1 Introduction
Because of the potential applications of metalloporphyrins in catalysis, energy storage, biotechnology, optical devices, and self-assembling of functional porphyrin into nanomaterial, metalloporphyrins have been the focus of a wide range of experimental researches [1–4]. In the both homogeneous and heterogeneous oxidation reactions, metalloporphyrin complexes show excellent catalytic activity [5–7]. The recent interest in nanocatalyst is because of the unusual properties, namely perfect activity and also product selectivities [8,9]. Carbon nanotubes as one of the important types of the solid supports, which are obtained by folding graphene layers into cylinders with special morphology, have appropriate structure favoring complex dispersion for the preparation of a hybrid catalyst [10]. Most current attention to using novel “clean” methods for catalytic reactions focuses on the benefits of green oxidant and nontoxic solvents in particularly aqueous media. Water is a brilliant alternative for toxic organic solvents in oxidation reactions not only because of the hazardous effects of organic solvents on the environment but also because of the low costs of this green solvent, especially for industrial purposes [11–14]. Alternatively, in the past decades, great attention has also been paid to the application of various catalysts in the remediation of organic contaminants in wastewater [15–17]. Phenolic compounds are among the persistent organic contaminants, which exhibit low biodegradability and can pose a range of serious problems to human health. Thus, different chemical and biological strategies were applied to degrade phenol derivatives in wastewater [18–20]. In line with our recent reports on the development of new strategies for environmentally friendly catalytic oxidation of organic substrates, we attempt to study the catalytic activity of metalloporphyrins in homogeneous and heterogeneous systems, for oxidation of hydrocarbons with hydrogen peroxide in nontoxic solvents [21–23]. In this report, we discuss the catalytic activity of multiwalled carbon nanotubes (MWCNTs) immobilized with meso-tetrakis(4-carboxyphenyl)porphyrinatoiron(III) chloride (Fe(TCPP)Cl), [Fe(TCPP)Cl@MWCNT], for oxidative degradation of sulfides and phenolic compounds with urea hydrogen peroxide (UHP) in mild conditions in water.
2 Experimental section
2.1 Instruments and reagents
The electronic absorption spectra were recorded on a single beam spectrophotometer (Camspect, UV-M330) in ethanol. Fourier transform infrared (FT-IR) spectra were recorded on an ABB Bomem FTLA 2000-100 in the range of 400–4000 cm−1 using spectral-grade potassium bromide. X-ray powder diffraction (XRD) was carried out on an X-Pert MDP diffractometer with cobalt anode, typically run at a voltage of 40 kV and a current of 30 mA. Thermogravimetric analysis (TGA) and difference thermal analysis (DTA) were carried out using Bahr STA-503, and transmission electron microscopy (TEM) images were obtained using a Siemens CM 30 instrument. Gas chromatography (GC) experiments were performed using a Shimadzu GC-14B equipped with a flame ionization detector with an SAB-5 capillary column (phenyl methyl siloxane 30 m × 320 mm × 0.25 mm). Chemicals reagents and solvents were purchased from Merck or Fluka chemical companies and were used without further purification. MWCNTs containing OH groups were purchased from Shenzen NTP Factory (China). Specifications of MWCNTOH used in this study were as follows: purity, 95%; outside diameter, 10–20 nm; inside diameter, 5–10 nm; length, 30 μm; specific surface area, 200 m2 g−1; and OH content, 3.06%. The free-base porphyrin, H2TCPP, and its iron complex were synthesized as reported [24,25].
Esterification of carboxylic acids with alcohols and phenols by using 2-(1H-benzotriazole-1-yl)-1,1,3,3-tetramethyluronium tetrafluoroborate (TBTU) in the presence of triethylamine as a base proceeded smoothly under mild conditions to afford the corresponding esters in high yields in acetonitrile at room temperature.
