1 Introduction
In recent years, efforts have been made to conserve water because of the growing demand for a limited resource. Water resource in general (surface, groundwater, etc.) is considered one of the most critical indicators for sustainable development in Mediterranean countries and especially those who have already felt the climate change effects. It is an important component for conservation and human health beside agriculture, industry and economic development. The world total population [1] is estimated to be up to 9.3 billion (33% more than was in 2011) and this will happen before 2050 while at the same time the needs for food are estimated to increase by 65% as indicated by Alexandratos and Bruinsma [2], at the same period. Also, the projection given by UN DESA [1] indicated that 6.3 billion citizens (50% higher) would have moved in urban areas affecting the metabolism of the cities [3]. These activities are related to the water consumption, raw material and other needs. In addition, water is a crucial component of local as well as national economies and is considered to be very important for developing and controlling jobs worldwide in all of the economy sectors. However, population growth, agricultural and industrial activities, as well as climate change effects put pressure on the global freshwater resource, as water stress today affects more than two billion citizens globally. Freshwater extraction has increased dramatically worldwide (about 1% per year since the 1980s), mainly because of the growing demand in developing countries. Also water scarcity either in developing or especially in not developing countries reduced the possibilities for further economic development or job position [4].
According to the United Nations Environment Program, 2.4 billion people nowadays (almost one in three) still have limited or no access to safe drinkable water. Water quality is crucial for domestic, agricultural and industrial purposes, and in developing countries with arid climates, groundwater is the main and only source like in South of Algeria.
It is well known that the quality of groundwater is strongly related to the different chemical substances and their concentration in the water, and for these reasons, assessment and monitoring of the physicochemical parameters such as pH, electrical conductivity, metals, fluorides, hardness and so forth are crucial. On the other hand, industrial and municipal waste has caused pollution in surface and ground waters. In many parts of the world, available water is nonpotable because of the high concentration of pollutants (such as metals, fluorides, etc.) [5,6].
Fluoride is considered to be a common element of groundwater. Natural sources are connected mainly to minerals rocks and volcanic activities. The use of phosphatic fertilizers in agricultural and other industrial activities such as clays in ceramics also contributes to the presence of fluorides in groundwater [7]. High fluoride concentrations (which are mainly associated with sodium bicarbonate water with relatively low concentrations of Mg and Ca) in groundwater may be responsible for bone diseases, mottling of teeth, thyroid, liver, and other organs, affecting the kids intelligence, male sterility, depression effects, neurologic manifestation, painful skin rashes and so forth [8–11]. World Health Organization suggests that the maximum limit of fluoride in potable waters must not exceed 1.5 mg/L [12]. In case fluorides are higher than 1.5 mg/L mottling of teeth may occur, whereas skeletal fluorosis may appear if fluoride concentration varies from 3 to 6 mg/L [12]. However, in several arid areas [7] governments have been forced to set strictly limits The concentration of fluoride in aquifers in northern Algerian Sahara often exceeds World Health Organization standards (>3 mg/L) and may related to teeth lesion [10].
Physicochemical processes have been widely studied for the removal of fluoride from water. Among these processes, electrocoagulation, coagulation, flocculation, filtration, chemical precipitation, adsorption, ion exchange and membrane technology were applied [11,13–15]. Also, among the best available techniques, sorption-based process includes fluoride adsorption on activated alumina and charcoal bone [11]. Moreover, the adsorption technique has already shown its potential in the treatment of inorganic pollutants (fluorine), toxic organic compounds, natural organic matter and volatile organic compounds [13–15]. Generally, adsorption phenomenon depends on the adsorbate and adsorbent nature. Physicochemical structures of the adsorbent material are crucial for determining the maximum adsorption capacity of the sorbent.
In this context, this article focuses on the application of clay, kalonite, for removal of fluoride ions from Saharan groundwater, located in Tindouf region (Algeria).
2 Materials and methods
2.1 Area description
Tindouf is the 37th of 48 Algerian districts. It is a Saharan city located in the southwest of Algeria (in the far west of the desert region Saoura, south of Hamada). It is located between 5° and 9° West of Greenwich line and between circle 26°–28° North of Equator, bordered to the north by Morocco border, northeast by the Bechar province, east by the Adrar province, south by Mauritania, and west by the nonautonomous territory of Western Sahara (Fig. 1). This province covers an area of 159,000 km2 with a total population of more than 60,000 inhabitants [16,17]. Tindouf climate is continental desert type, with the average lower temperature in January up to 5 °C and the highest in July up to 45 °C. Annual rainfall is very low and hardly exceeds 50 mm per year in the entire region. Evolution of evaporation, water infiltration and region flat topography can be defined as a water drainage system. Morphologically, Tindouf forms a flat and monotonous region (Hamada), except for some residual relief (−50 m elevation). It belongs to Western basin unit of the Northern Sahara. The river system is poorly developed with large dry wadis throughout the year [18,19]. The fluoride concentration in 56 water boreholes of Tindouf area varies between 0.16 and 3.31 mg/L. Water boreholes selected from the study area have been classified into four groups as low (0.1–0.5 mg/L) 6%, medium (0.5–1.5 mg/L) 20%, high (1.5–2 mg/L) 38% and very high (2–4 mg/L) 36% (Fig. 1).
