1 Introduction
Porous zeolite-like aluminophosphate (AlPO4-n) molecular sieves have attracted much interest since the first synthesis by Wilson in 1982 [1] because of their catalytic and effective sportive properties (chemical affinity of form and size, porosity and high sorption capacity). These molecular sieves constitute a material with selective sorption properties based on molecular size and shape difference. AlPO4-n contain several structures including zeolite topological analogues and a large number of novel structures [2]. Isomorphous substitution of their Si (IV) by P (V) leads to the synthesis of new materials abbreviated as SAPO-n and create a negative charge in the framework, which considerably influences their mechanism and capacity sorption [3]. The AFI structure consists of one-dimensional 12-membered ring pores (7.3 × 7.3 Å in size) and 10-membered ring pores (6.5 × 4.0 Å) for AEL structure [4]. These channels are larger than the diameter of the hydrated uranyl (6.5 Å) allowing its sorption by AFI and AEL materials [5]. The trapping of uranium within the structural gaps of AFI and AEL materials reduced volumes of radioactive effluents and convert it to a stable solid waste [6].
Uranium is a long-lived radioelement present in various radioactive liquid wastes. It is hazardous because of both its chemical toxicity and radioactivity [7]. Several techniques have been used for effluent decontamination. These include chemical precipitation, membrane-based separation, solvent extraction, ion exchange and sorption [8,9]. Among these techniques, sorption is often preferred because of its low cost and high removal efficiency [10]. The sorption of uranium onto various solids is a current process used in the purification of environmental and radioactive waste disposal [11]. Indeed, a large number of studies have focused on the use of natural and synthetic zeolites [12–17]. Inorganic sorbents such as zeolites are considered to be backfill materials in the safe processing of nuclear wastes because of their ability in retarding the release of radionuclides in the environment [18]. The aim of the present study is to highlight the effectiveness of porous zeolite-like aluminophosphate and silicoaluminophosphate with AFI and AEL structures for radioactive waste management.
2 Experimental section
2.1 Chemicals and reagents
All of the reagents used for the experiments are of analytical grade.
The synthetic stock uranium solution of 1 g/L at pH 3.5 was prepared by dissolving the appropriate amount of uranyl nitrate hexahydrated salt, UO2(NO3)2·6H2O (99%, Merck), in distilled water with 1 mL of nitric acid. Experimental uranium solutions of concentrations 50, 100 and 150 mg/L were obtained by dilution with distilled water.
2.2 Synthesis and characterization of AlPO4-5, SAPO-5, AlPO4-11 and SAPO-11 sorbents
AlPO4-5, SAPO-5, AlPO4-11 and SAPO-11 sorbents are synthesized hydrothermally according to the literature [2,19]. The gel composition for AlPO4-n and SAPO-n sorbents is Al2O3, 1 P2O5, and 1.4 R, 50 H2O and 0.8 Al2O3, 1 P2O5, 1.4 R, 0.2 SiO2, 50 H2O, respectively. The direct-structuring agents (R) used for AFI and AEL structures are tripropylamine and dipropylamine (99%, Fluka), respectively. The aluminium, silicon and phosphorous sources are pseudoboehmite catapal B (69%, Vista), aerosil SiO2 (100%, Deggusa) and phosphoric acid (85%, Merck), respectively. The gels are introduced into 120 mL Teflon lined stainless steel autoclaves and heated at 473 K under autogenic pressure for 24 and 48 h for AFI and AEL sorbents, respectively. After the heat treatment, the autoclave is cooled in water. The resulting solids are separated by filtration, washed with distilled water and dried at 353 K overnight. The synthesized materials are calcined at 823 K for 6 h to remove the organic template.
The structural analysis is performed using X-ray diffractometer, Philips X'PERT SW powder diffractometer, with Cu Kα1 radiation (k = 1.54060 Å).
The nitrogen adsorption–desorption measurements are made using a Quantachrome Nova 3200e surface analyser at 77 K. The surface areas of synthesized sorbents are obtained by the Brunauer–Emmett–Teller (BET) method. The chemical compositions are investigated by X-ray fluorescence spectrometer type MAGIX Pro. The calculated charge by TO2 of a silicoaluminophosphate material with a composition form, [Si4+]x[Al3+]y[P5+]zO2, is equal to 4x + 3y + 5z + (−2) × 2, where x, y, z, 2 are the molar fractions of Si, Al, P and O and 4, 3, 5 and −2 are the charge of Si, Al, P and O, respectively.