2.2 Immobilization of metalloporphyrin on MWCNTs
The heterogeneous catalyst was prepared by a covalent bond between Fe-porphyrin and MWCNTs according to previous reports [26,27]. In this method, esterification of the hydroxyl groups of the MWCNTs with carboxylic acid groups of the porphyrin by using TBTU in the presence of N,N′-diisopropylamine (DIPEA) proceeded smoothly under mild conditions to afford the ester bonds in high yield at room temperature [26]. In brief, a mixture of MWCNTs (400 mg), Fe(TCPP)Cl (295 mg), TBTU (200 mg), and DIPEA (150 mg) was added to 30 mL DMF. The suspension was stirred at room temperature for 48 h. The solid product was filtered and washed with DMF and ethanol. Finally, the black solids were dried at 70 °C for about 24 h. The preparation of MWCNT immobilized with Fe(TCPP)Cl is shown in Fig. 1.
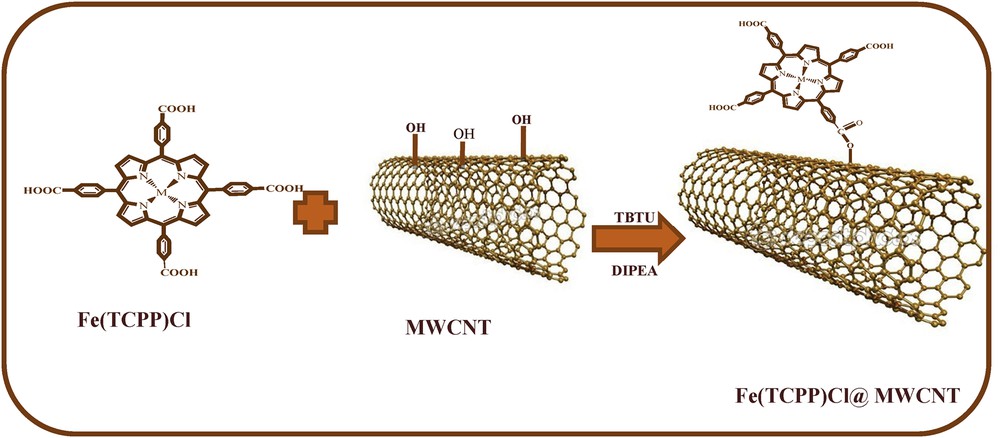
Schematic preparation of [Fe(TCPP)Cl@MWCNT].
2.3 General heterogeneous oxidation of sulfides
Catalytic experiments were carried out in a 5-mL test tube. In a typical procedure, UHP (0.16 mmol) was added to a mixture of sulfide (0.064 mmol) and catalyst (0.0032 mmol) in deionized water (1 mL), and the mixture was stirred for 90 min at room temperature. Eventually, after completion of the reaction, the organic substrate and products were extracted with 1 mL of chloroform, and the products were characterized by GC.
2.4 General heterogeneous oxidation of phenols
To a mixture of phenol (0.032 mmol) and catalyst (0.0016 mmol) in deionized water (1 mL), UHP (0.08 mmol) was added gradually. The mixture was stirred at 45 °C for 90 min, and the products were characterized by GC or high-performance liquid chromatography (HPLC).
3 Results and discussion
3.1 Characterization of the catalyst [Fe(TCPP)Cl@MWCNT]
The FT-IR spectra of MWCNT and [Fe(TCPP)Cl@MWCNT] (as KBr pellets) are presented in Fig. 2. The FT-IR spectra of the MWCNT show a sharp band at 1684 cm−1, which can be attributed to the presence of the hydroxyl group on the surface of modified nanotubes (Fig. 2a). The presence of the iron porphyrin on the nanotubes was confirmed by observation of two sharp bands at 1725 and 1387 cm−1, which is attributed to the esteric bond constructed between nanotubes and Fe-porphyrin (Fig. 2b). Also, the stretching vibration band of the phenyl rings in the meso positions appears at about 1659 cm−1 [27,28].