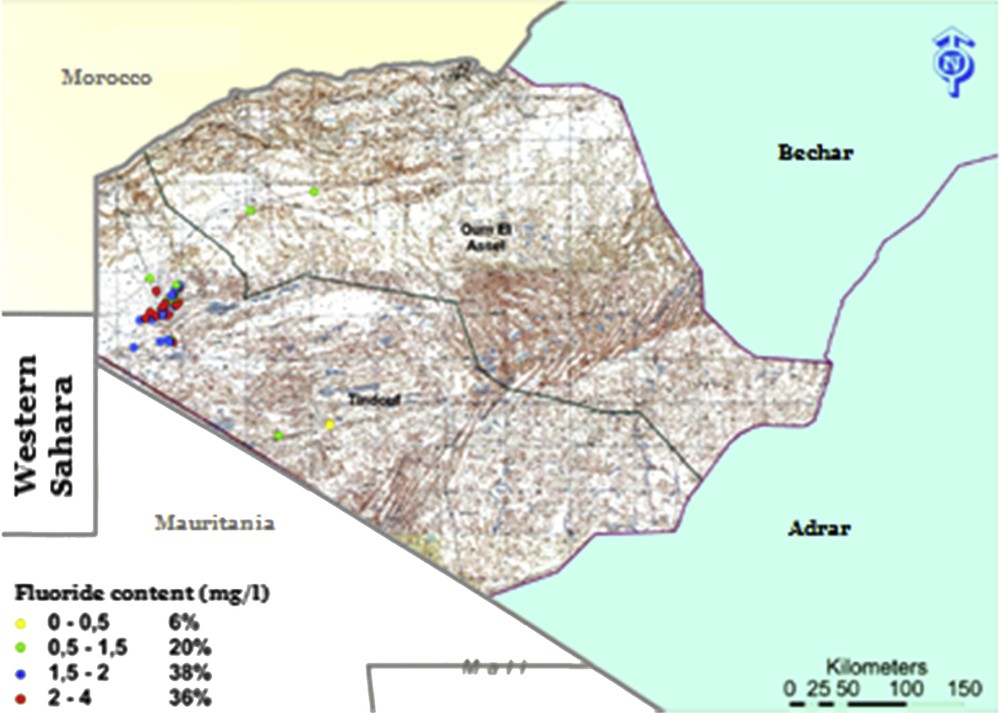
Fluoride ion distribution at Tindouf region.
2.2 Characterization of natural clay
In this study, natural clay in the form of white rocks was acquired from Tabelbala located in Bechar province of Algeria. This natural clay was used without any thermal or chemical treatment. The clay was crushed and sieved to articles with a diameter less than 2 μm. Then, the raw material was purified by sedimentation using distilled water and dried at 105 °C for 24 h after separation from water and impurities.
The physicochemical characteristics of the clay were performed by Fourier transform infrared (FTIR) spectroscopy using NICOLET 6700 IR spectrometer, X-ray fluorescence and X-ray diffraction (XRD) analyses using Philips (Magi XPRO) and PANalytical XPERT-PRO instruments, respectively. The point of zero charge (pHpzc) of adsorbent was determined with pH titration procedure. For this purpose, 50 cm3 of NaCl 0.01 M solution was put into flasks. The pH solution ranging from 2 to 9 in each flask was adjusted by addition of 0.1 M of HCl or 0.1 M of NaOH solution. Then, 0.15 g of clay was introduced in each flask and the final pH was determined after 48 h. The pHpzc is defined as the point where the curve of pHfinal versus pHinitial passes on the line pHfinal = pHinitial [20]. Cationic exchange capacity (CEC) was determined according to the method proposed by Kahr and Madsen [21].
2.3 Adsorption experiments
The adsorption isotherms at 28 ± 02 °C were obtained by introducing 100 mL of solution of different fluoride concentrations (5–100 mg/L) and with different doses (1–10 g/L) of local clay into well-closed flasks. Then, all of the solutions were shaken for 3 h, which was enough to reach equilibrium adsorption conditions. After shaking, the suspension was centrifuged, and the final fluoride concentration was measured using ionic chromatography apparatus (881 Compact IC pro) without any adjustment in pH values. The effect of pH on the adsorption process of fluoride was tested by varying the pH solution from 2 to 12 (using either NaOH or HCl). The amount of the adsorbed fluoride on natural clay was calculated according to Eq. (1):
(1) |
Two kinetic models, pseudo-first-order and pseudo-second-order models were investigated to study the kinetic data of fluoride ion removal.
The pseudo-first-order linear equation is given by Eq. (2):
(2) |
The pseudo-second-order kinetic model is considered to be the most suitable to describe the kinetic adsorption experiment. The linear form equation of this model is given by Eq. (3):
(3) |
The experimental data of equilibrium adsorption isotherms for fluoride ions into local clay adsorbent were modelled using the most frequently used isotherms, Freundlich and Langmuir. The Freundlich isotherm equation has the following form:
(4) |
The Langmuir model is the most widely used isotherm equation, which has the following form:
(5) |
(6) |
The thermodynamic data are given by
(7) |
(8) |
3 Results and discussion
3.1 Characteristics of natural clay
FTIR technique was used for the determination of functional groups present in the natural clay of Tabelbala (Fig. 2). Each link has a definite vibration frequency characteristic [22]. The valence vibration of the OH groups results in the presence of three absorption bands centred on the 3649 cm−1 frequency (external OH) and 3619 and 3690 cm−1 (internal OH). The hydroxyl groups may be distinguished by a doublet in 3619, 3649 and 3690 cm−1 (which is the pick of the kaolinite clay). The band corresponding to stretching vibrations of the SiO is between 1000 and 500 cm−1 and centred about 1034 cm−1. The band centred at 1648 cm−1 is attributed to deformation vibrations of HOH molecules of water [23]. The bands centred at 985, 836, 797, 674 and 508 cm−1 are assigned to the vibration deformation of the AlOHAl bonds, SiOAl/AlMgOH, cristobalite, SiOMg and MgOH, respectively [23]. The band centred at 915 cm−1 is not attributed solely to the connections of the deformation vibrations AlOHAl but is also attributed to the presence of kaolinite [24].
