1 Introduction
A membrane can be defined as a selective partition, which under the effect of a driving force will permit or prevent the flow of certain elements between the two media it separates. A transfer force may be generated by a gradient of pressure, concentration, or electrical potential applied to induce the permeation through the membrane. In most cases, the membrane module consists of an inlet (feed) and two outlets. The part of the fluid that passes through the membrane is called the permeate, whereas the retentate is the liquid consisting of the retained elements.
Generally, a ceramic membrane has an asymmetrical structure composed of three layers (Fig. 1): The outer layer forms a macroporous support and provides a high mechanical strength for the fabricated membrane. The second layer is the inner layer ensuring the separation. The intermediate layer binds the inner and the outer layers [2].
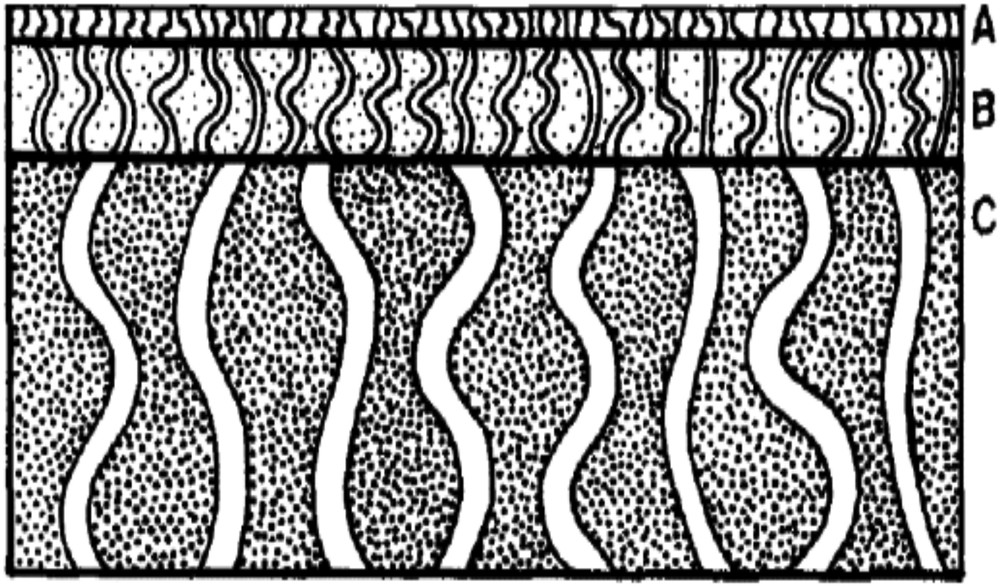
Schematic diagram of a composite membrane: (A) top layer, (B) intermediate layer, and (C) porous support [1].
Nowadays, the use of membrane separation processes has become more important in many industrial applications, especially as they have been proven to be highly efficient in many separation processes, including industrial effluent water treatment [3,4], air purification, food industries, and other environmental applications [5,6]. The research on new membrane technology would allow using a membrane process for solving many environmental problems at low cost.
Among the most commonly used materials for the manufacture of ceramic membranes we quote alumina (Al2O3), zirconia (ZrO2), titania (TiO2), silicon carbide (SiC), glass (SiO2), or a combination of these metal oxides, and other suitable materials including nonoxides (carbides, nitrides, borides, and silicides) and composites of combinations of oxides and nonoxides, as well as other clay minerals (e.g., kaolin, mullite, dolomite, etc.). Ceramic membranes are thermally stable, possess excellent tolerance to pH, and can withstand temperatures of up to several hundred degrees Fahrenheit.
In fact, remain challenge from the cost benefit point of view, the optimization of membrane filtration performance. For this reason, porous ceramic membranes have been used extensively in many industrial processes because of their many well-known advantages as compared with polymeric membranes [7–9]. Thus, many commercialized ceramic membranes have been made from expensive compounds, such as cordierite [7,10], titania, zirconia [11,12], and silicon carbide [13].
Recently, to reduce the cost of membranes researchers have focused their attention on the production of ceramic membranes using cheap raw materials, such as natural clay [14], apatite powder [15], dolomite, kaolin, bauxite [16–20], and mineral coal fly ash [21,22].