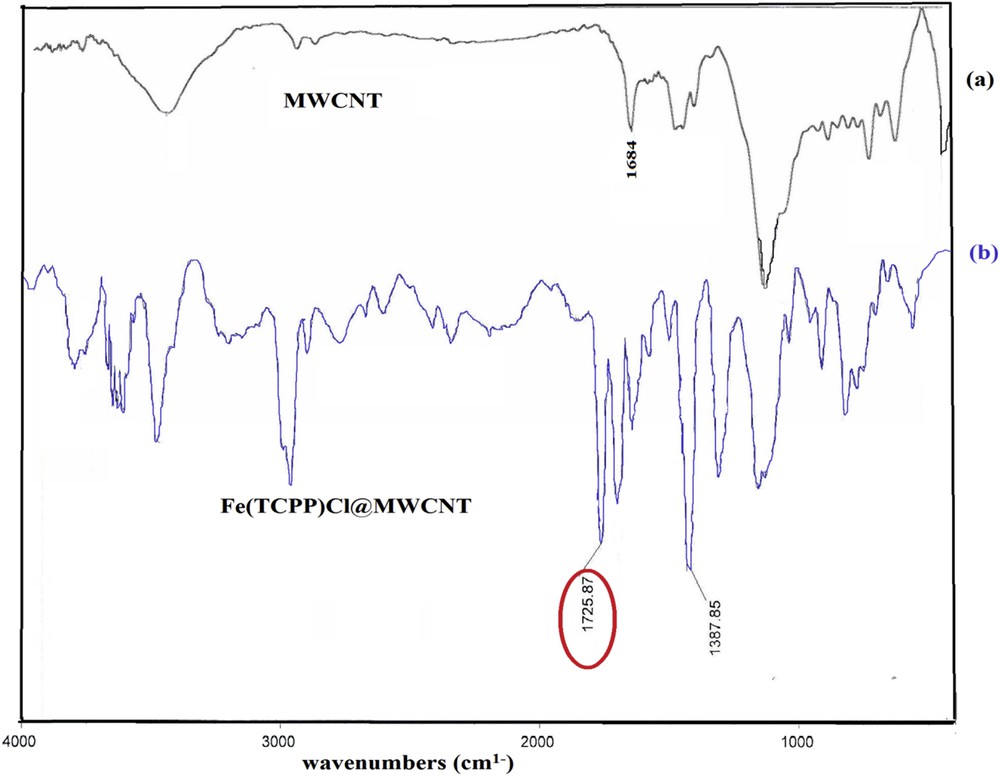
FT-IR spectra of MWCNTOH (a) and [Fe(TCPP)Cl@MWCNT] (b).
TEM of [(FeTCPP)Cl@MWCNT] displayed a good dispersion of Fe(TCPP)Cl molecules onto the surface of MWCNTs (Fig. 3). The presence of Fe-porphyrin on the surface of nanotubes is clearly detectable in the TEM image, which seems to confirm the linkage between nanotubes and metalloporphyrin.
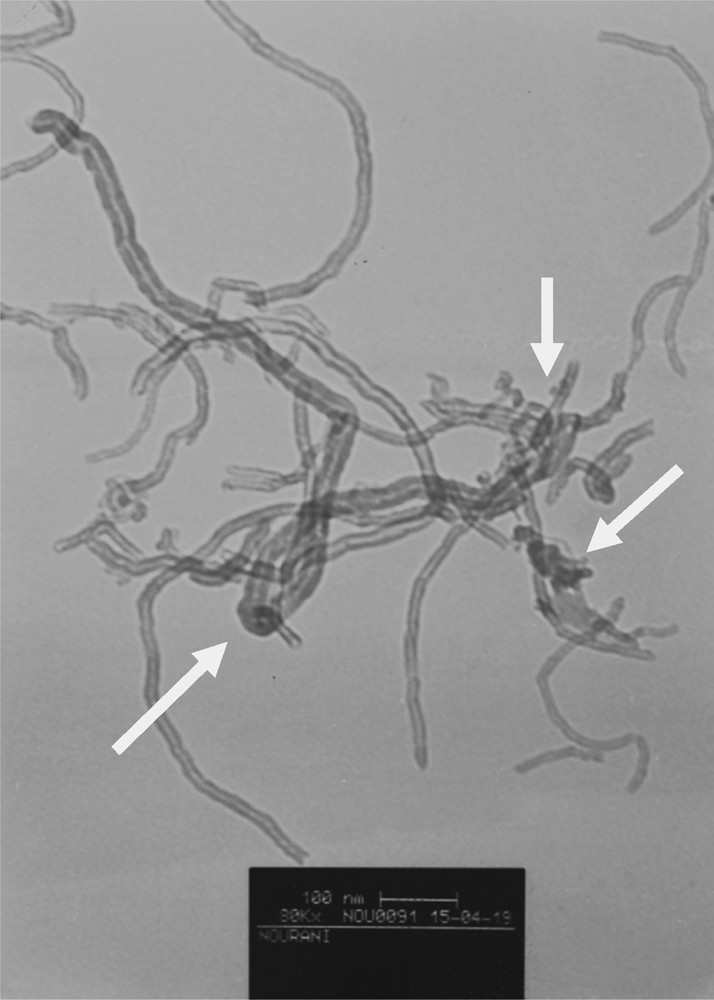
The TEM image of [(FeTCPP)Cl@MWCNT].
