1 Introduction
Natural antioxidants have been the focus of ever-growing amounts of interest in recent years because they have been linked to health benefits that reduce the risks of developing chronic diseases, such as cardiovascular disorders and cancer. Moreover, there appears a general perception among consumers that an intake of natural antioxidants is safer than synthetic analogs, meaning that the formulation of functional foods and nutraceutical products has become a hot topic.
Polyphenols possess well-known antioxidant capacity because of their ability to inhibit free radical generation, free radical scavenging activity, and the capability to chelate transition metal ions [1]. This activity, which is thought to be even stronger than that of vitamins C and E for many polyphenols [1,2], has led to increasing attention being paid to plants that are now being investigated both for their secondary metabolite composition and antioxidant activity [3].
Rosmarinic acid (RA) (Fig. 1) is a phenolic compound whose antioxidant activity has been well documented by a large amount of literature data, including in vivo studies, some of which regard their efficacy in reducing diabetes risks [4]. Although rosemary [5] is thought to be the main source of RA, the steady growth of pharmaceutical, cosmetic, and food products that contain this polyphenol has driven the search for other plants that bear high amounts of RA and, at the same time, the study of techniques that can provide rich extracts of this compound.
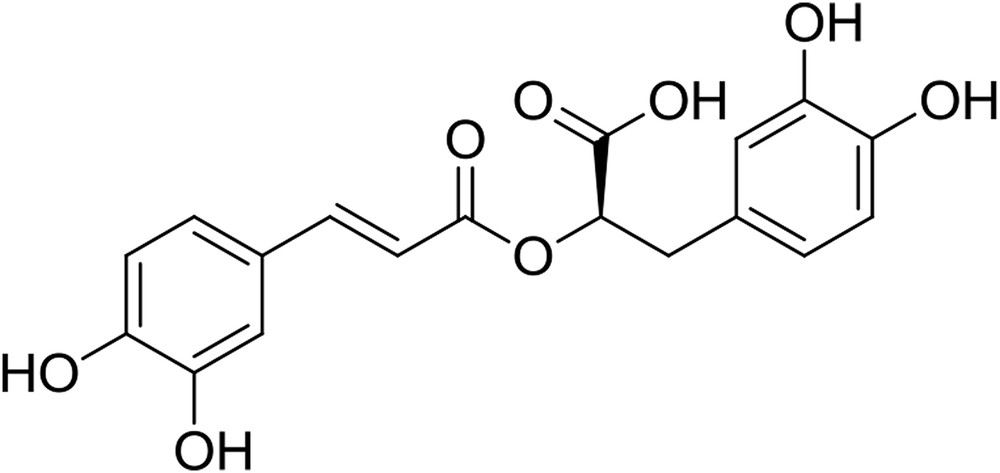
Rosmarinic acid.
An alternative source of polyphenols is lemon balm (Melissa officinalis L.), an aromatic perennial herb belonging to the mint family, Lamiaceae. Well known for its culinary properties, this plant is used for a variety of cognitive purposes, most of which center around improving cognition and reducing stress and anxiety [6,7]. Recent evidence suggests that lemon balm possesses several other beneficial properties, such as antioxidant, antimicrobial, and antitumor effects [8,9]. Of the constituents present in this herb, it is mainly the phenolic fraction, and principally RA, that possesses the properties mentioned [10,11]. It has recently been highlighted that lemon balm extracts are safe to use as food supplements in healthy individuals [12] and that they can provide relief for heart benign palpitation in humans [13], confirming the interest toward this plant.
The intensification of the extraction process using highly efficient green techniques is currently a very attractive topic [14,15], as the community aims to produce extracts that are richer in bioactive compounds to be used in the formulation of functional foods and nutraceutical products. It is also generally accepted that phenolic compounds can suffer from thermal degradation, meaning that extended exposure to high temperatures, as occurs in some conventional solvent extraction methods, should be avoided.