The elements' composition of the effluents is investigated by a GBC Avanta Sigma-type atomic absorption spectrophotometer.
2.3 Sorption experiments
The sorption of uranium (VI) onto synthesized AlPO4-5, SAPO-5, AlPO4-11 and SAPO-11 is studied using the batch technique. The batch sorption is performed by agitating in a thermostat water bath shaker a mass m (g) of the sorbent in polyethylene flasks containing a volume V (mL) of solution with different concentrations of uranium (50, 100, and 150 mg/L). The shaking speed is maintained at 200 rpm throughout the study. At the end, the sorbent is centrifuged for 5 min at 3500 rpm. The residual concentration of the ions left in the supernatant phase is determined using a UV-spectrometer following the Arsenazo III procedure [5,20]. The solution pH is adjusted to the required value ranging from 2 to 11 using a solution of 0.1 M HCl (37%) or 0.1 M NaOH (98%).
The sorption uptake and the equilibrium metal uptake capacity qe (mg/g) are, respectively, calculated from the following expressions:
(1) |
(2) |
To optimize uranyl ion removal conditions, experiments are first performed using the synthetic solution. The obtained optimized parameters (pHAEL = 7, pHAFI = 6, contact time = 120 min and solid-to-liquid ratio of 0.1/150 (g/mL)) are then used as experimental conditions in the uranium sorption tests using nuclear effluents.
3 Results and discussion
3.1 Characterization of AEL and AFI adsorbents
The X-ray diffraction (XRD) patterns of the prepared AEL and AFI sieves are shown in Fig. 1. The obtained diffractograms indicate that the hydrothermally synthesized AlPO4-5 and SAPO-5 are pure phase AFI and AlPO4-11 and SAPO-11 are pure phase AEL with high crystallinity. The X-ray diffractograms are identical to those presented in the literature characterizing AFI and AEL crystalline phases [21,22].
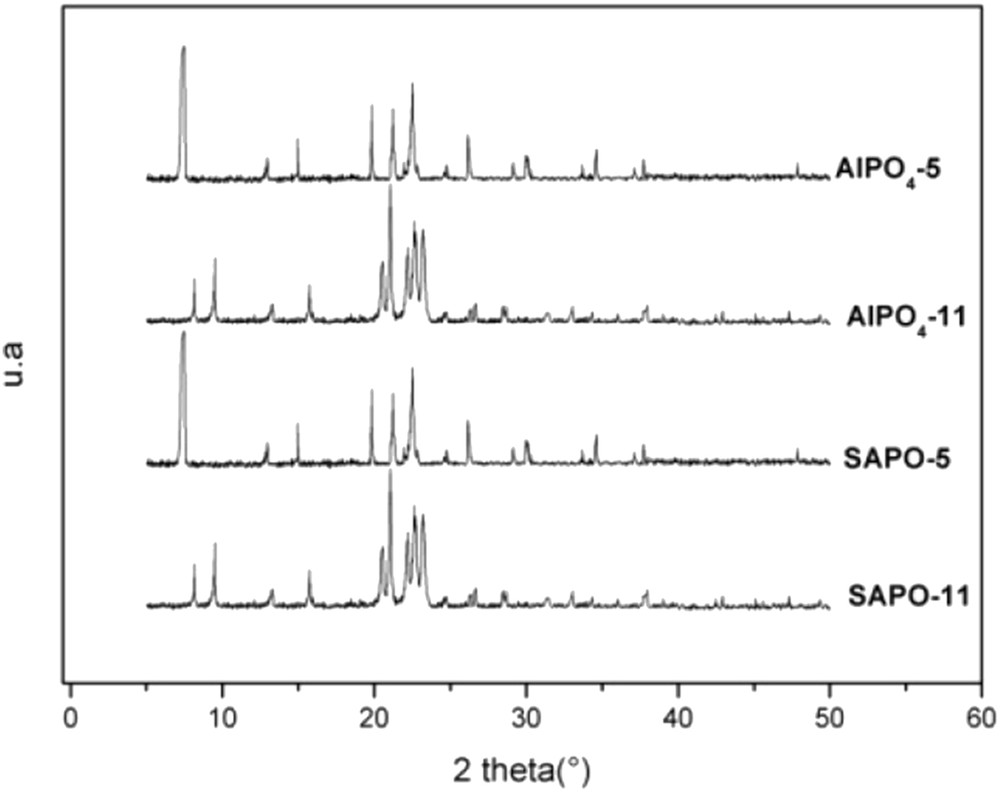
X-ray diffraction patterns of calcined AlPO4-5, SAPO-5, AlPO4-11 and SAPO-11 sorbents.