The percentage of the metal content of the supported catalyst was estimated by atomic absorption spectroscopy. On the basis of this value, the Fe content of the catalyst was determined to be about 500 μmol/g of the catalyst.
Fig. 4 shows XRD powder patterns of original MWCNT and [Fe(TCPP)Cl@MWCNT]. A sharp peak centered on the 2θ value of about 26° corresponds to the (002) planes of the MWCNTs, and the peaks around 43° are due to the (100) and (101) graphitic planes. These are the characteristic peaks of MWCNTs [29]. After immobilization of metalloporphyrin, no new peaks for catalyst appearance were observed, and all the mentioned peaks of nanotubes were clearly recognizable demonstrating excellent dispersion of metalloporphyrin on MWCNTs.

XRD patterns of MWCNTOH (a) and [Fe(TCPP)Cl@MWCNT] (b).
The presence of metalloporphyrin on the prepared catalyst was also confirmed by ultraviolet–visible (UV–vis) spectroscopy. The UV–vis absorption spectra of dispersed [Fe(TCPP)Cl@MWCNT] in ethanol exhibit characteristic absorption peaks nearly at the same wavelengths of homogeneous Fe(TCPP)Cl in ethanol, with a Soret band at 427 nm and a broad Q band at 660 nm (Fig. 5). These are strongly in line with the results obtained from FT-IR and atomic absorption spectroscopy, so it can be concluded that metalloporphyrins have been grafted onto the MWCNTs. Furthermore, we can conclude that the structure of Fe-porphyrin remains the same after immobilization on the surface of carbon nanotubes.
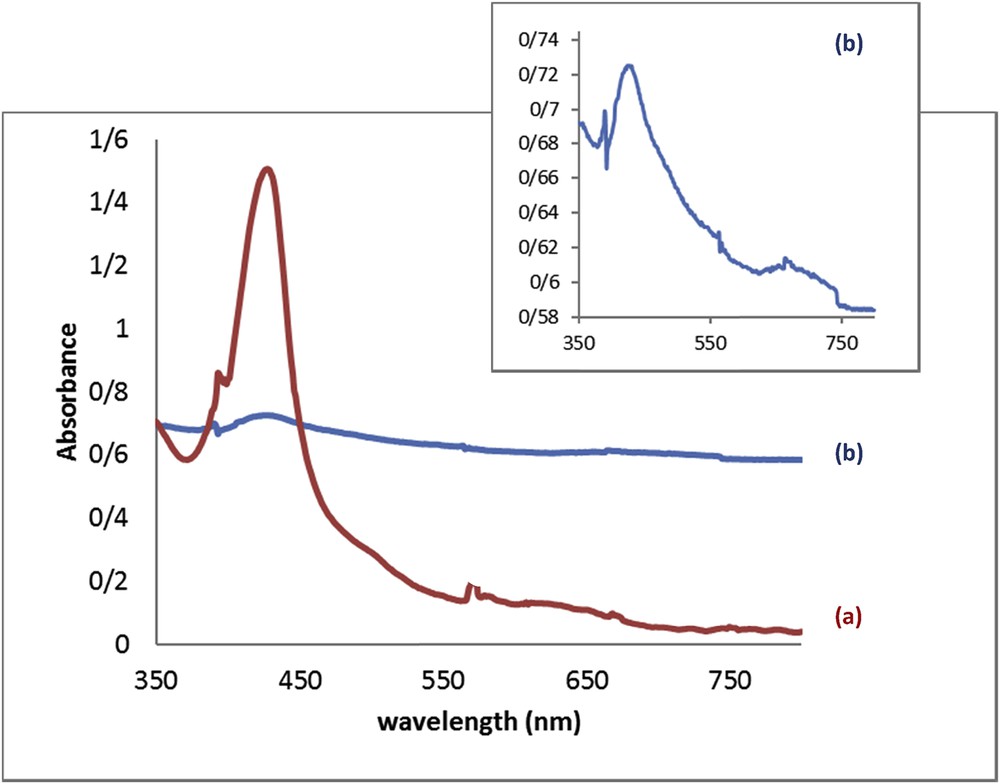
UV–vis spectra of Fe(TCPP)Cl (a) and [Fe(TCPP)Cl@MWCNT] (b) in ethanol.
