1 Introduction
Like aziridines, epoxides are well-known ring-strained heterocyclic systems in organic chemistry. The incredible reactivity of epoxides is accountable for the synthesis of a wide range of interesting molecular entities, and hence, these have been preferred as starting materials to design a variety of novel chemical transformations under controlled reaction pathways [1]. Generally, epoxides are vulnerable to several chemical species such as nucleophiles, electrophiles, acids, bases, and oxidizing and reducing reagents. The aminolysis of epoxides constitutes a well-acknowledged route in the literature [2], as the resulting product β-amino alcohols represent a broad range of β-adrenergic blockers. They have a diverse range of clinical applications in the treatment of cardiovascular diseases such as hypertension, angina pectoris [3,4], cardiac arrhythmias [5], and open angle glaucoma [6] and also reported to have diverse applications in medicinal chemistry [7]. Apart from this, they also find utility in the synthesis of biologically active synthetic and natural products [8], un-natural amino acids [8a,9], ligands in asymmetric synthesis and auxiliaries [9], and more recently as organocatalysts [10]. Literature reveals several routes for the synthesis of β-amino alcohols, but most of these are plagued with one or more drawbacks such as the use of strong acid salts (metal triflates) [2c,d,v], long reaction times [2a,c,d], excess solvents [2f], heavy-metal Lewis acids [2b,f], elevated temperature and pressure [2r–v], supercritical carbon dioxide (sc-CO2) [2w], poor regioselectivity especially with metal amides [2z], and the use of expensive, toxic, and stoichiometric amounts of reagents. On the other hand, epoxides rearrange and polymerize under acidic and basic conditions, which decreases the overall productivity of the process.
Recent reports by Yadav et al. demonstrating the use of active nanocrystalline sulfated titania (catalyst) [11a] and Sakthivel et al. describing MCM-22 as a catalyst [11b] for the regioselective ring opening of epoxides are an indication of renewed interest in the field of ring-opening reactions to generate β-amino alcohols. Therefore, design and development of an environmentally benign route for the regioselective synthesis of β-amino alcohols is still meaningful.
Over the past few decades, mechanochemical synthesis (MCS) has attracted attention of many synthetic chemists as an amazing conventional technology [12]. Friščić and Do have described mechanochemistry as the ‘force of synthesis’ [12d]. Various types of chemical transformations such as Knoevenagel condensations [13a], aldol condensations [13b], Dieckmann condensations [13c], Grignard reactions [13d], Reformatsky reactions [13e], reductions [13f], click reactions [13g], and others [14] have been performed using the MCS technique. Motivated by the efficacy of MCS technique, efforts have been put forth for the regioselective aminolysis of epoxides using aqueous LiBr under ordinary mortar–pestle grinding to deliver β-amino alcohols in good to excellent yield.
Furthermore, the developed protocol has been successfully applied to generate a novel series of benzotriazole-based β-amino alcohols as potential drug candidates owing to the fact that heterocyclic β-amino alcohols are the core component of many known β-blockers (Fig. 1) [15]. Furthermore, benzotriazole-based organic compounds are also known to embrace a wide range of activities [16], such as antifungal [17,18], antibacterial [18,21], anticancer [19], anti-HIV agents [19,20], anticonvulsant [21], and anti-inflammatory [21]. It is worth mentioning that the present exploration is an expansion of our efforts for the development of new synthetic routes for the synthesis bioactive molecules [22].

β-Amino alcohols containing heterocyclic moiety as a core component.
2 Results and discussion
At the outset, styrene oxide and aniline were selected as a model substrate for mortar–pestle grinding in the presence of two drops of water. A reasonable progress of reaction was observed (Table 1, entry 1), which gave us a clue that grinding under aqueous condition could be a better combination for the ring opening of epoxides. This observation may be correlated with the earlier findings by Saidi et al. [2a] from aqueous point of view. Subsequently, lithium bromide (10 mol %) was introduced to boost the aminolytic process. Delightfully, the reaction was completed in just 15 min with much improved yield (Table 1, entry 2). Choice of a lithium-based catalyst is simply because of its recyclability and the oxophilicity of lithium cation, which has been extensively discussed in the literature [23].
