1 Introduction
Naproxen [(+)-2-(6-methoxy-2-naphthyl)propionic acid] is an analgesic, anti-inflammatory, and antipyretic drug used in the treatment of rheumatoid arthritis, osteoarthritis, gout, nonrheumatic inflammation, and migraine. In some cases, it is also effective in reducing pain of orthopedic surgery, muscle cramps, and muscle stiffness [1]. Naproxen should be given with caution in elderly patients with kidney problems and in patients with inflammatory disease of the upper or lower channel of ear duct, hemophilia, platelet coagulation dysfunction, and gastrointestinal bleeding [1]. Different procedures have been developed for the determination of naproxen. They include capillary electrophoresis [2], spectrophotometric [3] and fluorometric determination [4–6], spectrofluorometry [7,8], second-derivative synchronous fluorescence spectroscopy [9], synchronous luminescence spectrometry [10], chemiluminescence [11,12], phosphorescence [13,14], high-performance liquid chromatography [15–19], and electrochemical techniques [20–25]. Among these methods, electrochemical techniques have received a lot of attention, as they are simple, economical, and precise. Because electrochemical measurement of pharmaceutical and biological compounds was not possible with conventional electrode because of overvoltage, electrodes were modified with nanoparticles. The nanostructure materials have different and important physical and chemical properties because of their nanoscale. Due to these features, nanomaterials can be used as a tool to develop and improve analytical processes. In this study, activated carbon nanoparticles (ACNPs) were used as a modifier. Activated carbon has a crystalline form, which is a good absorbent substance because it has a lot of pores in its internal structure [26]. This material is produced by the pyrolysis of organic materials and carbonaceous sources such as coal, peat, wood, sawdust, and nutshells, and its applications mainly depend on the raw material and the production process [27,28]. High internal surface area, high porosity, ability to absorb gases, and chemical liquids are unique features of activated carbon, which would render it as an excellent absorbent material used in the carbon electrode structure [29]. Therefore, using electrochemical methods by electrode modified with ACNPs, in addition to improving the surface of electrode for oxidation of drug and reducing the overvoltage, increases the sensitivity, accuracy, and selectivity of electrode. Determination of naproxen is the main aim of this study. On the basis of the conducted research, the use of a carbon paste electrode (CPE) modified with ACNPs has not been reported for the measurement of naproxen until now. Therefore, first, a modified CPE with ACNPs (ACNP/CPE) was made, and all parameters that can have effects on the current of naproxen (including instrumental and chemical parameters) were optimized; then, the naproxen in pharmaceutical and physiological samples is measured by using the prepared electrode. Measurement of naproxen in the presence of acetaminophen is another goal of this study. Therefore, finally, simultaneous measurement of acetaminophen and naproxen was done with modified electrode.
2 Experimental section
2.1 Chemicals and reagents
All of the chemical materials used in this study are listed in Table 1. As can be seen from Table 1, all reagents had analytical grades and were purchased from Merck (Darmstadt, Germany). ACNPs with an average size of 20–30 nm (Fig. 1) were purchased from Degussa Company. All solutions were freshly prepared with deionized water. Graphite powder and paraffin oil (DC350, density = 0.88 g cm−3), as binding agents (both from Merck), were used for preparing the pastes. The buffer solutions were prepared from orthophosphoric acid and its salts, and its pH range was between 2.0 and 9.0.
List of chemical materials used in this study.