The thermal response of [Fe(TCPP)Cl@MWCNT] showed the degradation of the heterogenized catalyst between 350 and 550 °C across two steps (Fig. 6), which demonstrates the high thermal stability of the catalyst. A small change in mass is detectable before 300 °C, which can be attributed to the adsorbed water on the surface of the heterogeneous catalyst and deprotonation of COOH groups [30]. The second weight decrease may be justified by the decomposition of Fe-porphyrin, followed by the formation of iron oxide and concludes in the oxidation of nanotubes. Also, approximately 9% remaining weight at the end of TGA analysis is because of the presence of iron oxide [31,32].
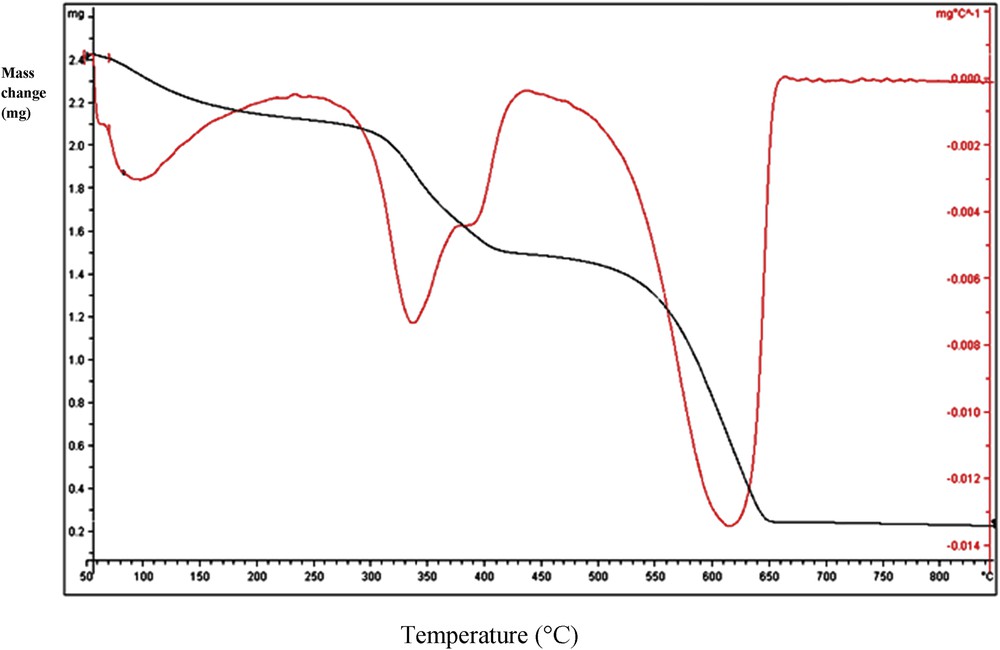
TGA/DTA curves of [Fe(TCPP)Cl@MWCNT].