The chemical composition obtained by the X-ray fluorescence analysis and the calculated charge of AFI and AEL sorbents are given in Table 1.
Textural characteristics and chemical compositions of AEL and AFI adsorbents.
Sorbents | SBET (m2/g) | V (cm3/g) | Chemical composition | Charge/TO2 |
SAPO-5 | 230 ± 1 | 0.371 ± 0.001 | Si0.075 Al0.489 P0.436 O2 | −0.055 |
AlPO4-5 | 220 ± 1 | 0.262 ± 0.001 | Al0.502 P0.498 O2 | 0 |
SAPO-11 | 153 ± 1 | 0.238 ± 0.001 | Si0.063 Al0.489 P0.448 O2 | −0.041 |
AlPO4-11 | 148 ± 1 | 0.212 ± 0.001 | Al0.499 P0.501 O2 | 0 |
The surface area calculated according to the BET model and the pore volumes for the samples prepared are summarized in Table 1. One can notice that the AFI porous volume and surface area values are larger than the corresponding values for AEL sorbents.
3.2 Application of AEL and AFI sorbents in nuclear effluents
The uranium sorption tests of the synthesized sorbents are performed using nuclear radioactive effluents collected from the Nuclear Research Centre of Draria (Algeria). The effluents are taken at different unit operations along the process of uranium purification and concentration in the uranium ore treatment. Three samples are collected and placed separately in polyethylene bottles of 450 cm3 volume each. The bottles are completely sealed for more than 30 days to allow radioactive equilibrium to be reached. The measurement of uranium-235 activity is carried out using a detector hyper-pure germanium of 30% efficiency. The detector has a resolution of 1.9 keV at the 60Co gamma-ray energy of 1332 keV. The gamma-ray spectrometer energy and efficiency calibration is performed using a 152Eu source in a Marinelli beaker (multi-gamma volume source in a water-equivalent