Chemical | Chemical formula | Purity (%) | Company |
Acetic acid | C2H4O2 | 100 | Merck |
Phosphoric acid | H3PO4 | 85 | Merck |
Sodium dihydrogen phosphate | NaH2PO4 | 99 | Merck |
Disodium hydrogen phosphate | Na2HPO4 | 99 | Merck |
Boric acid | H3BO3 | 99 | Merck |
Sodium hydroxide | NaOH | 99 | Merck |
Naproxen | CH3OC10H4CH(CH3)CO2H | 99 | Merck |
Paraffin oil | 100 | Merck | |
Graphite powder | C | 100 | Merck |
ACNPs | C | 99 | Degussa |
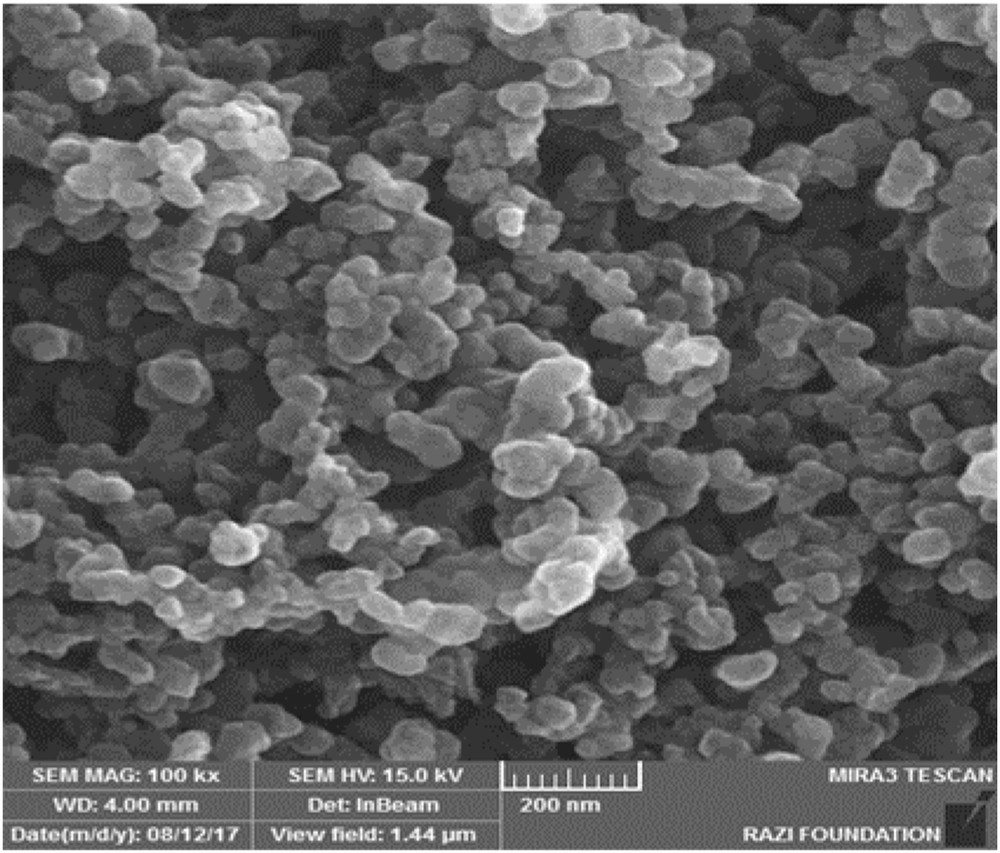
Scanning electron microscopy image of ACNPs.
2.2 Apparatus
In this study, an Autolab potentiostat PGSTAT 30 (Eco Chemie B.V., Netherlands) was used for electrochemical measurements including cyclic voltammetry (CV), differential pulse voltammetry (DPV), and electrochemical impedance spectroscopy (EIS), which contains a cell with three electrodes in the sample solution. These three electrodes are modified or unmodified CPE as working electrode, Ag/AgCl (3.0 M KCl) as reference electrode, and platinum wire as counter electrode. EIS experiments were obtained in the Fe2+/Fe3+ solution (0.1 M KCl solution containing 10 mM K3Fe(CN)6/K4Fe(CN)6), as the redox probe, at the open-circuit potential and over the frequency range of 0.01 Hz–100 kHz. Phosphate buffers with various pH values were made using Metrohome 827 pH meter. Analytical digital scale Skaltek SBA31 (d = 0.0001 g) was used to weigh chemicals, and Excel software was used to record the voltammogram curves and calculations.
2.3 CPE preparation
CPE, formed from the mixture of graphite powder with nonconductive organic compounds and insoluble materials in water, has a renewable surface, low price, and low current field. The composition of carbon paste is highly effective in electrode activity. For modification of carbon paste with ACNPs, first, 0.5 g of graphite powder was grinded in an agate mortar for 20 min. Then, 0.005 g of ACNPs were added to the graphite powder and after grinding for 20 min, about seven to eight drops of paraffin oil were added to it. The obtained homogeneous paste was compacted into an insulin syringe with a cross-section of 1.34 mm, and to connect the electrical device and drug solution in electrochemical measurements, a copper wire was imported into the carbon paste from the end of the syringe and was prepared to work with the electrochemical device.
3 Results and discussion
3.1 Definition of the appropriate amount of ACNPs for modification of electrode
Because ACNPs can affect the response of the electrode relative to naproxen, its amount was changed from 0.001 to −0.007 g, and CV and DPV of buffer solution, containing 10 μM of naproxen, were recorded. The results illustrated that the maximum current was obtained for the electrode with 0.005 g ACNPs and the current of electrodes containing 0.005, 0.006, and 0.007 g ACNPs is similar to 0.005 g ACNPs. Therefore, the electrode with 0.005 g ACNPs was chosen in the following experiments.