3.2 Aqueous oxidation of sulfides and phenolic oxidative degradation
To evaluate the catalytic activity of the prepared catalyst, [Fe(TCPP)Cl@MWCNT] was used to oxidize the sulfides and bring out the oxidative degradation of phenols with the green oxidant (UHP) in water.
3.2.1 Aqueous oxidation of sulfides
Oxidation of methyl phenyl sulfide with UHP as a green oxidant was studied as a model reaction in the presence of [Fe(TCPP)Cl@MWCNT] in water at room temperature. This reaction did not proceed in the absence of a catalyst and also gave very low yields of methyl phenyl sulfoxide in the presence of unsupported Fe-porphyrins (Table 1). On the basis of the results, the efficiency and stability of Fe(TCPP)Cl was improved through anchoring to MWCNTs. It should be mentioned that the overoxidation reaction was completely controlled and no sulfone was observed.
The effect of different catalysts on the oxidation of sulfide using UHP in water at room temperature.a
No. | Catalyst | Conversion % | Selectivity % (sulfoxide) |
1 | None | 0 | – |
2 | Fe(TPP)Cl | 4 | 100 |
3 | Fe(TCPP)Cl | 9 | 100 |
4 | Fe(TCPP)Cl@MWCNT | 38 | 100 |
a Reaction condition: the molar ratio for catalyst:MePhS:UHP is 1:20:35, reaction time: 90 min.
Because the performance of the oxidation reaction of sulfides is dependent on a variety of parameters, such as temperature, molar ratios of the oxidant, and the amount of the catalyst, all of these factors were studied during the optimization of the reaction. In searching for the optimal molar ratios of the UHP, we screened various ratios of UHP/catalyst and a ratio of 55 was found to give the best result (Table 2, entry 3). In addition, when the reaction time was raised to 120 min, a remarkable improvement was observed in the reaction conversion whereas selectivity for sulfoxide decreased (Table 2, entry 5). After an increase in the reaction temperature from 25 to 55 °C, a slight increase in the reaction conversion was observed, whereas selectivity dramatically decreased. Therefore, the optimal reaction conditions were established at a 50:1 ratio of UHP/catalyst and a 90 min reaction time at room temperature was chosen for further investigations.
The effect of different parameters on the catalytic activity of [Fe(TCPP)Cl@MWCNT] in the oxidation of sulfide using UHP in water at room temperature.a
Entry | Amount of catalyst (mg) | UHP/catalyst | Temperature (°C) | Time (min) | Conversion % (selectivity %) |
1 | 0.006 | 35 | 10 | 90 | 38 (100) |
2 | 0.006 | 45 | 10 | 90 | 49 (100) |
3 | 0.006 | 50 | 10 | 60 | 59 (100) |
4 | 0.006 | 50 | 10 | 90 | 75 (97) |
5 | 0.006 | 50 | 10 | 120 | 85 (94) |
6 | 0.002 | 50 | 10 | 90 | 21 (100) |
7 | 0.004 | 50 | 10 | 90 | 35 (100) |
8 | 0.008 | 50 | 10 | 90 | 100 (95) |
9 | 0.006 | 50 | 25 | 90 | 96 (100) |
10 | 0.006 | 50 | 55 | 90 | 100 (67) |
a Reaction condition: the molar ratio for catalyst:MePhS:UHP is 1:20:X, reaction solvent: H2O (1 mL).
Encouraged by the impressive results obtained for the oxidation of methyl phenyl sulfide, aliphatic and aromatic sulfides were subjected to oxidation under the optimized conditions in the presence of 5 mol % of [Fe(TCPP)Cl@MWCNT] in water (Fig. 7). On the basis of the results in Fig. 7, excellent selectivity to sulfoxide was achieved for all cases in the mild condition and overoxidation was controlled. This makes the process a qualified alternative for sulfoxide production.