Supercritical carbon dioxide extraction, ultrasound-assisted extraction (UAE), and microwave-assisted extraction (MAE) are efficient, unconventional techniques used for lemon balm treatment [10,16–18]. In particular, UAE and MAE are green technologies that could dramatically reduce extraction times and solvent volumes and at the same time increasing product yields and protecting the extract from thermal degradation [19–23].
Ince et al. [24] have recently compared the microwave (MW) and ultrasound (US) effects of phenolic extraction from lemon balm and found that MW extraction is the most advantageous in terms of yield and extraction time. Moreover, Nicolai et al. [25] have showcased a rapid and effective US-assisted process that is able to preserve the antioxidant activity of ethanolic M. officinalis L. extracts.
The stability, solubility, and bioavailability of natural bioactive compounds can be increased by cyclodextrins (CDs), polysaccharides that are well known for their ability to form inclusion complexes with several kinds of molecules [26]. This property has also been exploited to selectively extract bioactive compounds from plants [27]. The complexation of poorly water-soluble bioactive molecules in CDs can be achieved through solventless grinding in a ball mill, thus enhancing oligosaccharide and guest molecule interactions [28–30]. Mechanochemistry is, in fact, an enabling interdisciplinary research field that can present advantages in terms of extraction time, simplicity, and waste reduction [31]. These features could be exploited for the selective green extraction of natural compounds via the cogrinding of vegetal matrices with CDs in the solid state.
To use lemon balm extracts with high polyphenolic acid contents as natural additives in functional foods, extraction procedures that selectively enrich the product in the RA content would be useful [32–34], as they can limit or completely avoid the need for subsequent purification processes.
The present work evaluates the efficiency and selectivity of UAE and MAE dried lemon balm aerial part extraction processes by testing a variety of solvents. Pure ethanol, 70% ethanol, and pure water were chosen for MAE, whereas two sequences of solvents with increasing polarity were used for UAE: n-hexane (Hex), ethanol (EtOH), water (sequence 1); Hex, acetone (Ace), EtOH, water (sequence 2). We also investigated the dry extraction of a rewetted vegetal material in a ball mill in the presence of β-cyclodextrin (β-CD). Extraction yields, total phenols, and RA content were determined in the final extracts of each sample using an high-performance liquid chromatography-diode array detector (HPLC-DAD) method with the help of RA as the sole external standard.
2 Results and discussion
This work evaluates the efficacy of US and MW in the rapid and selective recovery of phenolic compounds from M. officinalis L. leaves. An extraction time of only 10 min and solvents with different polarities (Hex, Ace, and EtOH) were used in accordance with our previous experience with rosemary leaves [35]. Longer extraction times (20 and 30 min) did not improve extraction yields. Extraction efficiency was tested across a series of single-extraction step (MAE) and sequential procedures (UAE), carried out on the same sample batch. Yields, in terms of percentage of dried extract (DE) weight obtained over dried leaves (DL) weight for each sample, are reported in Table 1. Single step MAE, both in EtOH and EtOH/water 7:3 v/v, gave yields near 16%, whereas MW irradiation in pure water afforded the highest extraction yield (31.3%). The two US-assisted sequences started with apolar solvents and finished with water. Hex (UAE 1a and 2a) gave yields near 1%, acetone (UAE 2b) 1.83%, whereas higher yields (near 3.5%) were reached with EtOH (UAE 1b and 2c). The last step with water (UAE 1c and 2d), suitable for coextracting several polar components, showed the highest yields that ranged from 16% to 22%. The global extraction yield values for both the US-assisted sequences (26.5% and 22.7%, respectively, for UAE 1 and 2) fell between those of single step MAE in EtOH and water (around 16% and 31%, respectively), whereas ball mill extraction gave a lower value (11%), calculated on the weight of the relative methanol dry extract after the removal of β-CD.
Extraction yields expressed as a percentage of DE weight obtained over DL weight for each sample.