3.2 Electrochemical behavior of naproxen
To evaluate the efficacy and electrode resistance of modified and unmodified electrodes, cyclic voltammograms of these electrodes in the buffer solution without naproxen and with naproxen were investigated, and the results are shown in Fig. 2. The results show that modified electrode with drug and unmodified electrode without drug have maximum and minimum current heights, respectively. Fig. 2 curve c shows that the electrochemical response of naproxen on the surface of CPE has appeared in the potential of 0.936 V with Ipa = 0.45 μA, whereas for ACNP/CPE (Fig. 2, curve d), electrochemical signal of naproxen is observed in the potential of 0.932 V with Ipa = 1.7 μA. The small changes observed in peak potential indicate that ACNPs exhibit a slight catalytic effect. The oxidation current of naproxen on the ACNP/CPE surface is 4.5 times larger than on CPE. The reason for the observed high current in ACNP/CPE may be attributed to the characteristics of ACNPs such as high specific surface area and unique conductivity.
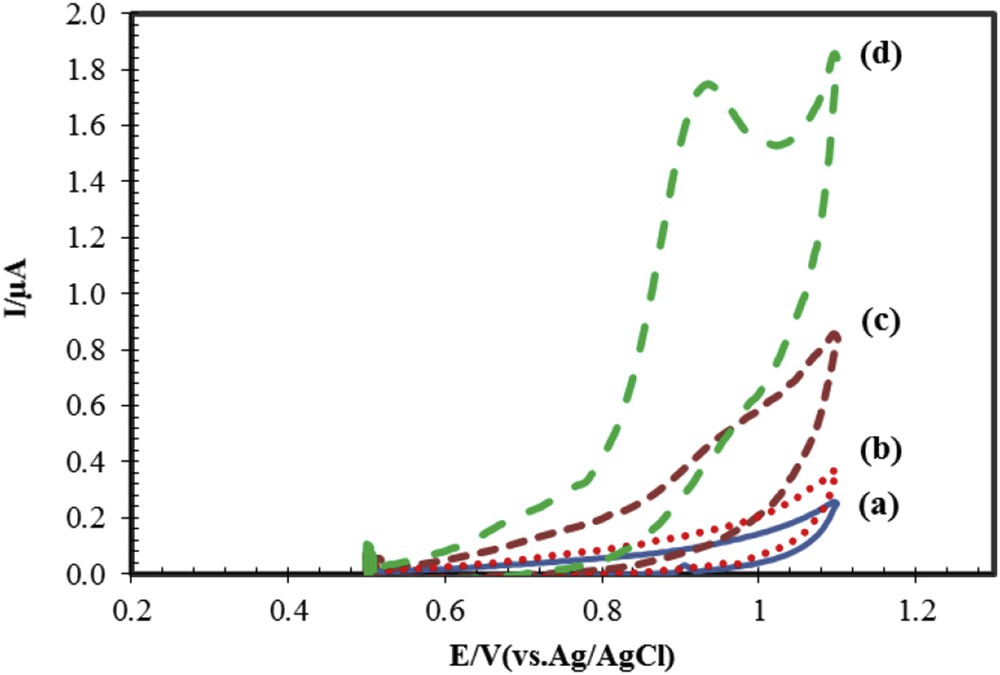
Cyclic voltammograms for modified and unmodified electrodes. (a and b) Bare and modified electrodes in buffer solution. (c and d) Bare and modified electrodes in buffer solution with naproxen. Conditions: 50 μM naproxen, buffer phosphate 0.1 M at pH 6.
3.3 Investigation of electrode with EIS and CV methods
Impedance is an electrochemical technique, which investigates all the reactions of an electrode surface that happen in the frequency range and different potentials. In this study, to determine the resistance of unmodified and modified electrodes by ACNPs in the process of drug oxidation, the Nyquist plots of these electrodes were compared in Fe(CN)33−/4− solution. According to the results (Fig. 3), modified electrode by ACNPs had lower resistance and better electron transfer rate with respect to the unmodified electrode. This illustrates that ACNPs have excellent electrical conductivity and let the electron transfer easier.