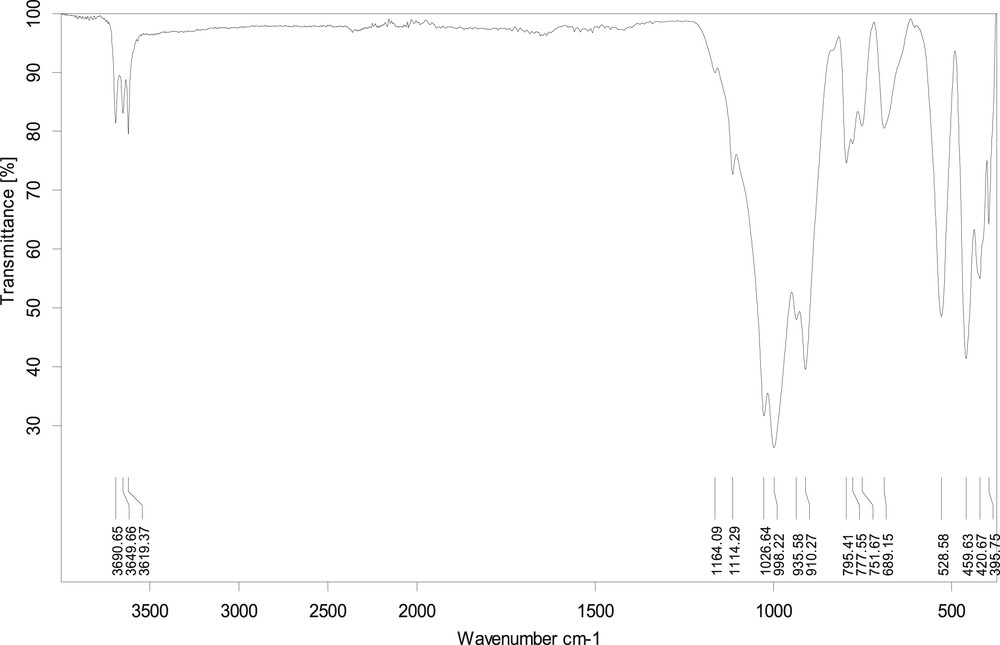
FTIR spectra of the clay.
The XRD is considered to be one of the most useful and common techniques that has been used in the mineralogical characterization (Table 1) of clay materials [25,26]. Fig. 3 shows the XRD pattern of the purified clay sample, consisting of kaolinite, quartz and a small amount of illite. For the kaolinite whose structure is type 1/1, the layer of kaolinite is formed of two layers (tetrahedral and octahedral). The peaks of this kaolinite were observed at 2θ = 12.39, 20.36, 23.11, 24.94 and 39.37, which correspond to the ratio of the peak intensities from basal diffraction to (0 0 1) (d = 0.714 nm), (1 1 0) (4.35 nm), (1 0 −1) (3.84 nm), (0 0 2) (3.566 nm) and (0 0 2) (d = 3.570 nm) [27,28].
Chemical composition of clay used in the experiment (wt %, from X-ray fluorescence data).
Chemical compound | SiO2 | Al2O3 | Fe2O3 | CaO | MgO | MnO | Na2O | K2O | P2O5 | TiO2 | SO3 | TOT |
(%) | 53.83 | 39.81 | 1.150 | 0.15 | 0.46 | 0.01 | 0.67 | 2.39 | 0.18 | 1.27 | <0.01 | 99.92 |
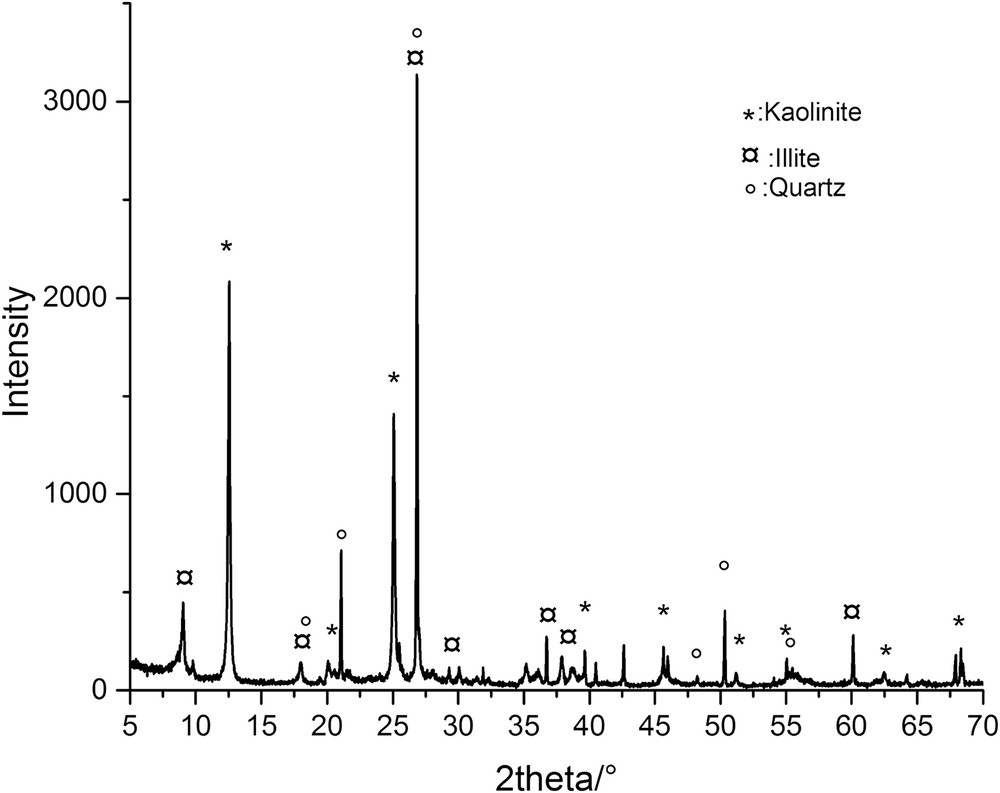
XRD spectra of the clay.