Optimization of reaction conditions for epoxide ring opening.a
Entry | Catalyst (mol %) | Solvent (two drops) | Time (min) | % Yield (3a)b |
1 | No catalyst | Water | 30 | 55 |
2 | LiBr (10) | Water | 15 | 98 |
3 | LiBr (5) | Water | 15 | 92 |
4 | LiBr (15) | Water | 15 | 98 |
5 | NaCl (10) | Water | 20 | 70 |
6 | LiI (10) | Water | 15 | 75 |
7 | LiCl (10) | Water | 20 | 70 |
8 | NaBr (10) | Water | 20 | 65 |
9 | AlCl3 (10) | Water | 15 | 75 |
10 | AlBr3 (10) | Water | 15 | 78 |
11 | FeCl3 (10) | Water | 15 | 80 |
12 | LiBr (10) | Toluene | 20 | 65 |
13 | LiBr (10) | 1,4–Dioxane | 25 | 65 |
14 | LiBr (10) | THF | 25 | 60 |
15 | LiBr (10) | DMF | 25 | 52 |
16 | LiBr (10) | DMSO | 25 | 67 |
17 | LiBr (10) | PEG-200 | 20 | 66 |
18 | LiBr (10) | Water | 30 | 65c |
19 | LiBr (10) | Neat | 25 | 87 |
20 | LiBr (10) | Neat | 40 | 90d |
a Reaction was carried out using 1a (1 mmol) and 2a (1.1 mmol).
b Isolated yield of purified product 3a.
c Reaction carried out at 30 °C using magnetic stirring.
d Reaction perfomed at 75 °C under the magnetic stirring.
Next, LiBr charge was optimized. At 5 mol % loading, yield decreased marginally to 92% (Table 1, entry 3); however, with 15 mol % loading, the corresponding product was obtained in 98% (Table 1, entry 4). Additional acid catalysts were evaluated for further optimization (Table 1, entries 5–11) but LiBr was found to be the best, indicating a better Lewis acidity furnished by the Li+(aq).
Different solvents were screened to evaluate the solvent effect (Table 1, entries 12–17); however, water was found to be the best both in terms of yield and reaction time (Table 1, entry 2). This is presumably due to the formation of Li+ ions in water, which in turn coordinate with the oxygen of the epoxide under continuous grinding. It is important to mention that water not only facilitates the aminolytic process, but also promotes in effective grinding of the reaction mixture.
Furthermore, it is pertinent to mention that LiBr-promoted ring opening of epoxides has been reported earlier under solvent-free conditions [23d]. However, the present study is focused on the aqueous mechanochemical grinding technique and further extended to generate a wide range of newer benzotriazole-based β-amino alcohols with an additional advantage of time economy and overall efficiency of the process.
Furthermore, grinding has also been observed to have a distinct effect over the reaction progress as same reaction under magnetic stirring was found less effective at ambient conditions (Table 1, entry 18). This is probably due to activation of covalent bonds [12e] of the strained oxirane ring by grinding akin to that of the literature records where CN bond formation processes have been realized through mechanochemical activation of covalent bonds [12f–i].
To generalize this protocol, optimized reaction conditions were extended to other epoxides including benzotriazole glycidyl ethers (1d,e) [24] using a variety of aliphatic and aromatic amines as nucleophile. The reaction proceeded well, relatively with both aliphatic and aromatic amines to produce the desired product in excellent yield (Table 2). Sterically hindered amines such as o-methylaniline, o-nitroaniline, and o-trifluoroaniline could not afford significant yield (15–20%) despite using high catalyst loading (up to 40 mol %) and reactants were recovered after reaction workup. On the other hand, p-nitroaniline and p-trifluoromethylaniline gave good product yields using 20 mol % catalyst loading (Table 2, entries 4, 5, 12, 13, and 23). The structure of new products was characterized by 1H NMR, 13C NMR spectra, and HRESIMS data; however, the structure of the known compounds was confirmed and authenticated by comparison of their physical and spectral data with that of reported in the literature [2d–f,i,o,s]. Furthermore, in case of cyclohexene oxide, trans-stereochemistry of the products was confirmed through coupling constants [2f].