resin matrix). The samples are packaged in Marinelli-type containers, and the acquisition of gamma spectra duration is 86,400 s. The spectrum deconvolution software used is gamma vision of ORTEC, an example of gamma spectrum is given in Fig. 2. The elements and radioelement compositions of the three studied effluents are given in Table 2.
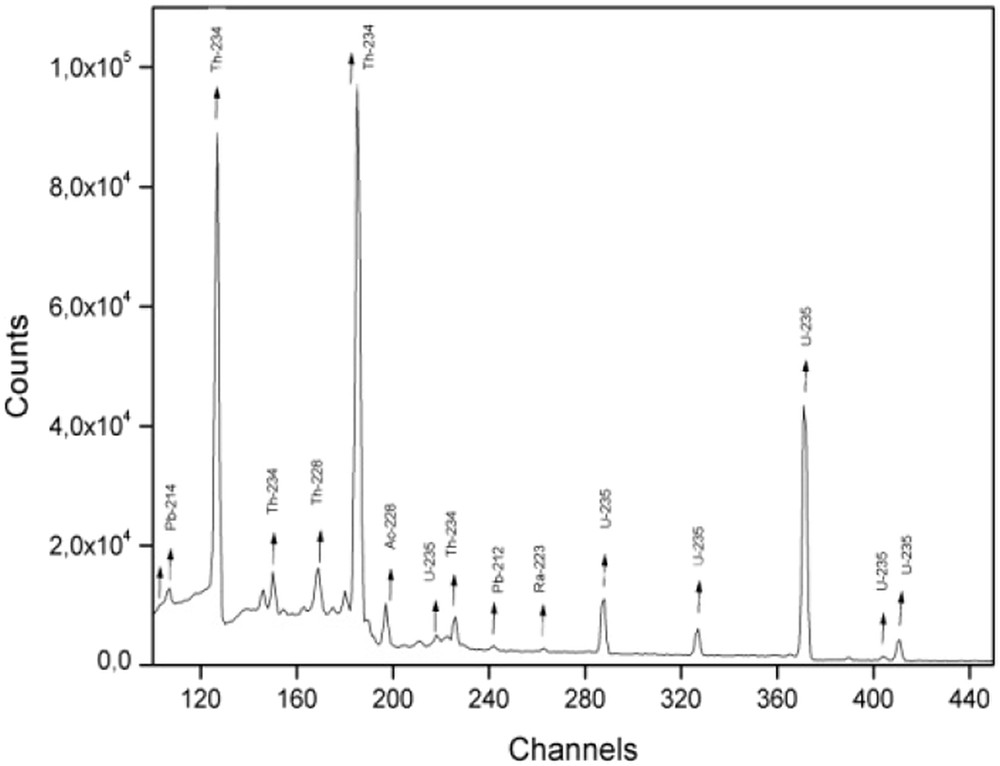
Portion of the gamma-ray spectrum of effluent 2.
The elements and radioelement compositions of the effluents.
Effluents | Nuclide | Average activity (Bq/L) | Energy (keV) | Element | Concentration (mg/L) |
1 | U-235 | 79.0 | 185.72 | U | 150 |
Pb-214 | 05.8 | 351.99 | Fe | 63 | |
Ac-228 | 05.6 | 338.40 | Mg | 9.52 | |
Th-234 | 905.1 | 112.81 | Cu | – | |
Pb-210 | 23.1 | 46.52 | Zn | – | |
Pb-212 | 02.9 | 238.63 | Mn | 0.7 | |
2 | U-235 | 49.1 | 185.72 | U | 100 |
Pb-214 | 03.5 | 295.22 | Fe | 62.5 | |
Ac-228 | 03.2 | 462.73 | Mg | 1.12 | |
Th-234 | 231.1 | 112.81 | Cu | Traces | |
Ra-223 | 01.3 | 122.40 | Zn | Traces | |
Pb-212 | 09.5 | 115.18 | Mn | – | |
3 | U-235 | 26.5 | 185.72 | U | 50 |
Pb-214 | 01.5 | 351.99 | Fe | 41.14 | |
Ac-228 | 01.3 | 968.90 | Mg | 28.60 | |
Th-234 | 346.2 | 92.80 | Cu | 3.11 | |
Pb-210 | 02.0 | 46.52 | Zn | 12.83 | |
Pb-212 | 24.1 | 115.18 | Mn | – |
To test the effluent composition influence on uranium sorption, experiments were performed using real effluents and synthetic uranium solutions at 50, 100 and 150 mg/L under experimental conditions of pHAEL = 7, pHAFI = 6, contact time = 120 min and solid-to-liquid ratio of 0.1/150 (g/L). The percentages of uranium (VI) ions' sorption from nuclear effluents and synthetic solutions onto SAPO-5, AlPO4-5, SAPO-11 and AlPO4-11 sorbents are presented in Fig. 3.