The pHpzc of Tabelbala natural clay is 6.4. The variation in the pH of solution (addition of H+ or OH− ions) affects the surface charges of sorbent materials. At pH < pHpzc the surface of adsorbent is positively charged and negatively charged at pH > pHpzc. According to Smolik and Harman [29] the pHpzc of mullite is 8, whereas that of the aluminosilicates of composition (Al2O3, SiO2) is 6–7.
The CEC of clay may be specified as the quantity of cations available for exchange (Ca2+, Mg2+, K+, Na+, H+ and Al3+) at a given pH. CEC of Tabelbala natural clay is 8 mequiv/100 g. According to Vasconcelos et al. [30], the CEC of kaolinite, illite and smectites varies from 3 to 15,10 to 40 and 80 to150, respectively, which are close to our findings because of the use of kaolinite.
3.2 Optimization of operating conditions for adsorption
Fig. 4 shows the fluoride removal as a function of adsorbent dose at a fixed initial concentration of 5 mg/L of fluoride, V = 100 mL, pH 5.8 and temperature 28 ± 02 °C. It was observed that the removal of fluoride increased from 46% to 90% and the residual amount decreased from 2.83 to 0.45 mg/g with increasing adsorbent dose to be 0.1–1 g, respectively. The comparison of the fluoride removal percentage of different adsorbents (with the same dose 10 g/L and the same initial concentration 5 mg/L) for fluoride adsorption is reported in Table 2. It clearly shows that Tabelbala kaolinite is the best adsorbent. The removal percentage is the same as the 20% La-chitosan [1] but higher than the bleaching powder (CaOCl2) [2], metal oxide (10% lanthanum) incorporated bentonite clay [3], activated charcoal [4] and tea leaves [5].
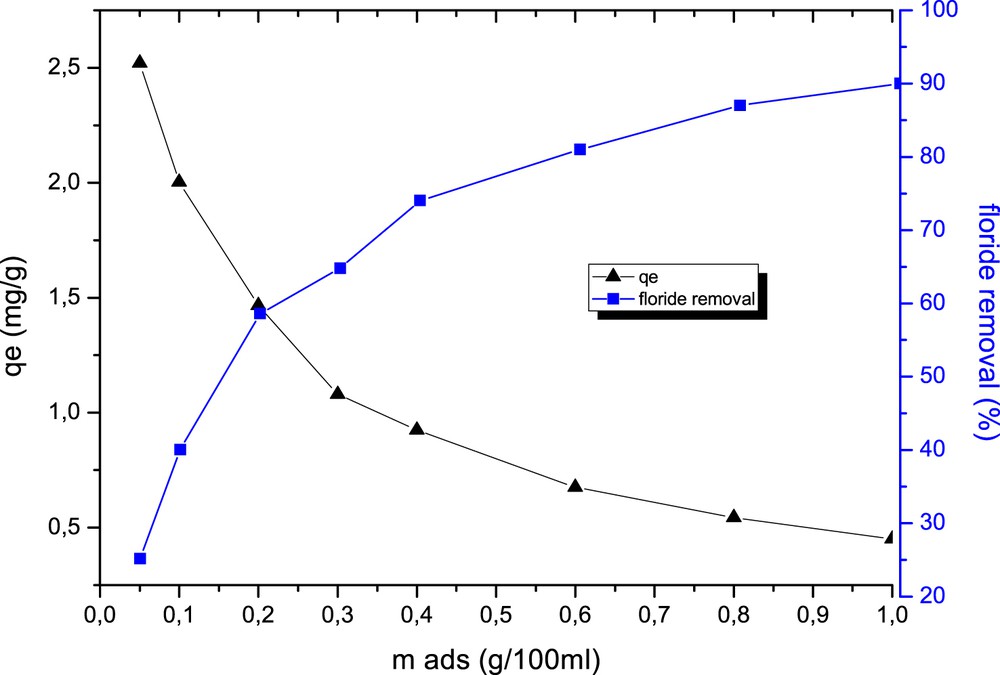
Variation in fluoride removal with clay dose (fluoride initial concentration, 5 mg/L; pH, 5.8; temperature, 28 ± 02 °C; contact time, 3 h).