Extraction method-solvent | Yield (w/w %) |
MAE-EtOH | 16.8 |
MAE-water | 31.3 |
MAE-EtOH/H2O 7:3 | 16.1 |
Sequence 1 | |
UAE 1a-Hex | 1.20 |
UAE 1b-EtOH | 3.60 |
UAE 1c-water | 21.7 |
26.5 | |
Sequence 2 | |
UAE 2a-Hex | 1.14 |
UAE 2b-Ace | 1.83 |
UAE 2c-EtOH | 3.45 |
UAE 2d-water | 16.3 |
22.7 | |
Ball mill–β-CD | 11.0 |
The structural identification of the various phenolic compounds in the extracts was carried out according to previous studies [35,36], and via our HPLC-DAD findings. The phenolic distribution of the MAE-EtOH extract is summarized in Fig. 2. It can be seen that, similarly to all other extracts, it contains RA and several minor phenols. These other compounds include cinnamic (C, D, and E) and flavonoidic (F) derivatives, as well as some nonidentified compounds (A and B).
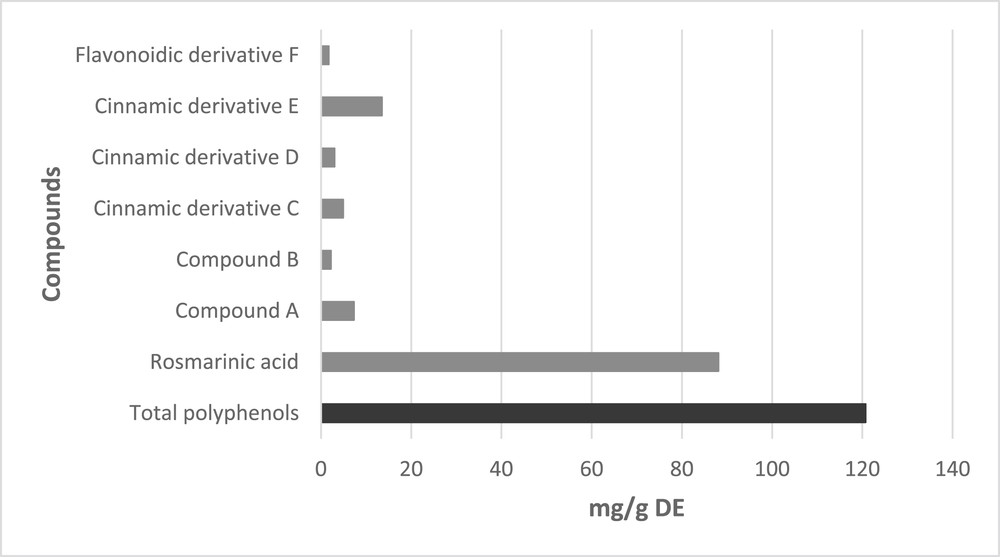
Characterization obtained from HPLC-DAD analysis of MAE-EtOH Melissa officinalis L. leaf extract.
The phenolic content was expressed as milligrams of total phenolic compounds (TP) and milligrams of RA per gram of DL (Table 2) and per gram of DE (Fig. 2). The TP indicates the sum of all the phenolic molecules determined by HPLC-DAD and that were recognized from their UV–Vis spectra and retention times. Furthermore, RA was used as the sole external standard for the preparation of the calibration curve and to express the data as it is the main component of all the extracts.
Extractive yields as milligrams/gram of DL evaluated as TP and RA amounts obtained from Melissa officinalis L. leaves.