Synthesis of β-amino alcohols from epoxides using conventional mortar–pestle grinding technique.a
Entry | Epoxide (1) | Amine (2) | Product (3) | Time (min) | Yieldb,c (%) |
1 | 2a: aniline | 15 | 98 | ||
2 | 2b: p-chloroaniline | 10 | 96 | ||
3 | 2c: p-methoxyaniline | 10 | 98 | ||
4 | 2d: p-nitroanilined | 15 | 80 | ||
5 | 2e: p-trifluoromethylanilined | 15 | 80 | ||
6 | 2f: o-trifluoromethylanilinee | 20 | 15 | ||
7 | 2g: o-nitroanilinef | 20 | – | ||
8 | 2h: o-methylanilinee | 20 | 20 | ||
9 | 2a: aniline | 20 | 96 | ||
10 | 2b: p-chloroaniline | 20 | 90 | ||
11 | 2c:p-methoxyaniline | 20 | 95 | ||
12 | 2d: p-nitroaniline | 20 | 80 | ||
13 | 2e: p-trifluoromethylaniline | 20 | 80 | ||
14 | 2f: o-trifluoromethylanilinee | 20 | 15 | ||
15 | 2g: o-nitroaniline | 20 | – | ||
16 | 2h: o-methylanilinee | 20 | 20 | ||
17 | 2a: aniline | 10 | 98 | ||
18 | 2i: isopropylamine | 10 | 96 | ||
19 | 2j: tertiary butylamine | 10 | 96 | ||
20 | 2a: aniline | 10 | 95 | ||
21 | 2b: p-chloroaniline | 10 | 96 | ||
22 | 2c:p-methoxyaniline | 10 | 98 | ||
23 | 2d: p-nitroaniline | 15 | 90 | ||
24 | 2k: p-bromoaniline | 10 | 96 | ||
25 | 2a: aniline | 10 | 96 | ||
26 | 2k: p-bromoaniline | 10 | 97 | ||
27 | 2b: p-chloroaniline | 10 | 97 | ||
28 | 2c: p-methoxyaniline | 10 | 98 | ||
29 | 2l: p-toluidine | 10 | 98 | ||
30 | 2i: isopropylamine | 10 | 97 |
a Reaction was carried out by taking 1:1.1 ratio of 1 and 2 (in the case of isopropylamine and tertiary butylamine, 2.5 equiv of amine was required and added in installments).
b Yield of isolated and purified products.
c All compounds gave C, H, and N analysis within ±0.4% and satisfactory spectral (IR, 1H NMR, 13C NMR, and ESI-MS) data.
d In case of p-nitroaniline/trifluoromethylaniline, 20 mol % of LiBr was used.
e In case of o-methylaniline/trifluoromethylaniline, 40 mol % of LiBr was used.
f In case of o-nitroaniline, analytically pure compounds could not be isolated.
The noteworthy feature of this protocol lies in the mild catalytic aminolysis of epoxides under aqueous grinding. This is because the reaction took relatively longer time in the absence of aqueous grinding even at high temperature, which reveals the significance of the grinding in the envisaged protocol (Table 1, entries 19 and 20). Grinding has probably brought a pressing intimacy between the reactant molecules and allows them to react in an efficient manner akin to the observation made by Lamaty et al. [12g], in which mechanochemical activation for effective CN bond formation has been well demonstrated. The present findings may serve as a better alternative to the synthesis of β-amino alcohols using nonconventional techniques [25].
Regioselectivity was studied by the reaction of styrene epoxide with various aromatic amines and it has been noticed that electronic factors dominated and reaction proceeds with attack on benzylic carbon to deliver the single regiomers as shown in Scheme 1.
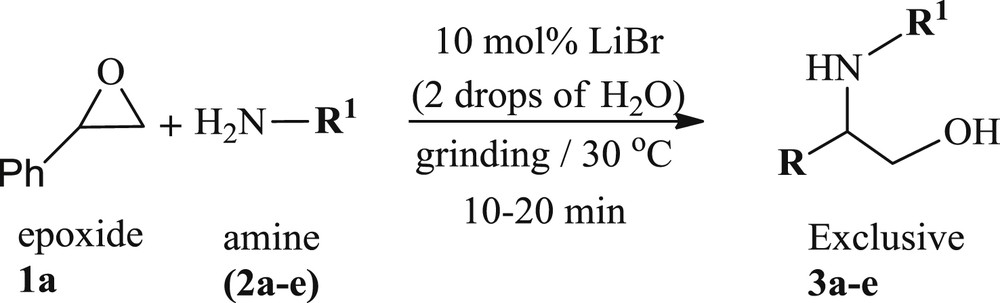
Regioselectivity in case of styrene epoxide.