Comparison of the fluoride removal percentage of different adsorbents for fluoride adsorption.
Adsorbent | R (%) | Reference |
20% La-chitosan | 90 | [31] |
Bleaching powder (CaOCl2) | 28 | [32] |
10% La-bentonite | 65 | [33] |
Modified immobilized activated alumina | 95 | [34] |
Activated charcoal | 84 | [34] |
Tea leaves | 85 | [35] |
Kaolinite of Tabelbala | 90 | This study |
The effect of solution pH on fluoride removal in clay (initial concentration 5 mg/L, adsorbent dose 10 g/L and temperature 28 ± 2 °C) is presented in Fig. 5. During these experiments, pH was adjusted between 2 and 12 using 0.1 N HCl and 0.1 N NaOH. The pHpzc of the natural clay is 6.4. At pH lower than pHpzc, the surface of the clay is positively charged that may improve the adsorption of fluoride ions. It was noticed that the adsorption capacity was relatively constant when pH increased from 4.5 to 6, leading to values of 0.442 and 0.448 mg/g, respectively. Between pH 4.5 and 6, the specific adsorption of fluoride on natural clay occurred because of the electrostatic interaction between the positively charged clay surface and negatively charged fluoride ions. At pH = 2, the adsorption capacity decreased considerably to 0.225 mg/g. In highly acidic pH, fluoride could exist preferably as the form of HF, and the adsorption of fluoride in natural clay could be reduced significantly. However, the adsorption capacity decreased sharply to 0.05 mg/g at pH 12. In highly alkaline solution (pH = 12), fluoride could exist preferably as NaF form due to the addition of NaOH into solution. At higher pH, more than 6.4 (pHpzc), the adsorption decreases as a result of the electrostatic repulsion between the negative surface charge of the clay and negative charge of fluoride ions. The competition between fluoride and hydroxide ions may be another phenomenon that reduced the removal of fluoride ions from water as indicated from Ali [36] and Nan et al. [37].
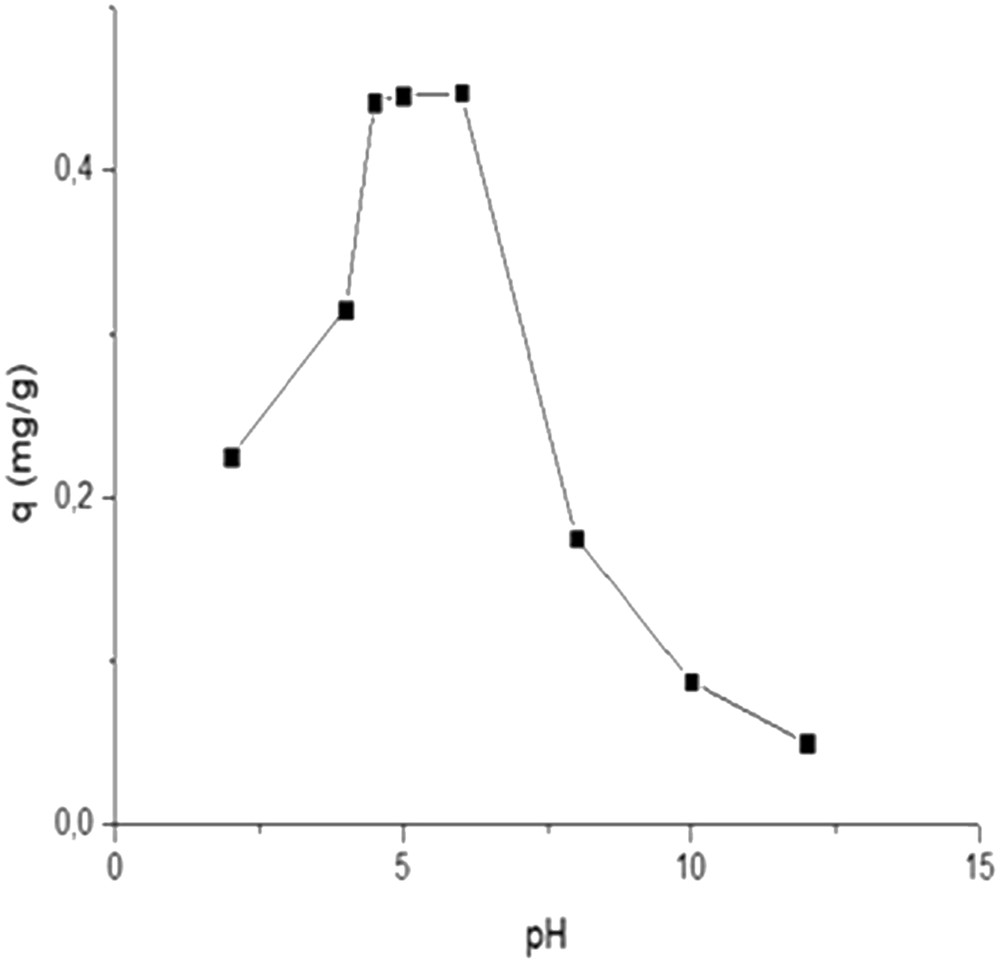
Effect of pH of fluoride adsorption on local clay (fluoride concentration, 5 mg/L; temperature, 28 ± 02 °C; contact time, 3 h).