Extraction method-solvent | TP (mg/g DL) | RA (mg/g DL) | % RA over TP |
MAE-EtOH | 20.30 | 14.80 | 72.90 |
MAE-H2O | 13.60 | 11.70 | 86.0 |
MAE-EtOH/H2O 7:3 | 8.37 | 6.91 | 82.0 |
UAE 1a-Hex | 0.005 | – | |
UAE 1b-EtOH | 1.57 | 0.83 | 52.70 |
UAE 1c-H2O | 0.54 | – | |
UAE 2a-Hex | – | – | |
UAE 2b-Ace | – | – | |
UAE 2c-EtOH | 4.63 | 4.11 | 88.70 |
UAE 2d-H2O | 0.35 | 0.16 | 45.90 |
Ball mill | 3.94 | 3.18 | 80.70 |
The goal of an extractive procedure is usually to combine the highest possible recovery of the target molecules (exhaustive extraction) with the final high quality of the extract. The evaluation of these aspects can be performed by measuring the ability to extract compounds from the raw material (data in milligrams/gram of DL) and evaluating selectivity (data in milligrams/gram of DE); results often show high extractive yields but low selectivity.
The best phenolic component extraction from raw material (Table 2) results were indubitably achieved under MAE-EtOH, with 20.3 mg/g of TP and 14.8 mg/g of RA.
Although water is not the preferred solvent for TP extraction because of its low selectivity (<50 mg/g TP on DE), MAE-H2O gave interesting results. Its high extraction yields mean that the total amounts of TP and RA in DL (13.6 and 11.7 mg/g, respectively) were quite close to MAE-EtOH values. This result confirms those previously reported by Ince et al. [24] by reaching 39.8 mg of RA in the aqueous extract obtained after 5 min of extraction time (solvent ratio of 1 g/30 mL) at a constant MW power of 407 W from 5 g of dry aerial part. The same authors revealed that 24 h of maceration in water at 40 °C lead to lower RA recovery, 41%.
The extractive yields obtained by US were consistently lower for all the tested solvents, only ethanol, after hexane and acetone (UAE 2c-EtOH) lead to 4.63 mg/g of TP being recovered at high selectivity for RA (4.11 mg/g DL).
Kim et al. [32] optimized RA extraction conditions in an Erlenmeyer flask, using the response surface methodology, by shaking a mixture of plant/solvent (1 g DL/20 mL of solvent) in a water bath at 100 rpm. A maximum yield of 46.1 mg RA/g DL was obtained using a methanol concentration of 59.0% (v/v), an extraction temperature of 54.8 °C, and an extraction time 64.8 min. A purity of 11% RA was achieved after the extract filtration, concentration, and freeze-drying process. Extract purification by column chromatography, using Sephadex LH-20 and methanol as the eluent, then allowed the authors to reach an RA purity of 38.8%. This conventional extraction certainly leads to extracts with a higher RA content, but does so in a much more laborious and less selective procedure.
The mechanochemical approach to TP extraction showed good selectivity, namely RA over TP close to 80% (3.18 mg/g), which is comparable to the values obtained from MAE-EtOH and UAE 2c-EtOH, although it did not give a high extractive yield (3.94 mg/g).
Fig. 3 summarizes extract composition results, expressed as TP and RA concentration per gram of DE.
- These quantitative results confirmed observations made under classic maceration in our preliminary tests with lemon balm leaves and our previous investigation on Rosmarinus officinalis L. [37], in which the highest RA yield was achieved in the third step with ethanol of the same US-assisted sequence with 67.7 mg/g DE.
