TiO2 and ZrO2, the high surface area and increase in pore volume may contribute to the increase in the activity of TiO2ZrO2 catalysts.
BET surface area and X-ray diffraction data of TiO2, ZrO2 and TiO2ZrO2 mixed oxides.
Catalyst | BET surface area (m2 g−1) | Pore diameter (nm) | Pore volume (cm3 g−1) | Crystallite size (nm) | XRD phases |
TiO2 | 59 | 3.92 | 0.081 | 49.86 | Anatase and rutile |
ZrO2 | 26 | 3.92 | 0.035 | 116.84 | Monoclinic |
TiO2ZrO2 | 171 | 4.42 | 0.2385 | Amorphous | Monoclinic |

BET surface area and pore size distribution of TiO2ZrO2, ZrO2, and TiO2.
The XRD patterns of pure TiO2, ZrO2 and TiO2ZrO2 mixed oxide are depicted in Fig. 3. The characteristic peaks of crystal phases for ZrO2 are observed at 2θ = 28.02, 31.54, 37.31, 50.05 and 63.93° with monoclinic having tetragonal phases (JCPDS No. 83-936). On the other hand the characteristic peaks of TiO2 were observed at 2θ = 25.38, 37.70, 43.87, 48.12, 53.9, 62.39, 69.14 and 74.93° consisting of anatase and rutile phases (JCPDS No. 84-1286) whereas TiO2ZrO2 displayed only an amorphous peak with the presence of a zirconyl titanate phase (JCPDS No. 8-352). In particular, independent diffraction lines due to the crystalline TiO2 and ZrO2 phases were not observed. This change of phase from crystalline to amorphous has been reported in several studies during the stages of TiO2ZrO2 mixed oxide preparation [52]. The crystallinity of zirconyl titanate (ZrTiO4) as observed from the XRD patterns may influence the activity of the catalyst.

XRD patterns of (a) TiO2, (b) ZrO2 and (c) TiO2ZrO2.
The particle size morphology of the catalysts was examined by SEM and these images represent the non-uniformly distributed irregular shaped morphology of the TiO2ZrO2 sample with particle size averaging between 2 and 5 μm (Fig. 4b).