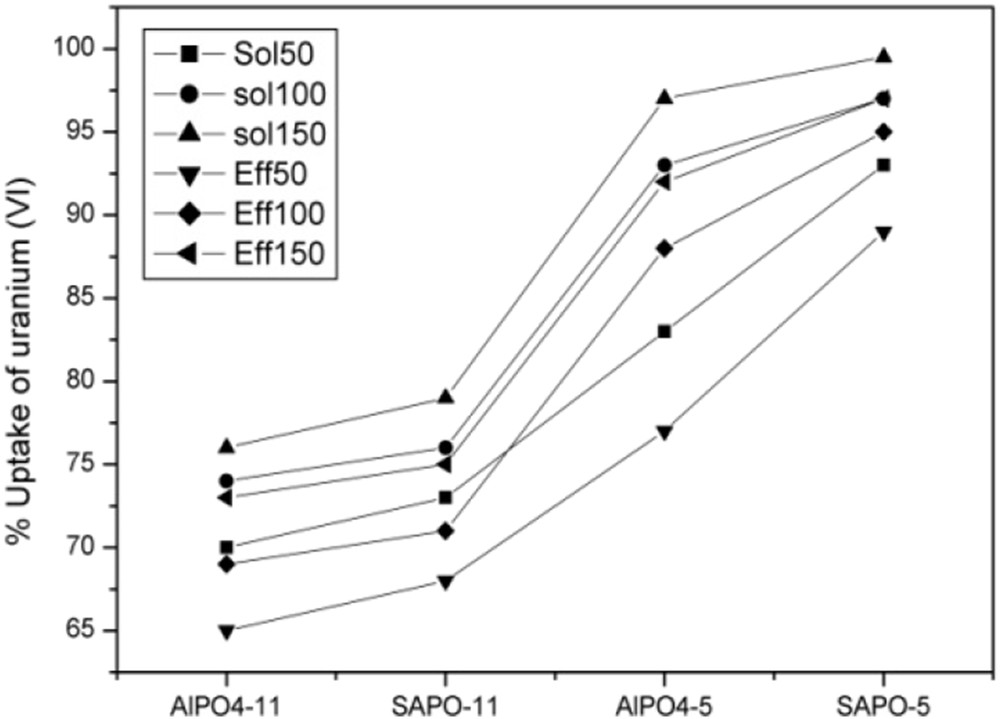
The uranium uptake percentage from synthetic solution and real effluents sorbed onto AlPO4-5, SAPO-5, AlPO4-11 and SAPO-11.
According to the obtained results presented in Fig. 3, the sorption rate of uranium (VI) follows the order: % SAPO-5 > % AlPO4-5 > % SAPO-11 > % AlPO4-11. The same trend is observed for both real effluents and synthetic solutions. It appears that AFI sorbents present the larger values of uranium uptake for all the solutions used in this study. This behaviour may be explained by the difference in the structure where SAPO-5 and AlPO4-5 are part of a 12-membered ring pore structure, which permits uranium to diffuse out the pore at a relatively faster rate than onto SAPO-11 and AlPO4-11, which belongs to a 10-membered ring pore structure [2]. Furthermore, the higher surface area and pore volume (Table 1) are responsible for the good sorption properties of the adsorbent with AFI structure. We can also notice, for the sorbents belonging to the same structure, the SAPO sorbent is more efficient than the AlPO; this behaviour may be explained by the silicoaluminophosphate framework negative charge, which could favour the sorption of positively charged uranyl ion species in contrast to the neutral framework of aluminophosphate [3]. As can be seen from Fig. 3, uranium (VI) sorption uptake increases with initial uranium concentration for all sorbents used. This can be because of the increase in mass driving force from solution to the sorbent surface, which enhances the interaction between sorbate and sorbent [12,22]. For the same concentration used, the percentage of uranium (VI) ions is larger in the synthetic solution than in real effluent. This is mainly because of the presence of other elements in the effluents (Table 2), which can be cosorbed with uranium and compete with it to occupy the active sites for the sorbents [23]. Thus, the coexistence of various elements such as thorium, iron and manganese may compete for the sorption sites with uranium ions resulting in a substantial reduction of uranium removal. Indeed, it has been demonstrated that the inhibition of uranium sorption is because of the presence of thorium and iron, which are considered as the most potent competitors of uranium for sorptive sites [24,25].
3.3 Decontamination factor
Decontamination factor (DF) is a dimensionless quantity used to describe the ratio of the contamination level before treatment to that after treatment. It is very useful for the selection of suitable materials as sorbent and commonly used in waste management applications [26].
The DF is calculated using the following equation:
(3) |
As can be seen from Table 3, DF values are more important for AFI sorbents, indicating a significant decontamination effect of both sorbents. This value increases with effluent activity and temperature. Moreover, SAPO-5 exhibits a higher DF value, showing that it is more suitable for uranium (VI) removal from nuclear effluents than AEL sorbents.
Effluent decontamination factors of AEL and AFI sorbents.