But, in the case of benzotriazolated epoxides 1d,e both aliphatic and aromatic amines attacked exclusively at the less hindered carbon of an epoxide ring to afford the single region isomers (Scheme 2). Presumably, steric factor alone has dominated the regioselectivity in the case of benzotriazole glycidyl ethers and, therefore, single region isomers have been obtained exclusively. Furthermore, the formation of the single region isomers 3r-ad has also been confirmed by 1H NMR and mass spectroscopy. The fragmentation pattern obtained by ESI-MS [m/z (relative intensity)] of the representative compound 3t has been shown in Scheme 3.

Regioselectivity in case of benzotriazole glycidyl ether.
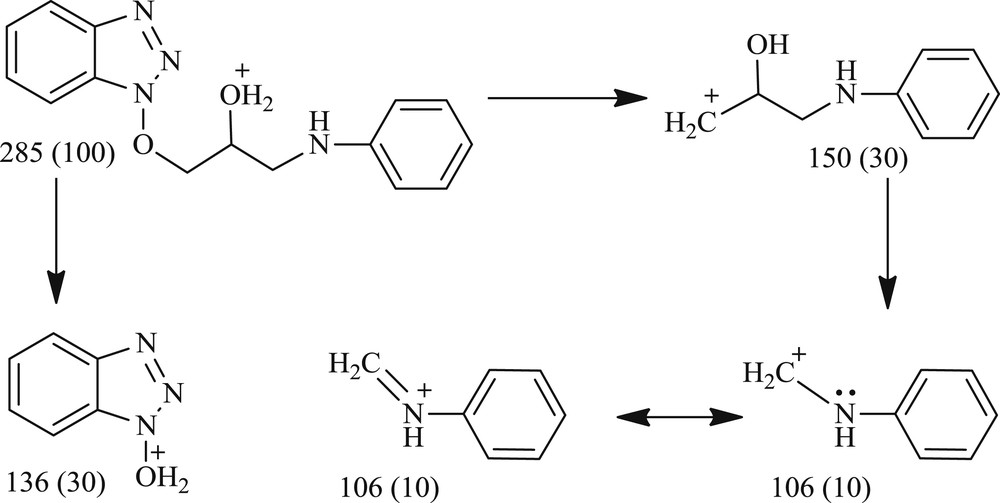
Fragmentation pattern of 3t [ESI-MS m/z (relative intensity)].
Finally, a plausible mechanism for the LiBr-assisted aminolysis has been depicted in Scheme 4. Li+(aq) has been supposed to coordinate with the oxygen atom of the epoxide 1, which facilitates the nucleophilic attack of amine 2 that finally mediates through the transition state to deliver target β-amino alcohols.

Proposed mechanism for ring opening of epoxide.
3 Conclusion
A simple and efficient route for the synthesis of β-amino alcohols via regioselective ring opening of epoxides has been developed. Furthermore, the developed methodology has successfully been applied for the synthesis of diverse benzotriazole nucleated analogs of β-amino alcohols. The newly synthesized compounds may act as potential drug candidates particularly in the field of β-adrenergic receptor blockers. Operational simplicity, complete regioselectivity, and ambient temperature with special emphasis on aqueous mortar–pestle grinding makes this protocol an attractive alternative route for the regioselective synthesis of β-amino alcohols.
4 Experimental section
4.1 General information
All of the chemicals used in the experiments were purchased from Sigma–Aldrich, Himedia, and SDFCL and were used as received without any further purification. The progress of the reaction was checked by thin-layer chromatography (TLC) using silica gel-G. Purification of crude products was carried through column/flash chromatography using 60–120 mesh size silica gel. Melting points were recorded in open capillaries using the ANALAB melting point apparatus and are uncorrected. The IR spectra were recorded as KBr discs or neat using Perkin–Elmer FT-IR spectrometer. 1H (400 MHz) and 13C (101, 126 MHz) NMR spectra were recorded as CDCl3/DMSO-d6 solutions with tetramethylsilane as an internal standard using Bruker advance III HD spectrometer. Mass analysis was carried out using Nexera UHPLC @ 130 Mpa with an SIL-30AC Nexera autosampler coupled with LC-MS 8030 tandem mass spectrometer (Shimadzu Corporation Tokyo, Japan). High-resolution mass spectral data were obtained from Agilent 6540 UHD Q-ToF mass spectrometer coupled with LC system.