The scanning electron micrograph of the TiO2ZrO2 catalyst.
3.2 Activity studies
In our initial studies, the reaction of 2-aminophenol (1) and benzaldehyde (2a) was chosen as a model for finding the optimization conditions (the effect of temperature, solvent and catalyst concentration) to achieve good yield of the desired products. In order to investigate the effect of temperature on the reaction, the concentration of the catalyst was kept constant at 10 mol % and a mixture of substrates was treated with toluene as a solvent. The reaction was monitored by TLC at different temperatures ranging from 40 to 80 °C, with an increment of 10 °C each time. Our results indicate that, after stirring the reaction mixture at room temperature for 180 min, the yield of the corresponding product (3a) was low (Table 2, entry 1), Subsequently, the mixture was heated in an oil bath and increasing the reaction temperature from 40 to 60 °C (Table 2, entry 2–4), resulted in better yields and reaction time. Further increase in the temperature (>60 °C) did not show any significant improvement in the yield and the reaction time (Table 2, entry 5–6). Therefore, 60 °C was selected as the reaction temperature for all subsequent reactions.
In order to establish the real effectiveness of the catalyst for the synthesis of 2-phenylbenzo[d]oxazole (3a) a test reaction was performed without the catalyst at room temperature in air. It was found that only a trace amount of product was obtained in the absence of catalyst even after 720 min (Table 3, entry 1). Then the reaction is carried out in the presence of the catalyst with different concentrations (5, 10 and 20 mol %) and optimized as 10 mol %, where high yields of the product, 90%, with shorter time (Table 3, entries 4) were achieved without any byproducts. Upon further increase in the concentration of the catalyst, the rise in the yields of the product remains unaffected (Table 3, entries 5).
Effect of catalyst concentration in the synthesis of 2-phenylbenzo[d]oxazole (3a)a.
Entry | Catalyst concentration (mol %) | Time (min) | Yield (%) |
1 | – | 720 | 40 |
2 | – | 420 | Traceb |
3 | 5 | 60 | 75 |
4 | 10 | 20 | 90 |
5 | 20 | 20 | 90 |
a Reaction condition (entry 3–5): 2-Aminophenol (1 mmol) and benzaldehyde (1 mmol), at 60 °C by varying the amount of catalyst in toluene.
b Reaction at room temperature.
After optimizing the temperature and amount of catalyst, the reaction is carried out in different solvents like toluene, acetonitrile, ethanol, THF, and dichloromethane. It is observed that the reaction proceeds in different solvents with varied intervals of time along with yield (Table 4) and it is found that acetonitrile (Table 4, entry 2) is the best solvent for this transformation using TiO2ZrO2 as a catalyst within 15 min at 60 °C.
Effect of solvent in the synthesis of 2-phenylbenzo[d]oxazole (3a)a.
Entry | Solvent | Time (min) | Yieldb (%) |
1 | Toluene | 20 | 90 |
2 | Acetonitrile | 15 | 93 |
3 | Ethanol | 30 | 75 |
4 | Tetrahydrofuran | 120 | 87 |
5 | Dichloromethane | 90 | 69 |
a Reaction condition: 2-Aminophenol (1 mmol), benzaldehyde (1 mmol), and solvent (5 mL) at 60 °C using 10 mol % of catalyst.
b Isolated yields.
Once the effective temperature, catalytic amount and solvent optimization were proven thereafter, to show the generality of this method, a series of various aromatic aldehydes were treated with 2-aminophenol under optimized reaction conditions and the results are summarized in Table 5. The aromatic aldehydes with substituents at ortho, meta or para-positions show equal ease towards the product formation in high yields. In contrast, aromatic aldehydes having groups like Cl, F, Br and OMe showed better reactivity and the reactions were completed in shorter time (Table 5, entry 8, 9, 12 and 14) and showed excellent yields. Hetero-aromatic aldehydes like furfuraldehyde and thiophenaldehyde afforded the desired product in quantitative yields (Table 5, entry 15 and 16). We eventually achieved excellent yields in a very short duration under milder reaction conditions. We have also investigated the effect of substituents on 2-aminophenol under the same optimized reaction conditions and it is found that the reaction of substituted 3-fluoro-2-aminophenol with benzaldehyde showed good yield of 3q (Table 5, entry 17). It is observed that the catalyst has excellent tolerance to functionalized groups on 2-aminophenol. All the synthesized products are known compounds and were characterized by comparing their melting points, 1H, 13C NMR and MS.
Synthesis of 2-aryl benzoxazole derivatives using TiO2ZrO2 as a catalysta.
Sl. No. | Aldehyde | Entry | R | Productb | Reaction Time (min)c | Yield (%)d |
1 | 3a | H | 15 | 91 | ||
2 | 3b | H | 20 | 93 | ||
3 | 3c | H | 15 | 92 | ||
4 | 3d | H | 15 | 86 | ||
5 | 3e | H | 25 | 85 | ||
6 | 3f | H | 20 | 87 | ||
7 | 3g | H | 20 | 89 | ||
8 | 3h | H | 15 | 92 | ||
9 | 3i | H | 15 | 90 | ||
10 | 3j | H | 20 | 88 | ||
11 | 3k | H | 15 | 90 | ||
12 | 3l | H | 20 | 93 | ||
13 | 3m | H | 25 | 89 | ||
14 | 3p | H | 15 | 93 | ||
15 | 3n | H | 20 | 89 | ||
16 | 3o | H | 20 | 91 | ||
17 | 3q | 6-Me | 40 | 83 |
a Reaction condition: 2-Aminophenol (1 mmol), benzaldehyde (1 mmol), and TiO2ZrO2 (10mol %) at 60 °C in acetonitrile.
b All products were characterized by comparison of their mp, NMR, MS and elemental analysis with those of authentic samples.
c Reaction is monitored by TLC.
d Isolated yield.
Further, in order to show the excellent catalytic activity of TiO2ZrO2 and the efficiency of this procedure, we have compared some of the results obtained by our method with those of the reported methods (different supported metal Lewis acid catalysts and metal oxides such as Sm(OTf)3, Zn(OTf)3, TiO2 and ZrO2) in the literature, as shown in Table 6. From the above table, it is evident that the best reaction condition was obtained using TiO2ZrO2, as a catalyst for the benzoxazole synthesis (Table 6, entry 3). These results reveal that the present protocol could serve as a better method than the existing/reported methods. Therefore, the present methodology is superior to the reported methods in terms of yield and reaction time.
Comparison of the efficiency of catalysts for synthesis of 2-phenylbenzo[d]oxazole (3a)a.
Entry | Catalysts (10 mol %) | Reaction time (min) | Yieldb (%) |
1 | TiO2 | 720 | 48 |
2 | ZrO2 | 600 | 71 |
3 | TiO2ZrO2 | 15 | 93 |
4 | Sm(OTf)3 | 180 | 88 |
5 | Zn(OTf)3 | 300 | 89 |
a Reaction condition: 2-Aminophenol (1 mmol), aldehydes (1 mmol) and the corresponding catalysts (10 mol %) at 60 °C in acetonitrile.
b Isolated yields.
The reusability of the catalyst is an important factor from economic and environmental points of view and has attracted much attention in recent years. Therefore, we have also attempted to study the reusability of the TiO2ZrO2 catalyst for at least three more cycles. After the fresh run, 93% yield was obtained and then the catalyst was recovered by filtration from the reaction mixture. The recovered catalyst was dried again under vacuum for 10 h and tested in up to three more reaction cycle runs. We found that the recovery and reuse of the catalyst showed minimal decrease in yields without loss of catalytic activity. The product 3a was obtained in 93, 87, 81 and 76% yields after successive cycles (Fig. 5).