Effluents | U-235 activity (Bq/L) | Decontamination factor (DF) | |||||||
SAPO-5 | AlPO4-5 | SAPO-11 | AlPO4-11 | ||||||
293 K | 323 K | 293 K | 323 K | 293 K | 323 K | 293 K | 323 K | ||
1 | 26.5 ± 0.2 | 44.84 | 1000 | 5.36 | 100 | 3.60 | 17 | 3.33 | 14.28 |
2 | 47.1 ± 0.2 | 49.50 | 1667 | 11.86 | 200 | 3.89 | 25 | 3.54 | 20.00 |
3 | 79.0 ± 0.2 | 53.00 | 5000 | 24.78 | 500 | 4.70 | 50 | 3.75 | 33.33 |
3.4 Thermodynamic study
The thermodynamic parameters, that is, enthalpy ΔH°, entropy ΔS° and free energy ΔG° for the sorption of uranium (VI) ions onto AFI and AEL are calculated from the slope and the intercepts of ln Kd versus 1/T linear regression plots Fig. 4. The estimated values of the thermodynamic parameters for the sorption of uranium (VI) onto AFI and AEL sorbents are summarized in Table 4.
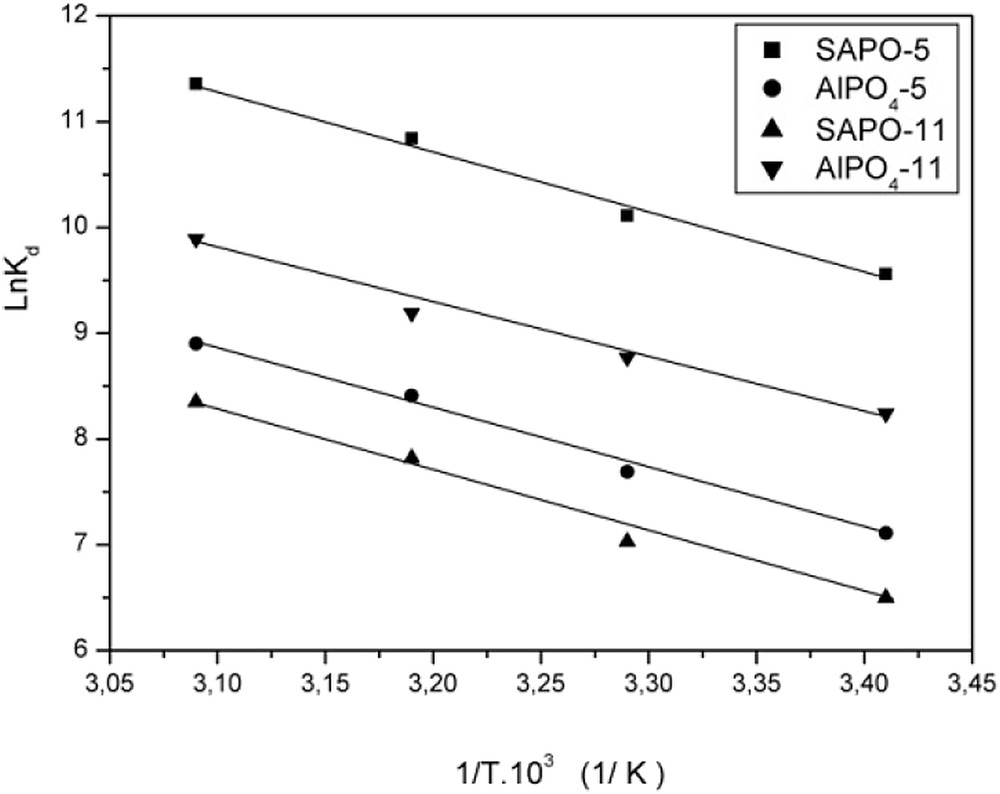
The thermodynamic parameters for the sorption of uranium (VI) onto AlPO4-5, SAPO-5, AlPO4-11 and SAPO-11 sorbents.
Thermodynamic parameters for uranium (VI) sorption onto AEL and AFI sorbents.