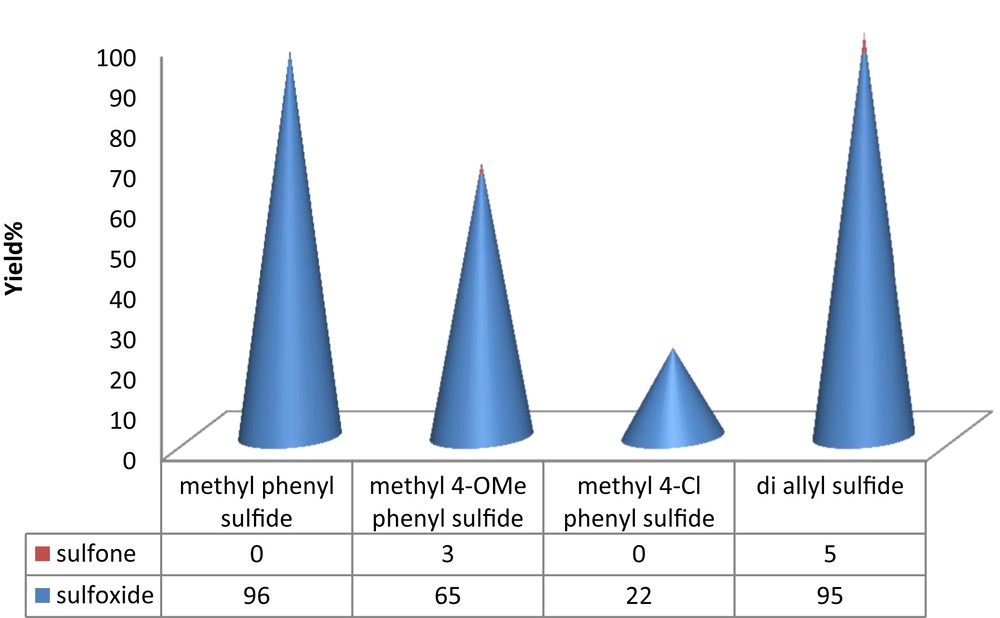
Oxidation of sulfides with UHP in the presence of [Fe(TCPP)Cl@MWCNT] at room temperature. Reaction condition: the molar ratio for catalyst:sulfide:UHP is 1:20:55, solvent: H2O (1 mL).
Highly selective oxidation of sulfides in green reaction media was one of the most challenging issues in the last decade and many experimental studies have been conducted on this aspect [33–36]. Details of the reaction condition of some of the published data have been compared by this work and presented in Table 3. Evidently, the present method has significant advantages in terms of the usage of green solvents, the usage of the green oxidant without any activator and selectivity to sulfoxide.
A comparison of the presented catalytic system with some previously reported cases for the sulfoxidation of methyl phenyl sulfide.
Entry | Catalyst | Solvent | Oxidant | Conversion % (selectivity %) | Reference |
1 | MnTPPBr4(OAc) | EtOH | UHP/HOAc | 80 (77) | [23] |
2 | Fe(TPP)Cl-MWCNT | Water | TBAOX | 92 (100) | [31] |
3 | Mn(TPP)OCN | Acetone | PVP-H2O2 | 80 (87) | [33] |
4 | Pd Schiff base | CH3CN | H2O2 | 72 (99) | [35] |
5 | Ti-MWW | – | H2O2 | 100 (89) | [36] |
6 | Fe(TCPP)Cl@MWCNT | Water | UHP | 96 (100) | This work |
In the catalytic cycle of metalloporphyrins for oxidation reactions with hydrogen peroxide, two common intermediate species have been suggested in the literature (Fig. 8) [23,33]. In the catalytic cycle, hydrogen peroxide has been coordinated to metal center to form Fe–OOH (Fig. 8a), followed by the generation of high valent metal–oxo (Fig. 8b). Direct oxygen transfer from the metal–oxo porphyrin radical cation to the sulfide is generally accepted to be the mechanism for sulfide oxidation in the presence of H2O2 and metalloporphyrins [5]. According to the previous reported data, the high valent metal–oxo species cause the overoxidation of sulfides to corresponding sulfones [33,37]. In the absence of sulfone products in this catalytic system, selective formation of the sulfoxide product at room temperature suggests the formation of FeOOH species (Fig. 8a), whereas at 55 °C (Table 2, entry 10) production of sulfone may be because of increased formation of high valent metal–oxo species (Fig. 8b).