The preparation of a porous ceramic membrane with enhanced porousness and effective separation presents a great interest. Several researches used several pore-forming agents such as aluminum powder [19], sawdust, starch, carbon, or organic particulates [23–25].
Ceramic membranes are the most used in the industrial field. They are not easy subjects to bacterial contamination and abrasion as organic membranes [26]. Furthermore, they can preserve their flow and water permeability properties over time.
This article outlines an extensive overview of research projects in the preparation of low-cost porous ceramic membranes with high performances, used as filters in many different industrial processes and also for solving various environmental problems.
2 Manufacturing of ceramic membranes
Elaboration of ceramic membranes can be carried out in three steps: the first step consists in the formation of particle suspension, the second step consists in shaping the particle suspension into a membrane precursor with a desired geometry, and the final step consists in heating the membrane precursor [2]. The obtained substrate can be modified by the layer deposition method tailoring the membrane selectivity and other membrane properties.
Ceramic membrane supports can be fabricated using different methods depending on the application requirements, the desired membrane structure, and the specific materials. The most common manufacturing processes are slip casting, tape casting, pressing, extrusion, and freeze casting [27,28].
2.1 Slip casting method
Slip casting is a simple and economical technique occasionally used for advanced ceramic membrane preparation. This method has been used for a long time in the traditional ceramic industry [29]. This method has the advantages of shaping complex geometries and irregular forms and also achieving good material homogeneity.
Indeed, a particle suspension is well mixed and then poured into a porous mold, so that the solvents can diffuse through the pores because of the driving capillary action, forming a cake layer by particle precipitation on the internal surface of the mold, followed by a rapid consolidation step of the particle layer to avoid particle penetration through the mold (Fig. 2) [30]. This method has been applied to prepare a ceramic membrane from different low-cost materials, such as kaolin [31] and fly ash [22,32]. Generally, ceramic membranes prepared by slip casting are known for their high permeation properties, resulting in the presence of smaller pore size over thinner region. Jedidi et al. [22] have reported the use of the slip casting method for developing a porous tubular ceramic membrane based on mineral coal fly ash. The obtained membrane has a homogeneous surface without any macrodefect when heated at 800 °C, having an average pore diameter of about 0.25 μm and a hydraulic permeability of about 475 L/(h m2 bar). The obtained membrane was applied in the treatment of dyes from wastewater generated by the wash baths in the textile industry.

The principle of slip casting method to prepare ceramic membranes [30].
2.2 Tape casting method
Tape casting method is a widely used fabrication technique in the production of thin and smooth ceramic sheets. This technique, which was introduced by Glen N Howatt in the mid-1940s during the Second World War for the production of thin piezoelectric materials [33], has been known for a long time for the production of ceramic membranes. This technique involves pouring the powder suspension into a reservoir, which in turn passes under an adjustable casting knife that controls the thickness of the cast layer, defined by the gap between the knife blade and the moving carrier. After that, the ceramic tape obtained passes through a drying zone where solvent evaporation from the membrane surface takes place (Fig. 3) [34].

The principle of tape casting.
This method has been adopted by several researches for the fabrication of a ceramic membrane [1,9,35]. Nandi et al. [36] have elaborated circular discs of 52.5 mm diameter and 4.5 mm thickness from kaolin and other suitable low-cost materials such as quartz, calcium carbonate, sodium carbonate, sodium metasilicate, and boric acid. After sintering at 1000 °C, the obtained ceramic membrane has good properties with an average pore size, porosity, and flexural strength of 810 nm, 33%, and 8 MPa, respectively. Also, Jana et al. [37] have studied the use of clay and kaolin mixture to prepare a low-cost ceramic membrane by tape casting method, the obtained ceramic discs sintered at 1000 °C have shown good performances, with an average pore size, porosity, pore density, and flexural strength of 0.31 μm, 22%, 4.80 × 1012 m−2, and 12.81 MPa, respectively. Das et al. [35] have also synthesized an alumina membrane by the tape casting method, with pore size and porosity ranging from 0.1 to 0.7 μm and 25–55%, respectively. The obtained membrane has been found to be suitable for the complete removal of bacteria from water.