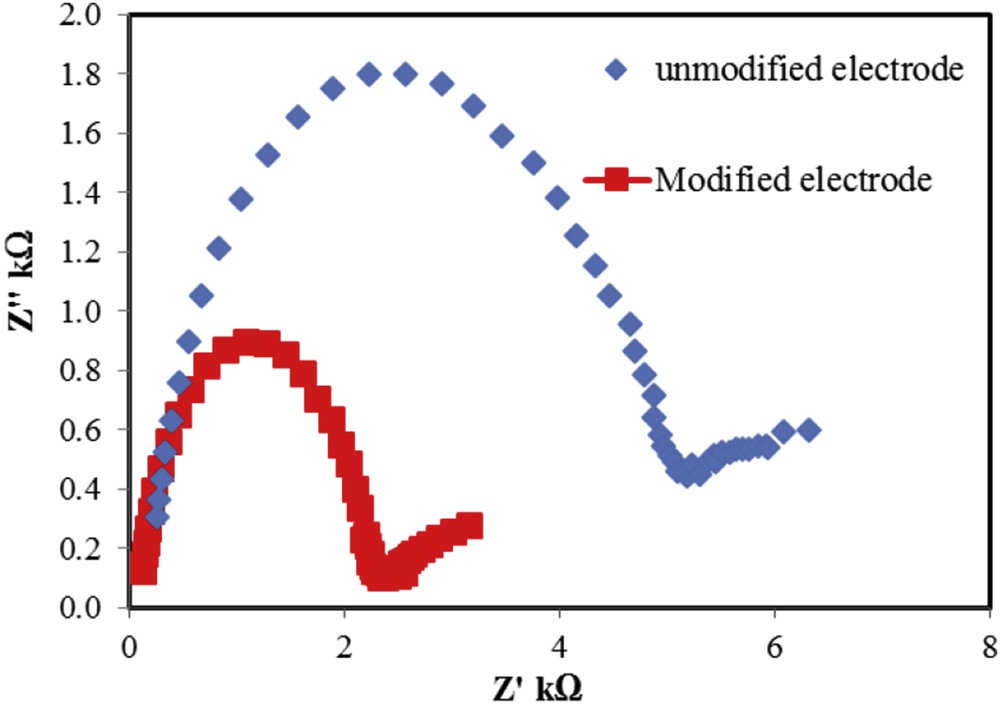
Nyquist plot of unmodified and modified electrodes by ACNPs in Fe2+/Fe3+ solution.
To compare the active surface area of CPE and ACNP/CPE, the real surface area of each electrode was obtained by using the Randles–Sevcik equation (Eq. 1).
(1) |
For this purpose, cyclic voltammograms were drawn for each of the electrodes in a 0.1 M KCl solution containing 1 mM of K3Fe (CN)6 at different scan rates. In Eq. 1, Ipa, n, A, D, ν, and C0 are anodic peak current, electron transfer number, surface area of electrode, diffusion coefficient, scan rate, and concentration of K3Fe(CN)6, respectively, where, for K3Fe(CN)6, n is equal to 1 and D is equal to 7.6 × 10−6 cm2 s−1. Therefore, using the slope of Ipa–ν1/2 curve and the values of n and D, the surface areas of CPE and ACNP/CPE obtained were 0.039 and 0.121 cm2, respectively. The results indicate that the surface area of ACNP/CPE is 3.1 times larger than that of CPE. The reason for increasing the surface area of ACNP/CPE can be attributed to the high specific surface area of the ACNPs.
3.4 Effect of the potential scan rate on the behavior of naproxen with CV method
To study the kinetic parameters including the determination of absorption or leakage of the process of drug oxidation on the surface of the CPE modified with ACNPs, the effect of the potential scan rate on the behavior of naproxen was investigated. In this way, cyclic voltammogram for drug was examined in the scan rate range of 30–130 mV, and the results are shown in Fig. 4a.
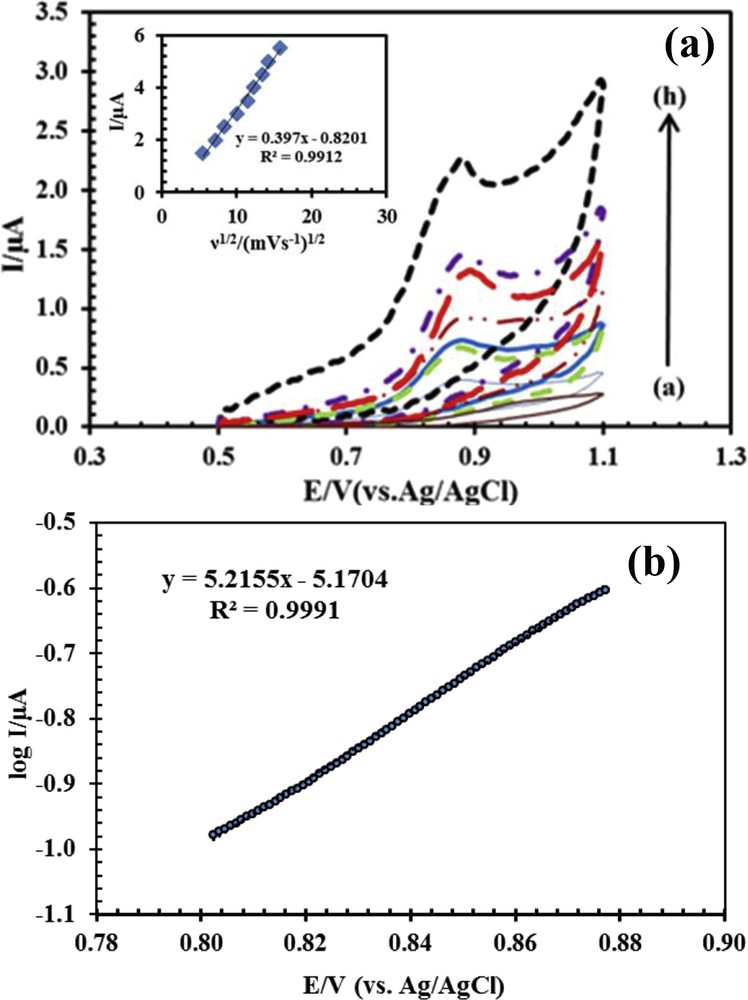
(a) Cyclic voltammogram for 50 μM naproxen with ACNP/CPE in 0.1 M phosphate buffer solution at pH 6, (a–h) 30, 50, 80, 100, 110, 115, 120, and 130 mV s−1; inset: variation of Ip versus square root of scan rate (ν1/2). (b) Tafel curve of log current versus potential from the rising section of CV with a scan rate of 50 mV s−1 obtained for 50 μM naproxen in 0.1 M phosphate buffer solution at pH 6.