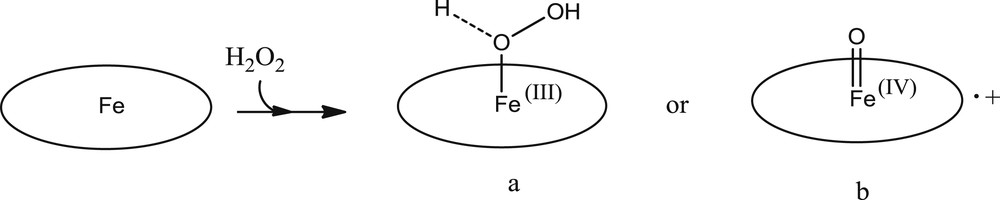
Proposed intermediate species in the catalytic oxidation reactions in the presence of metalloporphyrin.
3.2.2 Oxidation aqueous oxidation of phenols
Excellent results obtained in the oxidation of sulfides with UHP in the presence of [Fe(TCPP)Cl@MWCNT] in water made us curious to study the catalytic activity of this clean catalytic system for phenolic oxidative degradation. Oxidation of 4900 ppm of 3,5-dimethylphenol as a model substrate was studied in the presence of the heterogeneous catalyst. The effect of different reaction parameters such as temperature, molar ratios of the oxidant, and the amount of the catalyst was optimized and results are presented in Table 4. On the basis of the results (Table 4, entries 4–7), increasing the temperature slightly to 40 °C significantly increases the catalytic activity for oxidative degradation of phenols. According to the HPLC analysis results, this catalytic system for phenol degradation in water with UHP led to the production of the corresponding catechol, benzoquinone, and hydroquinone, which are important chemical materials used as pesticides, antioxidants, polymerization inhibitors, flavoring agents, photography chemicals, and medicine [38].
The effect of different parameters on the catalytic activity of [Fe(TCPP)Cl@MWCNT] in the oxidation of phenol using UHP in water at room temperature.a
Entry | Temperature (°C) | Time (min) | UHP/catalyst | Conversion % |
1 | 25 | 90 | 45 | 3 |
2 | 25 | 90 | 55 | 10 |
3 | 25 | 90 | 65 | 12 |
4 | 40 | 20 | 55 | 21 |
5 | 40 | 60 | 55 | 63 |
6 | 40 | 90 | 55 | 97 |
7 | 40 | 120 | 55 | 100 (14)b |
a Reaction condition: the molar ratio for catalyst:2,5-dimephenol:UHP is 1:20:X, reaction solvent: H2O (1 mL).
b Without catalyst.
Next, to establish the degradation activity potential of this catalytic system, oxidative degradation of various phenols with UHP was carried out in the presence of a catalytic amount of [Fe(TCPP)Cl@MWCNT] in neat water under the optimized reaction conditions (Table 5). The desired conversion was obtained within 90 min in the mild condition for all phenols. According to the results, it seems that the efficacy of this catalytic system is closely dependent on the electronic and steric demands of the substrate.
Oxidative degradation of phenols with UHP in the presence of [Fe(TCPP)Cl@MWCNT] in water in the mild condition.a
Entry | Phenol | Conversion % |
1 | Image 2 | 98 |
2 | Image 3 | 87 |
3 | Image 4 | 85 |
a Reaction condition: the molar ratio for catalyst:phenol:UHP is 1:20:55 at 40 °C, solvent: H2O. Reaction time: 90 min.
4 Conclusions
Selective oxidation of sulfides to their corresponding sulfoxides and the oxidative degradation of phenols by using UHP as a safe oxidant in aqueous media were both efficiently enhanced in the presence of a Fe-porphyrin grafted onto MWCNTs. No toxic solvents or byproducts were involved, and no laborious purifications were necessary. This, together with the use of water standard “green” solvents and wide substrate range, makes this cost-effective, environmentally benign methodology suitable for applied goals.
Acknowledgments
The financial support of this work by K.N. Toosi University of Technology research council is acknowledged.