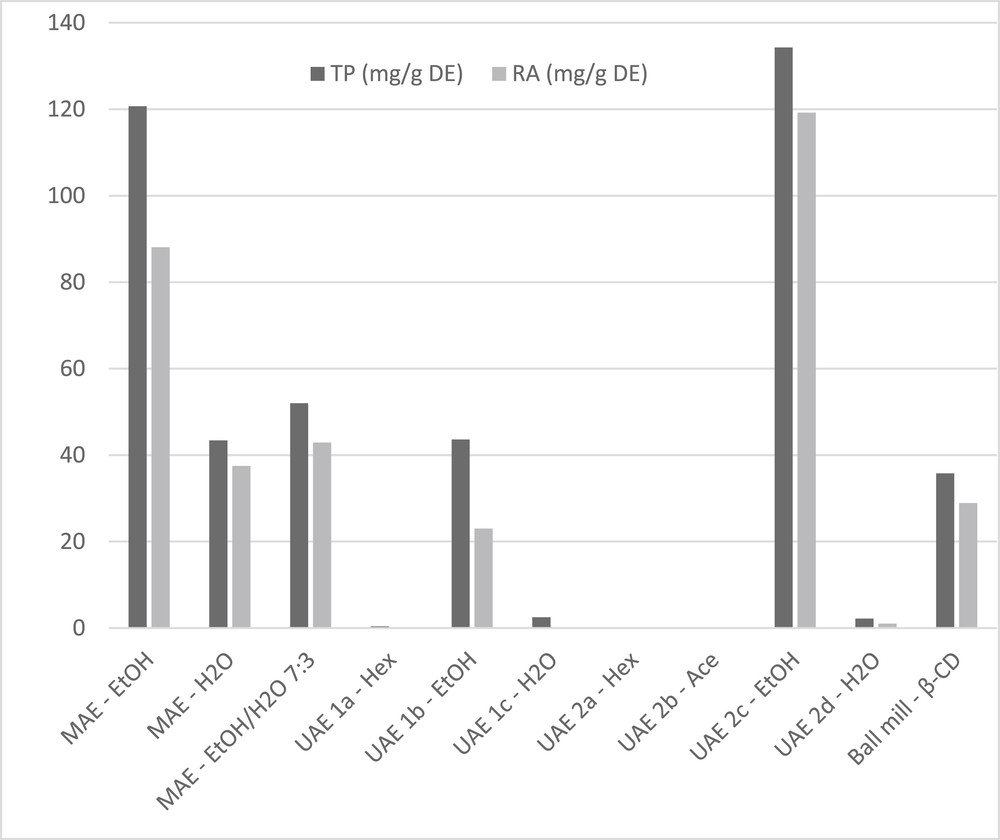
TP and RA ratio in all the dry extracts obtained from Melissa officinalis L. leaves.
As described below, a comparison between our data and those previously reported underlines the advantages that US- and MW-assisted techniques provide in terms of time required, selectivity, and sustainability, thanks to reduced solvent consumption. Moreover, it is confirmed that faster treatment of the vegetal matrix prevents the degradation of secondary metabolites. Lin et al. [34] have achieved a TP amount of 138.42 mg/g of DE with an RA quantity of 87.33 mg/g of DE (63%) in an ethanolic lemon balm extract that was produced by stirring 10 g of DL in 500 mL of a solvent at 25 °C for 24 h. Our results with UAE 2c-EtOH gave similar TP contents, but higher selectivity for RA (88.7%). Petenatti et al. [9] reported an RA amount of 3.48 mg/g of DE when the dried M. officinalis material was subjected to enzyme-assisted extraction with cellulase, endo-β-1,4 xylanase, and pectinase in a phosphate–citrate buffer at pH 5, whereas a concentration of 90.53 mg/g of DE was obtained in an ethanolic extract derived from pressurized liquid extraction at 150 °C for 20 min.
As expected, Hex and Ace extraction steps in UAE sequences did not afford extracts containing TP, but influenced the selectivity of the subsequent step in EtOH. In particular, pretreatment with acetone allowed the extractive capacity of EtOH (UAE 2c > UAE 1b) to be improved. Overall, data in Table 2 and Fig. 3 show that mean RA contents of between 72% and 88.7% were achieved, with only two lower values, of close to 50%, being obtained by UAE (Table 2). MAE can therefore be considered as the most suitable method with which to combine exhaustive extraction with high, final extract quality, as evaluated in terms of TP and RA content.
3 Conclusions
UAE and MAE were fast and efficient procedures for the extraction of polyphenolic compounds from lemon balm. RA is generally always the main component of TP, whereas EtOH was the best solvent for both extraction techniques. The best results, in terms of extractive yields of phenols and, in particular, of RA, were achieved using MAE in EtOH.