2.3 Pressing method
Pressing method is a well-known method used mainly for the fabrication of ceramic membranes for fundamental research. This method is commonly based on the pressing of a dry powder by means of a press machine. Indeed, after mixing the powder homogeneously (raw material with pore-forming agent ratios), the obtained product is pressed uniaxially (Fig. 4), that is, it undergoes stress by a punch in a mold with immobile walls to obtain the desired membrane support shape. This process allows very high production rates. For consolidation, the obtained flat membrane support must go through heat treatment, generally at a temperature reaching that of the used materials sintering [38].
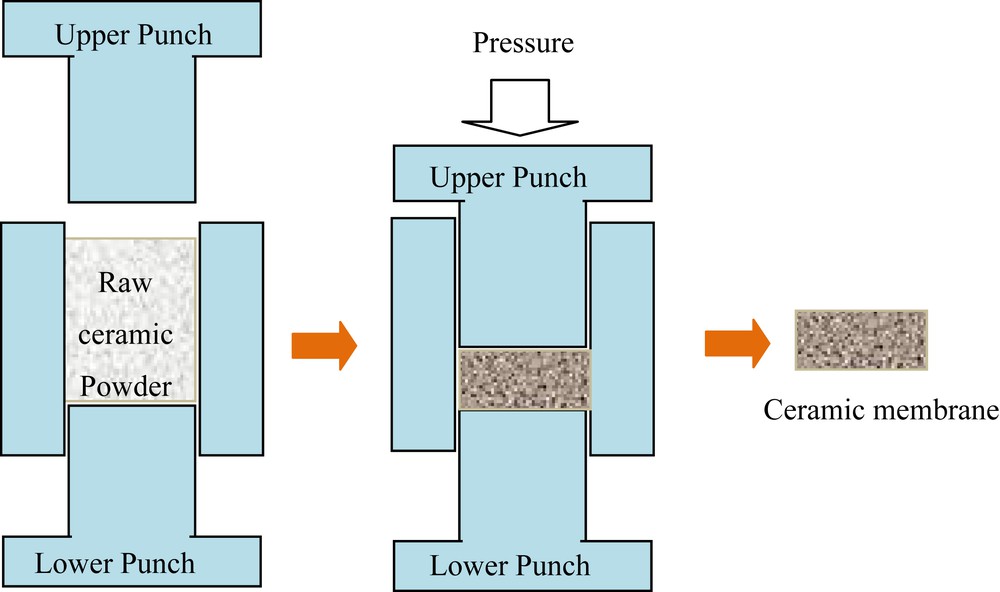
The principle of pressing method.
Generally, ceramic membranes produced by the pressing method have well-defined characteristics, such as uniform porosity and homogeneous physical properties over the total membrane part. Del Colle et al. [39] have applied this method to manufacture tubular porous and supported ceramic membranes based on zirconia. The microfiltration (MF) porous membranes exhibit an average pore size of 1.8 μm, whereas the ultrafiltration (UF) ones present an average pores size of 0.01–0.03 μm in the top layer and 1.8 μm in the support. The obtained ceramic membranes were intended for the demulsification of oil–water suspension. Hristov et al. [40] have also prepared low-cost ceramic membrane support by semidry pressing of natural zeolite powder. The discs with diameter 30 mm and thickness 4 mm formed after firing at different temperatures (from 800 to 1000 °C) presented high porosity (38%) and very uniform pore distribution. In another study, Huang et al. [41] have introduced the pressing method at 25 MPa for producing the low-cost ceramic membrane based on kaolin. Likewise, Issaoui et al. [19] have used the dry pressing method at 66 MPa for producing flat ceramic membranes from kaolin and cermet membrane supports from kaolin–aluminum powder.