Sorbents | ΔH° (kJ/mol) | ΔS° (J/mol K) | ΔG° (kJ/mol) | |||
293 K | 303 K | 313 K | 323 K | |||
SAPO-5 | 47.88 | 242.51 | −23.21 | −25.63 | −28.06 | −30.48 |
AlPO4-5 | 41.95 | 211.16 | −19.95 | −22.06 | −24.17 | −26.28 |
SAPO-11 | 47.63 | 221.40 | −17.27 | −19.48 | −21.70 | −23.91 |
AlPO4-11 | 48.05 | 217.82 | −15.80 | −17.98 | −20.16 | −22.33 |
The observed positive values for the enthalpy ΔH° indicate the endothermic nature of the process. Moreover, the positive value for entropy ΔS° reflects the affinity of AFI and AEL sorbents to uranium (VI) removal. The negative ΔG° values observed for all sorbents show that the sorption process of uranium (VI) is spontaneous and the degree of spontaneity increases with increasing temperature.
3.5 Sorption diffusion
In the objective to determine the sorption mechanism, the kinetic results obtained under experimental conditions (pHAEL = 7, pHAFI = 6, solid-to-liquid ratio of 0.1/150 g/L, [U] = 50 mg/L and time ranging from 5 to 240 min) are analysed by the intraparticle diffusion model expressed as
(4) |
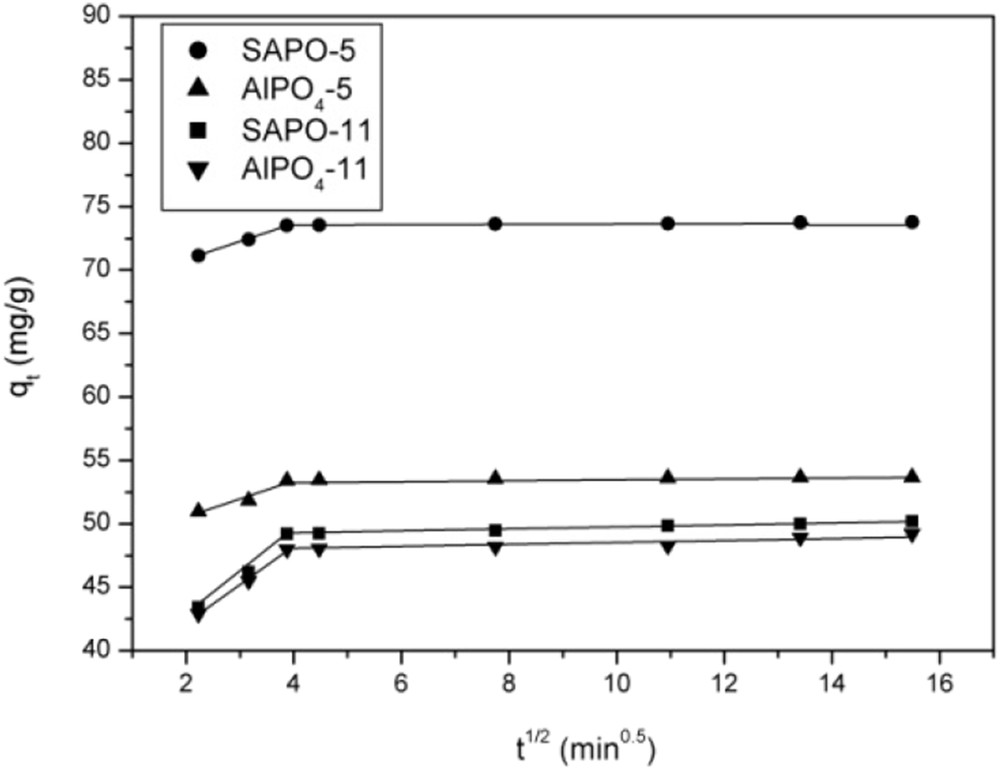
Intraparticle diffusion plots for uranium (VI) sorption onto AlPO4-5, SAPO-5, AlPO4-11 and SAPO-11 sorbents.
The first straight line corresponds to the external surface fast sorption. The second straight line is the gradual sorption stage. Similar two-stage kinetics was earlier reported [13]. The calculated intraparticle diffusion constants kid1, kid2 and C1 and C2 are presented in Table 5.
Intraparticle diffusion rate constants for uranium (VI) adsorption onto AEL and AFI sorbents.