The linearity of the relationship between Ip and ν1/2 shows that the oxidation of naproxen on the surface of a modified electrode was controlled by mass transfer and the species penetrates into the electrode for the oxidation. Therefore, the electro-oxidation of naproxen on the surface of ACNP/CPE was associated with a fast electron transfer kinetic [30].
Tafel curve, which is used to obtain electron transfer coefficient (α), was obtained from the CV plot with a scan rate of 50 mV s−1. The data of the rising section of CV identified as Tafel area were used for drawing Fig. 4b. The slope of the obtained Tafel curve is equal to (1 − α)nF/RT. By substituting the numerical values of n, F, R, and T in the slope of the Tafel curve, the amount of α for the oxidation of naproxen obtained was 0.85.
3.5 Effect of pH
Oxidation of drug profoundly depends on the pH of the sample solution. To determine pH optimum, the solutions of buffer phosphate were prepared in the pH range of 2.0–9.0. The voltammogram curves for determination of optimum pH with DPV method for CPE were obtained, and the results are presented in Fig. 5. The curves of peak current versus pH and peak potential versus pH are shown in Fig. 5band c, respectively. The results of Fig. 5b show that the maximum current has been obtained for pH 6.0. Therefore, phosphate buffer with pH 6 was selected as the optimum pH for subsequent experiments. As shown in Fig. 5c, the peak potential has a linear relationship with pH. It shows that the proton participates in the electrochemical reactions on the surface of the electrode and the change in pH causes the change in potential oxidation. In other words, the reaction was done