Reusability of the catalyst for the synthesis of 2-phenylbenzo[d]oxazole (3a).
It is found in the literature that mixing of two dissimilar oxides can lead to totally different physicochemical properties and catalytic behaviour due to the formation of a stable compound. TiO2 acts as the Lewis base and ZrO2 acts as the Lewis acid. Herein, the active ZrTiO4 phase of the mixed oxides not only takes advantage of carrying both acidic and basic sites but also extends their application through the generation of new catalytic sites. In the TiO2ZrO2 mixed oxides, the interaction of the individual oxides can be associated with the TiOZr linkages [49].
A proposed catalytic cycle for the synthesis of benzoxazoles (3a) is described in Scheme 2. The plausible mechanism of TiO2ZrO2 catalysed condensation of 2-aminophenol with aldehydes for the synthesis of 2-aryl benzoxazoles is based on the literature [53]. Initially, the amine add to the carbonyl group of the aldehyde to form amide (A) and then the TiO2ZrO2 catalyst would most likely coordinate with the oxygen group of the amide bond to polarize the carbonyl group to form the oxonium intermediate (B). Then subsequent attack of lone pair of electrons of the hydroxyl group of aminophenol on the carbonyl group of amide takes place to get the intermediate C via intramolecular cyclization followed by removal of the water molecule to get 2-aryl substituted benzoxazole and the regenerated catalyst.

Proposed plausible mechanism for synthesis of 2-aryl benzoxazole using TiO2ZrO2 catalyst.
4 Conclusions
In conclusion, we have developed a mild, easy, and efficient method for the synthesis of 2-aryl substituted benzoxazole using the TiO2ZrO2 mixed oxide composite as a green and speedy catalyst. The activity of the catalyst directly depends on the physicochemical properties of the ZrTiO4 and its surface acid-base bi-functionalities. The remarkable advantages of this method such as the efficiency, high yields with short reaction times, simple reaction conditions, clean product profile, ease of preparation of the catalyst, inexpensive and environmentally friendly catalyst, ease of product isolation and cost efficiency made this approach an interesting alternative to the existing methods. All of these make it a useful and attractive strategy for the preparation of various benzoxazole derivatives simply by changing different substrates. We believe that this new catalytic protocol may be useful for the synthesis of the highly complex benzoxazole molecule for future medicinal chemistry research. The scope, mechanism (labelling study), and synthetic applications to natural products are under investigation.
Acknowledgements
The authors thank Prof. Geetha R. Balakrishna, Director, CNMS, Jain University, Bangalore, Karnataka, India. The authors also thank the DST and Nano mission (SR/NM/NS-20/2014).