4.2 General experimental procedure for the regioselective synthesis of β-amino alcohols 3
A 1:1.1 equiv of epoxide 1 and amine 2 (in case of isopropyl and tertiary butylamine 2.5 equiv is required) and LiBr (10 mol %) in two drops of water were subjected to aqueous mortar–pestle grinding for specified time (Table 2). After completion of the reaction (TLC), 2 mL of distilled water was added to the reaction mixture and product 3 was extracted with ethyl acetate, washed with brine (1 mL), and dried over anhydrous Na2SO4. The excess solvent was removed and crude products were subjected to column/flash chromatography using EtOAc/n-hexane to get pure products, 3a-ad in good to excellent yield. Physical data of representative compounds are given below.
4.2.1 Compound 3t
1-((1H-Benzo[d][1,2,3]triazol-1-yl)oxy)-3-(phenylamino)propan-2-ol (Table 2, entry 20): light brown solid (270 mg, 95%), mp 65–67 °C. TLC Rf = 0.45 (1:1, hexane/ethyl acetate). IR (KBr, νmax/cm−1): 3485 (OH), 3255 (NH), 1265 (NH), 1066 (CO), cm−1. 1H NMR (400 MHz, CDCl3): δ = 8.00 (d, J = 8.4 Hz, 1H), 7.64 (t, J = 8.5 Hz, 1H), 7.53 (dd, J = 14.5, 7.4 Hz, 1H), 7.45–7.35 (m, 1H), 7.18 (t, J = 7.9 Hz, 2H), 6.75 (t, J = 7.3 Hz, 1H), 6.67 (d, J = 7.7 Hz, 2H), 4.67–4.63 (m, 1H), 4.62–4.56 (m, 1H), 4.41–4.34 (m, 1H), 3.46 (dd, J = 13.2, 4.4 Hz, 1H), 3.33 (dd, J = 13.2, 7.0 Hz, 1H) ppm; 13C NMR (101 MHz, CDCl3): δ = 147.89, 143.58, 129.51, 128.56, 128.48, 127.27, 125.07, 120.41, 118.48, 113.51, 108.86, 82.63, 67.87, 46.36 ppm; ESI-MS m/z (relative intensity): 285.10 [M+H]+ (100), 150 (10), 136 (30), 106 (10); ESI-HRMS calcd for C15H16N4O2: [M+H]+ 285.1346, found 285.1345; Anal. calcd for C15H16N4O2: C, 63.37; H, 5.67; N, 19.71; found: C, 63.40; H, 5.65; N, 19.69.
4.2.2 Compound 3ad
1-((6-Chloro-1H-benzo[d][1,2,3]triazol-1-yl)oxy)-3-(isopropylamino)propan-2-ol (Table 2, entry 30): white solid (276 mg, 97%), mp 88–90 °C. TLC Rf = 0.25 (1:1 hexane/ethyl acetate). IR (KBr, νmax/cm−1): 3480 (OH), 3260 (NH), 1260 (OH), 1055 (CO). 1H NMR (400 MHz, CDCl3): δ = 7.92 (d, J = 8.9 Hz, 1H), 7.70 (d, J = 1.6 Hz, 1H), 7.34 (dd, J = 8.9, 1.7 Hz, 1H), 4.58 (dd, J = 10.5, 3.5 Hz, 1H), 4.49 (dd, J = 10.4, 6.8 Hz, 1H), 4.09 (ddd, J = 11.8, 7.3, 3.7 Hz, 1H), 2.94 (dd, J = 12.2, 3.8 Hz, 1H), 2.89–2.84 (m, 1H), 2.75 (dd, J = 12.2, 8.4 Hz, 1H), 1.11 (d, J = 6.3 Hz, 6H) ppm; 13C NMR (101 MHz, DMSO-d6): δ = 141.45, 133.90, 133.44, 130.82, 125.92, 121.35, 109.45, 82.01, 67.14, 50.81, 25.39 ppm; ESI-MS m/z (relative intensity): 285.10 [M+H]+ (100), 170 (30), 116 (20); ESI-HRMS calcd for C12H17ClN4O2: [M+H]+ 285.1113, found 285.1111; Anal. Calcd for C12H17ClN4O2: C, 50.62; H, 6.02; N, 19.68; found: C, 50.65; H, 6.04; N, 19.71.
Acknowledgments
The authors sincerely thank UGC, New Delhi, for financial support through F. No. 39-788/2010 (SR) and IIIM Jammu for providing spectra and microanalyses.
The authors also thankful to Prof Sanjeev Jain, Hon'able Vice Chancellor, SMVD University, and to Prof. V. Verma, Head, DoBT, for their kind support to provide the necessary facilities.