in more positive potential by decreasing the pH and increasing the H+ ion concentration. The slope of linear equation in Fig. 5c is equal to 0.0563. This means that the number of protons and electrons participating in the oxidation reaction of naproxen is equal (Scheme 1) [24].
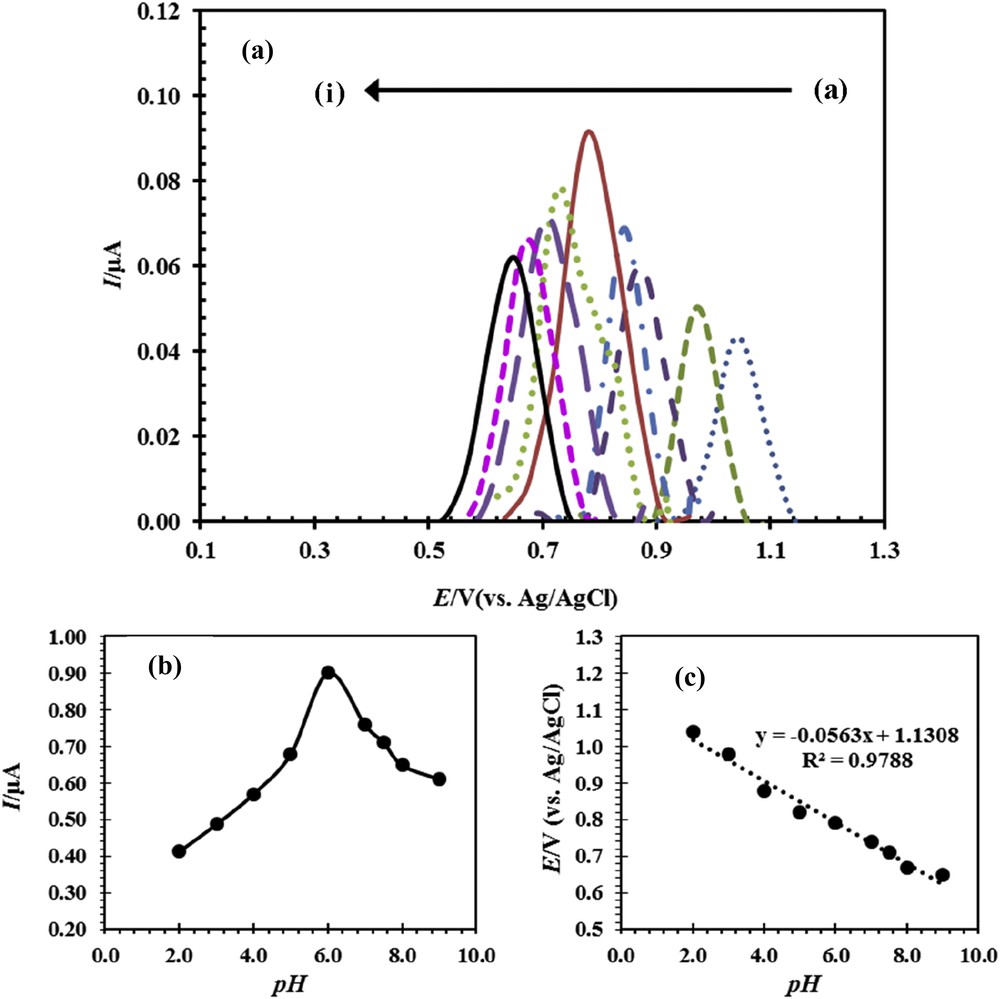
(a) Differential pulse voltammogram curve for ACNP/CPE in 0.1 M phosphate buffer solution in the pH range 2–9, containing 50 μM naproxen, (a–i) 2, 3, 4, 5, 6, 7, 7.5, 8, and 9. (b) The effect of pH on the peak potential. (c) The effect of pH on the peak current of naproxen oxidation.

Proposed mechanism for the oxidation of naproxen.
3.6 Calibration curve
To evaluate the quantitative and the detection limit in electrochemical methods, DPV method was used because it has higher sensitivity than CV. Because DPV parameters of the pulse height and the pulse width can affect the peak sensitivity and morphology, these parameters have been investigated, and the results show that the values 40 mV of the pulse height and 50 ms of the pulse width cause the most suitable DPV signal. The curves are obtained by different concentrations of naproxen, and the results are shown in Fig. 6. These results indicate that the current changes versus the concentration of naproxen were linear in the range of 0.1–120 μM, and the detection limit was 0.0234 μM.
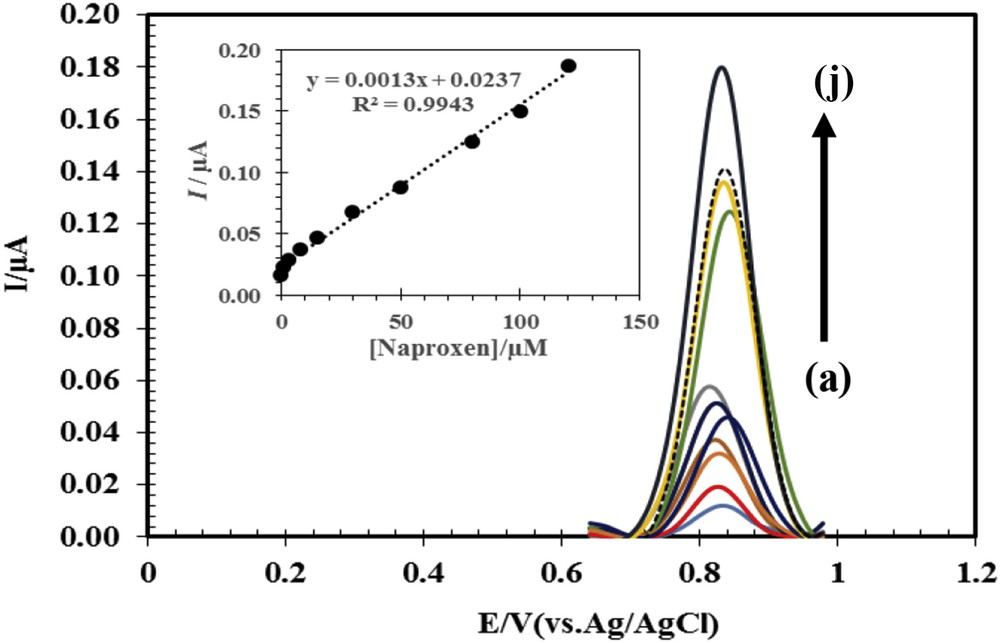
Differential pulse voltammogram for different concentrations of naproxen with ACNP/CPE in 0.1 M phosphate buffer solution at pH 6; (a–j) 0.1, 1.0, 3.0, 8.0, 15.0, 30.0, 50.0, 80.0, 100.0, and 120.0 μM. Inset represents current changes versus different concentrations of naproxen.