Sorbents | kid1 (mg g−1 min−0.5) | C1 (mg/g) | kid2 (mg g−1 min−0.5) | C2 (mg/g) |
SAPO-5 | 1.44 | 67.88 | 0.02 | 73.46 |
AlPO4-5 | 1.46 | 47.56 | 0.02 | 53.37 |
SAPO-11 | 3.50 | 35.96 | 0.08 | 48.82 |
AlPO4-11 | 3.07 | 35.43 | 0.03 | 47.91 |
As expected, the diffusion rate kid1 in the first stage is larger than in the second (kid2). Indeed, uranium (VI) is adsorbed quickly by the external surface via film diffusion. When the external surface reaches saturation, the uranium (VI) enters the internal pores [7].
The value of the intercept C provides information related to the thickness of the boundary layer. Larger values of the intercept obtained for SAPO-5 suggest that the surface diffusion has a larger role as the rate-limiting step [27]. This behaviour may be explained by both the AFI structure and uranium speciation.
The species of uranium are influenced by pH solution, different mononuclear and polynuclear U (VI) hydrolysis products in the form [(UO2)p(OH)q](2p − q)+ as a function of pH values are present in uranium solution. The repartition is determined by the following equilibria:
(5) |
(6) |
(7) |
(8) |
(9) |
At pH between 3.0 and 5.0, the polynuclear products [(UO2)2(OH)2]2+, [(UO2)3(OH)4]2+ and [(UO2)3(OH)5]+ are present with UO22+ and [UO2(OH)]+ and are available for sorption. At pH higher than 6, the hydrolysis is more intense and other polynuclear product [(UO2)4(OH)7]+1 is formed [28]. All these species can be readily sorbed by the AFI and AEL sorbents. Moreover, at pH values higher than 7.0, carbonate uranyl ion UO2(CO3)22− and UO2(CO3)34− are formed [29,30]. The diameter of the hydrated uranyl is 6.5 Å, because the ionic radius of the uranyl ion is equal to 1.8 Å and the atomic radii of oxygen and hydrogen are 0.74 Å and 0.37 Å, respectively [5]. This diameter is less than that of the channels of the AFI and AEL structures, which consist of one-dimensional 12-membered ring pores (7.3 × 7.3 Å in size) and 10-membered ring pores (6.5 × 4.0 Å), respectively, that allow its diffusion in the AFI sorbents more favourably than onto AEL sorbents.
4 Conclusion
In the present study, AFI and AEL sorbents are synthesized successfully and characterized. According to the X-ray diffraction analysis the elaborated samples are pure phase AFI and AEL with high crystallinity. The as-prepared materials are used in the sorption of uranium (VI) ion from nuclear effluents.
Attempts for effective removal of uranium (VI) from radioactive effluents with different activity obtained from Nuclear Research Center of Draria, Algeria using SAPO-5, AlPO4-5, SAPO-11 and AlPO4-11 sorbents are made. The uptake of uranium ions sorbed onto the sorbents varies between 70 and 98% for the investigated nuclear effluents activities. Furthermore, the DFs calculated are very important for high activity effluent. Moreover, SAPO-5 exhibits a higher DF value at 323 K. The thermodynamic studies show that the sorption process is spontaneous and endothermic because of the negative ΔG value and positive ΔH value. The analyses of the experimental kinetics data of the studied sorbents by the intraparticle diffusion model indicate that the uranium (VI) is sorbed quickly by the external surface via film diffusion, when the external surface reaches saturation; the uranium (VI) enters the internal pores. This study concludes that the elaborated SAPO-5, AlPO4-5, SAPO-11 and AlPO4-11 are suitable sorbent candidates for the removal of uranium. However, sorbent with AFI structure is a highly effective material for the removal of uranium (VI) ions from nuclear effluents.
Acknowledgements
The author (N.B.) thanks Mr. Mouzai of CRND Nuclear Research Center for his cooperation in our experiments of gamma spectroscopy. The author is also grateful to Dr S. Belkhiri, teacher–researcher at the Polytechnic Military School of Algiers, and to Mr. M. Izerouken, researcher director at the CRND Nuclear Research Center for Scientific and Technical Assistance.