3.3 Kinetic adsorption
The kinetic adsorption of fluoride ions in clay was performed at initial pH = 5.8 and initial concentration of 5 mg/L, with 10 g/L of natural clay. The adsorption study clearly implies the determination of the equilibrium time. The results in Fig. 6 indicated that the equilibrium time was 2 h. The fluoride adsorption capacity increases with increasing initial fluoride concentration. Such behaviour is due to the availability of fluoride ions in a solution at higher concentration.
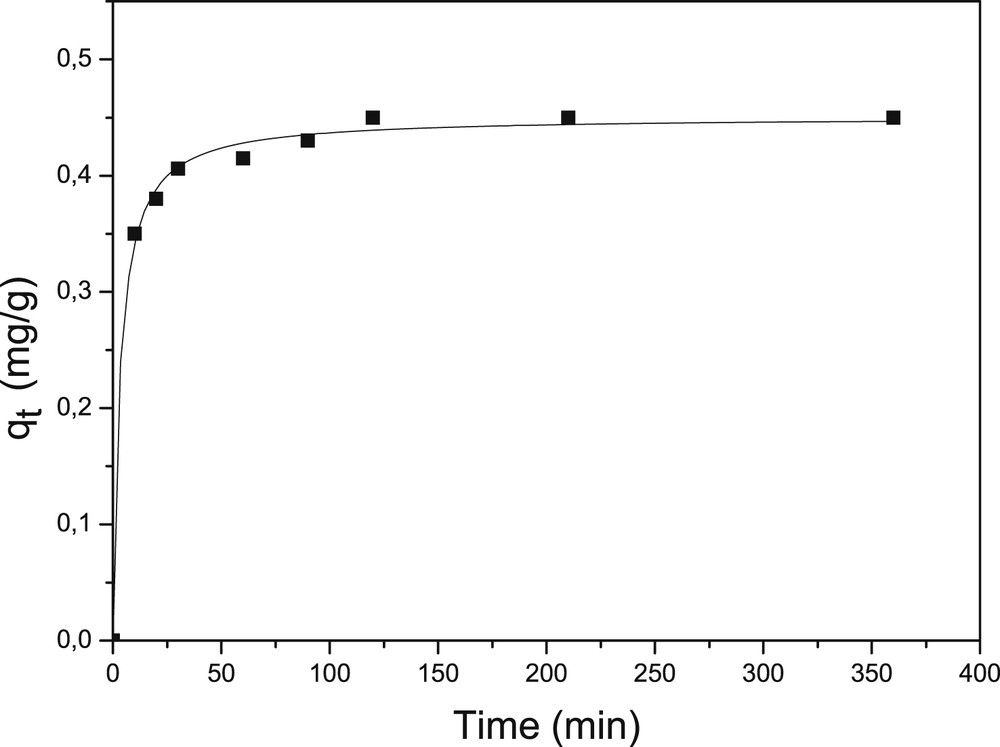
Effect of contact time of fluoride adsorption on local clay (fluoride concentration, 5 mg/L; pH, 5.8; temperature, 28 ± 02 °C).
The pseudo-first-order kinetic parameters k1and qe are 0.042 min−1 and 0.100 mg/g, respectively. The values of pseudo-second-order kinetic model of fluoride adsorption on the clay adsorbent k2 and qe are 0.496 g/mg/min and 0.463 mg/g, respectively. The pseudo-second-order kinetic model has been considered to be the most appropriate and is widely used to describe the adsorption kinetics. The results show that the pseudo-second-order kinetic model fitted better than pseudo-first-order kinetic model because the correlation coefficient found in the linearization of pseudo-second-order kinetic model equation is higher (0.999) and the adsorption capacity is 0.463 mg/g nearly close to the experimental value (0.450 mg/g).
3.4 Adsorption isotherms
The extracted parameters using Freundlich and Langmuir isotherms are presented in Table 3. The 1/n value of the Freundlich equation is below one indicating a normal adsorption with a correlation coefficient of R2 = 0.984 according to the nonlinear regression. The values of the Langmuir parameters, q0 and b, are 3.74 mg/g and 0.064 L/mg, respectively, with relatively high regression coefficient (R2 = 0.938) obtained from nonlinear regression. The value of r for an initial fluoride concentration of 5 mg/L was found to be 0.096, which shows that the adsorption of fluoride onto clay is favourable.
Parameters of the Freundlich and Langmuir equations for fluoride adsorption isotherms on natural clay.
Freundlich model | Langmuir model | ||||
(mg/g (mg/L)n) | 1/n | R2 | (mg/g) | (L/mg) | R2 |
0.548 | 0.419 | 0.984 | 3.74 | 0.064 | 0.938 |
The modelling of adsorption isotherms by Freundlich and Langmuir equations showed that Freundlich isotherm fitted better than Langmuir isotherm because the correlation coefficient found for Freundlich equation is higher (0.984). According to the Langmuir theory, the uptake capacity of fluoride on natural clay of Bechar region is 3.74 mg/g.