Linear dynamic range, detection limit, and sensitivity obtained for ACNP/CPE in this study are compared with some electrochemical methods reported for determination of naproxen in Table 2. According to Table 2, except DyNw/CPE, linear dynamic range and detection limit of ACNP/CPE are better than all electrodes presented in this table.
Comparison of the proposed electrode in this study with some other previously reported electrodes.
Electrode type | Determination method | Dynamic range | Detection limit | Sensitivity | Ref. |
MWCNT/GCEsa | Amperometric | 10–100 mM | 0.6 mM | 0.394 μA μM−1 | [22] |
MWCNT/msCYP1A2-SPEb | Amperometric | 9–300 mM | 16.00 μM | 0.54 μA/mM mm | [21] |
MWCNT-Gr-IL|CCEc | DPV | 0.8–100 μM | 0.123 μM | 0.3533 μA μM−1 | [24] |
DyNW/CPEd | Square wave voltammetry | 0.001–500 μM | 0.0005 μM | 0.387 μA μM−1 | [23] |
Platinum electrode | DPV | 1–25 μg mL−1 | 0.24 μg mL−1 | 0.1142 μA mL μg−1 | [20] |
ZnO/MWCNTs/CPEe | Square wave voltammetry | 1.0–200 μM | 0.23 μM | 0.256 μA μM−1 | [25] |
ACNP/CPEf | DPV | 0.1–120 μM | 0.0234 μM | 0.0013 μA μM−1 | This work |
a Multiwalled carbon nanotubes (MWCNTs) modified glassy carbon electrode (GCE).
b MWCNTs and microsomal cytochrome P4501A2 (msCYP1A2) on a graphite screen-printed electrode (SPE).
c MWCNT–graphene hybrid combined with a hydrophobic ionic liquid (IL) as a binder to modify carbon ceramic electrode (CCE).
d Dysprosium nanowire modified CPE.
e ZnO nanoparticles and MWCNTs modified CPE.
f Modified CPEs with activate carbon nanoparticles.
3.7 Effect of interferences
To evaluate the selectivity of the method, the effect of different species on anodic peak current of naproxen was examined, and the results are shown in Table 3. In these experiments, the used species were similar to the species in the physiological samples. Each species was first added to have the same concentration as that of naproxen, and then another addition causes the concentration of interfering to be increased. The tolerance limit was taken as the maximum concentration of the foreign substances, which caused an approximately ±5% relative error in determination of current. As can be seen in Table 3, the concentration of interference species is very high with respect to naproxen concentration. The results indicated that selectivity of the modified electrode for determination of naproxen is very good and can be easily used for real samples.
The effect of interferences on 1 × 10−5 mol L−1 naproxen measurement with ACNP/CPE in 0.1 mol L−1 phosphate buffer at pH 6.
Species | The molar ratio of interference species to the concentration of naproxen |
Ascorbic acid | 800 |
Glycine | 500 |
Citric acid | 1000 |
Oxalate | 800 |
Na+, K+, Mg2+, Ca2+ | 900 |
NO3−, CO32−, Cl− | 850 |
Fructose, sucrose, glucose | 400 |
Aspirin | 200 |
3.8 Reproducibility, repeatability, and stability of the modified electrode
The reproducibility of the preparation procedure of the modified CPE was estimated by determination of 10 μM naproxen solution using six independently prepared modified electrodes based on the same fabrication procedure, and the obtained relative standard deviation (RSD) values were 1.2%. Reproducibility in the preparation and determination procedure was affirmed by the obtained low RSD. The RSD of the peak currents of 10 μM naproxen for 15 repeated determinations with the same modified electrode was 0.26%. This indicates that repeatability of modified electrode for determination of naproxen is desirable. Stability of the modified CPE was examined by keeping the electrode in the buffer solution with pH 6.0 for 1, 2, and 3 weeks; after this time, the DPV of the electrode was compared with DPV of the electrode before immersion. The results indicated that even after 4 weeks of immersion, change in the peak current of naproxen was less than 5%, which indicated that the stability of the electrode was very good.