4 Experimental section
4.1 Plant materials, solvents, and chemicals
M. officinalis L. leaves were collected in the Florentine countryside. Acetone and ethanol (ACS grade, ≥99%) were used for extractions (Sigma–Aldrich). Acetonitrile CHROMASOLV (gradient grade, for HPLC, ≥99.9%) and formic acid (ACS grade, ≥99.5%) were purchased from Sigma–Aldrich for HPLC analyses, whereas Milli-Q H2O was obtained in the laboratory from a Milli-Q Reference A+ System (Merck Millipore). A standard of RA was purchased from Sigma–Aldrich.
4.2 Extractions
DLs from the same batch of M. officinalis L., dried at room temperature in the dark for several days, were used for the various extractions. All the extraction procedures in this work are summarized in Table 1.
4.2.1 UAE
UAE was carried out by means of a probe system (Danacamerini-Turin) equipped with a titanium horn (Ø = 15 mm) with a conical tip (Ø = 25 mm) working at 19.5 kHz (150 W, mean temperature near 45 °C). Wider tip diameters have less amplitude but can accommodate larger extraction volumes and ensure more homogeneous sonication. On the basis of a previous study [35], we chose a plant/solvent ratio of 1 g of DL/10 mL of a solvent and an extraction time of 10 min. A series of different sequential extractions were applied, as shown in Table 1. In sequence 1, Hex was used in the first extraction step followed by EtOH (second step) and water in the last extraction cycle, whereas Hex was followed by Ace, EtOH, and finally water in sequence 2.
4.2.2 MAE
MAE was performed in a closed multimode reactor (Synthwave, Milestone, Bergamo, Italy) under N2 (20 bar) at 100 °C (maximum power 500 W). As highlighted previously [35], we chose a plant/solvent ratio of 1 g of DL/10 mL of a solvent and an extraction time of 10 min. EtOH, water, and EtOH 70% v/v were used as solvents in a single extraction step.
4.2.3 Dry extraction in ball mill
One gram of the rewetted vegetal material was cogrinded in the presence of β-CD (1:2 w/w) for 40 min at 350 rpm in a planetary ball mill (PM100 Retsch GmbH), using 50 mL grinding stainless steel jars and milling balls (mixture of 48 × 5 mm and 1500 × 2 mm; stainless steel). The dark green paste obtained was poured into a Büchner funnel with a sintered glass disc and washed with abundant amounts of water (100 mL). The filtered solution was freeze-dried overnight, the β-CD complexes were re-extracted with methanol, and the residual β-CD was filtered off using a paper filter.
4.3 HPLC-DAD analyses
All extracts were dissolved in a defined solvent volume and the solutions were directly analyzed using HPLC-DAD.
The analyses were carried out using an HP 1100L liquid chromatograph equipped with a DAD detector (Agilent Technologies, Palo Alto, CA, USA). Applied conditions were as described in a previous study [36]. A 150 mm × 2 mm i.d., 4 μm Fusion, RP18 column (Phenomenex, USA) equipped with a precolumn of the same phase was used. The mobile phases were (A) 0.1% formic acid/water and (B) CH3CN. The multistep linear solvent gradient used was 0–15 min at 15%–25% B, 15–25 min at 25%–35% B, 25–35 min at 35%–50% B, 35–40 min at 50%–100% B with a final plateau of 8 min at 100% B. Equilibration time was 10 min, the flow rate 0.2 mL min−1, and oven temperature 26 °C, whereas the injection volume was 5 μL. Quantitative determination of the phenolic compounds was performed using RA at 330 nm as an external standard, the calibration curve was in a linearity range of between 0.1 and 9.4 μg with an R2 of 0.9999.
Acknowledgments
This work has been supported by the University of Turin (Fondi Ricerca Locale 2014 and 2015). We are also grateful to Ente Cassa di Risparmio di Firenze for supplying a part of the instrumentation used in this research.