It is clear from Fig. 7 that when temperature increases from 30 to 55 °C, the removal of fluoride increases slightly from 0.45 to 0.48 mg/g. The thermodynamic parameters for the uptake of fluoride on clay were determined at different temperatures using Log (aqe/Ce) = f (1/T) (Fig. 8). The thermodynamic data indicated that fluoride adsorption increased with increasing temperature and the positive value of ΔH0 indicated the endothermic nature of the adsorption of fluoride onto natural clay. The value of ΔS0 (+91.4) indicated that the randomness was favourite at the interface between solid and solution. The negative values of ΔG0 indicate that the adsorption process was spontaneous and according to the results, the adsorption process is characterized as endothermic nature (Table 4). This result is similar to several other studies on fluoride adsorption to clay particles such as Mg/Al/Fe layer double hydroxides [38], magnesium incorporated bentonite [39], attapulgite [40], micronized kaolinite [41] and pyrophyllite clay [42].
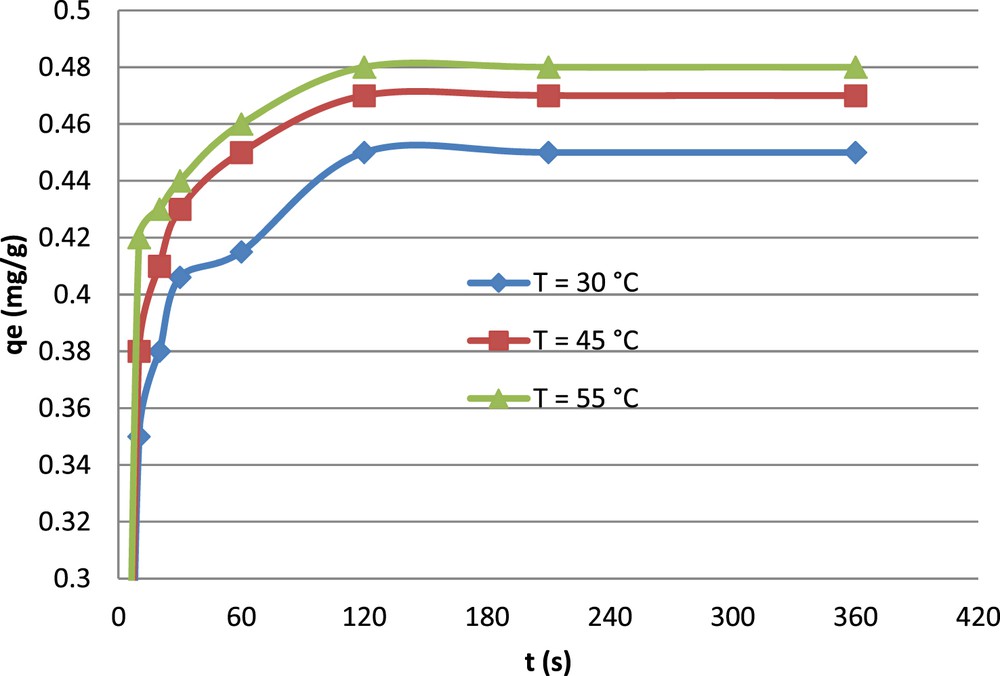
Isotherm adsorption of fluoride by natural clay (pH, 5.8; contact time, 3 h).
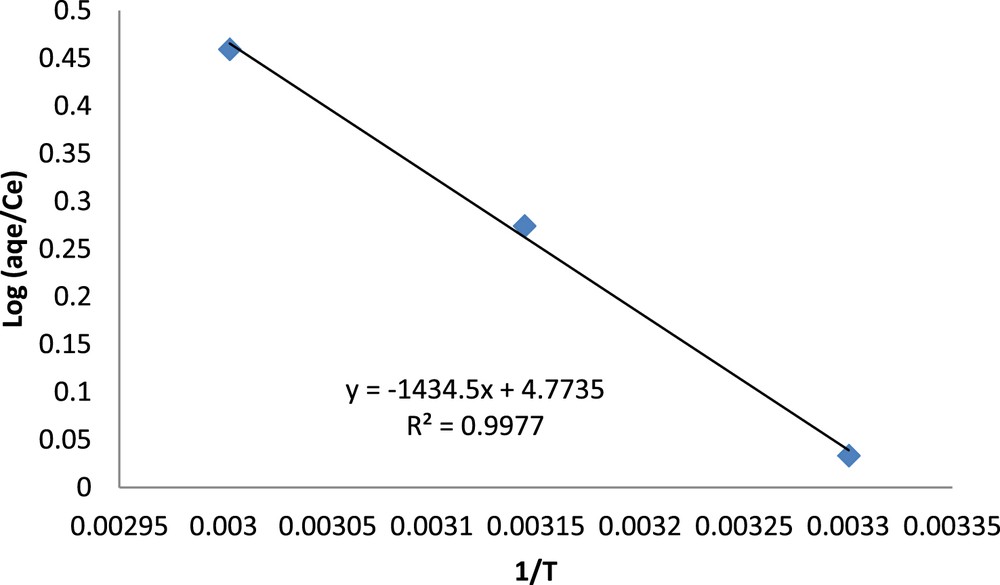
Determination of thermodynamic parameters of fluoride adsorption onto the clay.
Thermodynamic parameters for fluoride adsorption onto natural clay.