3.9 Determination of naproxen in pharmaceutical samples and human blood serum
To evaluate the efficacy of applied method in real samples, the content of naproxen in tablet (250 and 500 mg) was obtained by the standard addition method. The results are presented in Table 4. To investigate the effect of tissue of physiological samples on naproxen measurement, the blood serum sample was prepared, and after addition of a known concentration of standard solution of naproxen, the amount of the percentage recovery was obtained by the DPV method (Table 5). The results in Tables 4 and 5 show that their complicated compound of tablet, blood serum, and real samples did not have any effect on the efficacy of naproxen.
The results of naproxen measurement in pharmaceutical samples.
Concentration of naproxen labeled | Naproxen added (μM) | Naproxen found (μM) | Recovery (%) |
250 mg | – | 4.98 ± 0.9 | 99.8 |
30 | 34.88 ± 1.2 | 99.7 | |
60 | 64.83 ± 1.3 | 99.7 | |
90 | 94.82 ± 1.4 | 99.8 | |
500 mg | – | 9.98 ± 1.1 | 99.8 |
20 | 29.95 ± 1.3 | 99.8 | |
40 | 49.84 ± 1.4 | 99.7 | |
60 | 69.79 ± 1.5 | 99.7 |
Naproxen measurement in human blood serum sample.
Human serum | Naproxen added (μM) | Naproxen found (μM) | Recovery (%) | RSD (%) |
Sample 1 | 20 | 19.8 ± 1.2 | 99.0 | 1.1 |
Sample 2 | 40 | 39.5 ± 1.3 | 98.8 | 1.3 |
Sample 3 | 60 | 59.2 ± 1.3 | 98.7 | 1.3 |
Sample 4 | 80 | 78.8 ± 1.4 | 98.5 | 1.4 |
Sample 4 | 100 | 98.7 ± 1.2 | 98.7 | 1.4 |
3.10 Determination of naproxen in the presence of acetaminophen
As mentioned in Section 1, one of the goals of this study was to determine naproxen in the presence of acetaminophen. For this purpose, in the first stage, the concentration of acetaminophen was kept 150 μM, and naproxen concentration was changed from 25 to 130 μM. In the second stage, the concentration of acetaminophen was changed from 35 to 190 μM, whereas that of naproxen was fixed in 50 μM. According to DPVs in Fig. 7, the peak current of acetaminophen remains constant when the concentration of naproxen is changed, and a linear relationship between the concentration and peak current of naproxen can be observed. Similar conclusions were obtained for the second stage. These results demonstrate that measuring these two drugs together by using the modified electrode is possible. Therefore, in the biological samples where these two drugs exist, this electrode may be useful for their simultaneous determination.
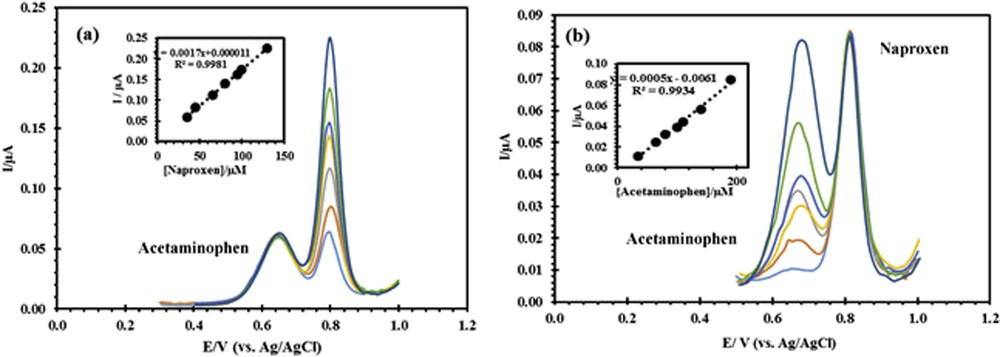
DPV of different concentrations of (a) naproxen (35, 45, 65, 80, 95, 100, and 130 μM) in the presence of 150 μM of acetaminophen and (b) acetaminophen (35, 65, 80, 100, 140, and 190 μM) in the presence of 50 μM of naproxen.
4 Conclusions
In this study, the electrochemical behavior of the modified CPE by ACNPs in the oxidation process of naproxen was investigated. Addition of ACNPs to CPE increased the peak current of naproxen up to 4.5 times due to its high electrical conductivity and high specific surface area. The detection limit for naproxen drug using the modified CPE was 0.0234 μM. The linear relationship between the peak current and the square root of potential showed that the oxidation of naproxen was controlled by mass transfer and the species penetration to the electrode for the oxidation. The selectivity of this method was achieved by investigating the effect of interferences. This method was demonstrated by pharmaceutical and real samples showing that it did not have any significant difference from the standard method. Moreover, this method was stable, available, fast, simple, economical, and precise.
Acknowledgments
The financial support of the research council of Payame Noor University of Isfahan is gratefully acknowledged.