T (°C) | ΔH0 (kJ/mol) | ΔS0(kJ/K mol) | ΔG0 (kJ/mol) |
30 | 27.466 | 91.4 | −0.227 |
45 | −1.60 | ||
55 | −2.97 |
3.5 Co-ions effect
The fluoride in groundwater of Tindouf area is related to other co-ions such as NO3−, Cl−, Na+, SO42−, and HCO3−, which can enter in competition with fluoride ions during adsorption into natural clay sites. The competition of ions present in natural water and fluoride ions on the clay surface was also considered. To prepare synthetic water close to the natural water characteristics used in this study, different solutions were prepared by dissolving an appropriate amount of sodium salts of nitrate, chloride, sulfate and bicarbonate. Adsorption experiments were carried out by adding 50–300 mg/L of nitrate, chloride, sulfate and bicarbonate ions in individual solution of 5 mg/L fluoride. Then 10 g/L of natural clay of Tabelbala was added to each flask. The adsorption study was performed under these experimental conditions: initial pH 5.8, temperature 28 ± 02 °C, contact time of 3 h and agitation speed up to 200 rpm.
The presence of chloride and nitrate do not affect the adsorption of fluoride and a slight improvement in the removal of fluoride is observed (Fig. 9). Similar observations have been reported by Eskandarpour et al. [43]. In contrast, fluoride adsorption decreases in the presence of sulfate and carbonate ions. Fluoride removal decreases by sulfate ion may be assigned to the electrostatic repulsive forces, which decrease the possibility of fluoride interactions with the active sites present on the clay surface [44]. Carbonate ions show the harmful effect on fluoride uptake. This behaviour can be explained by the competition between fluoride and carbonate ions and may also be due to the high affinity of carbonate ions on the clay surface. Similar results were reported from Nan et al. [37], Kagne et al. [45] and Abhijit et al. [46], where the effective removal of fluoride is not considerably influenced by chloride, nitrate and sulfate but is notably influenced by carbonates.
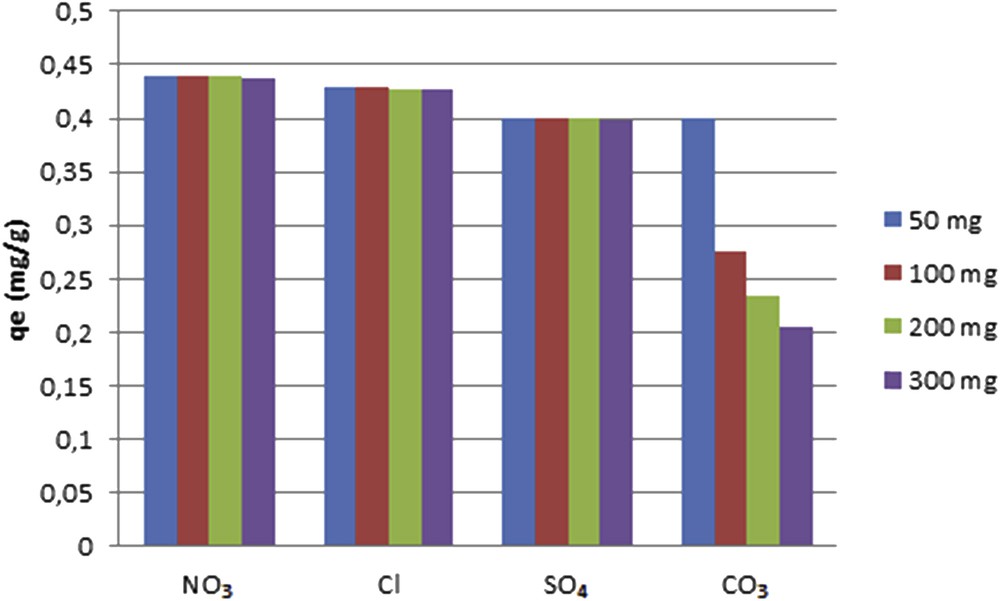
Effect of different concentrations of competing anions on fluoride adsorption by the clay adsorbent.
4 Conclusions
In this study, the removal of fluoride ion from aqueous solution using kaolinite clay was examined. Kaolinite clay was characterized using different analytical techniques. The major chemical compounds of natural clay are SiO2 (53.83) and Al2O3 (39.81), whereas its pHpzc and CEC are 6.4 and 8 mequiv/100 g, respectively. During adsorption tests, the optimum pH for maximum fluoride removal varies between 4 and 6 while the equilibrium time is 120 min. Adsorption kinetics systems are described by pseudo-second-order model. Furthermore, the Freundlich isotherm is suitable to describe the equilibrium tests. The adsorption capacity is 0.556 mg/g according to the Langmuir method. The results of thermodynamic data show that fluoride adsorption increased from 0.45 mg/g (90%) to 0.48 mg/g (96%) with increasing temperature from 30 to 55 °C. The positive value of ΔH0 indicates the endothermic nature of fluoride adsorption. The negative value of ΔG0 indicates that the adsorption process was spontaneous. The nitrate and chloride ions existing with fluoride ion in water do not affect the fluoride uptake into kaolinite clay. However, sulfate and carbonate decrease the fluoride elimination. According to the obtained results, the kaolinite clay could be used as an efficient and low-cost material for excess fluoride removal from groundwater.
Acknowledgements
This work was supported by la Direction Générale de la Recherche Scientifique et du Développement Technologique (DGRSDT) in Algeria through the Laboratory of Chemistry and Environmental sciences at the University of Tahri Mohamed Bechar (Algeria).