2.4 Extrusion
Extrusion is also a conventional method for producing ceramic membranes, extensively used for the fabrication of porous tubular configuration. In this method, a plasticizing agent and binding agent are necessary for the production of a ceramic pulp with rheological characteristics (plasticity, hardening degree after drying, etc.) to make shape by extrusion possible [42]. The shaping method of the paste depends on the geometry of the final membrane support. It is a plastic continuous deformation process, in which paste is forced with a relatively simple piston press to flow through the die opening of a smaller cross-sectional area, which dictates the shape, pore size distribution, and the porosity of the final product (Fig. 5) [43]. The raw ceramic membranes are dried (at room temperature) and then treated under high-temperature conditions, generally at a low ramping rate to avoid the formation of cracks on the ceramic layer, up to the sintering temperature of the used material. The extrusion process has been commonly used to prepare different ceramic membranes using inexpensive materials. Jedidi et al. [44] have fabricated low-cost tubular ceramic support for the membrane by extrusion of fly ash paste. The obtained samples sintered at 1125 °C have shown homogeneous surface exempt of macrodefect, with mean pore diameter and the pore volume of 4.5 μm and 51%, respectively. Elmoudden et al. [45] have manufactured also porous tubular ceramic membranes from calcinated clay using the extrusion method. The sintered membranes at 1130 °C have shown a mean pore diameter of 9 μm and porosity of 38%.
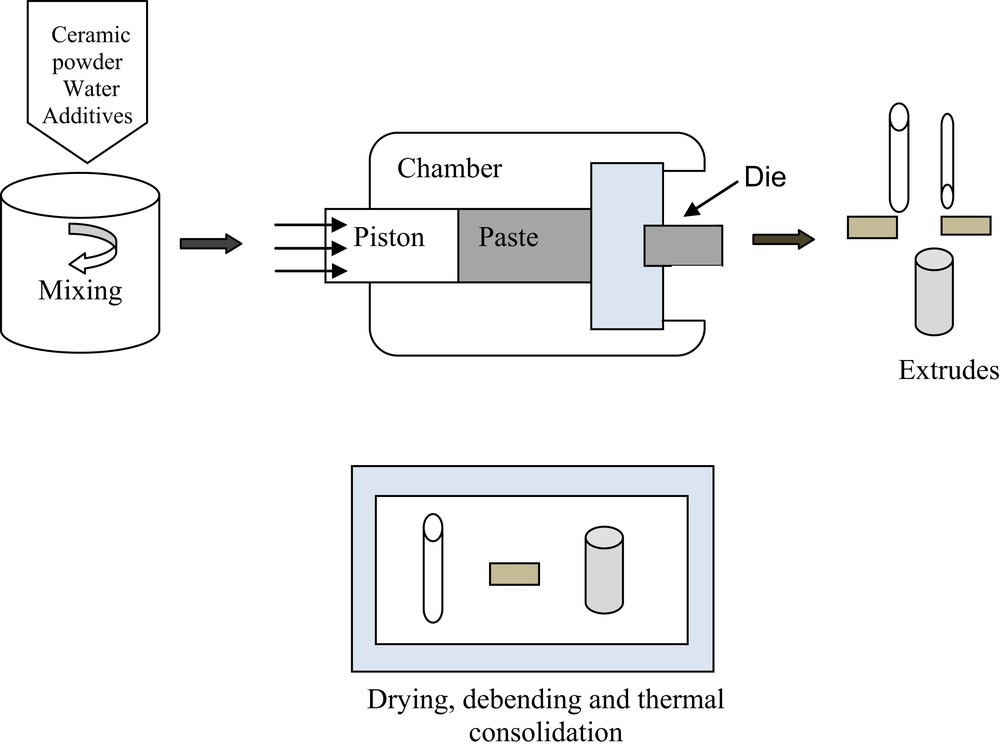
The principle of extrusion method.
Also in other studies, Boudaira et al. [46] have elaborated tubular ceramic supports for membranes from local kaolin and calcite mixtures using the extrusion method. These supports sintered at 1150 °C with homogeneous surfaces and interesting characteristics (an average pore size of about 4 μm, a porosity ratio around 50.5%, and a tensile strength ≈28 MPa) were selected to be substrates for the membrane layers used in MF.
Also in the same frame, recently Issaoui et al. [47] have manufactured low-cost tubular macroporous supports for ceramic membranes using the extrusion method. After sintering at 1250 °C, the water permeability measured was about 612 L/(h m2 bar) for samples elaborated from a powder mixture containing 80 wt% kaolin and 20 wt% of starch.
2.5 Freeze-casting method
The freeze-casting method also called ice-templating is considered as one of the most recent, attractive, and novel technique for the preparation of highly porous ceramic membrane structures that are hierarchically organized. In this method, ceramic slurry is generally frozen by the bottom and followed by sublimation of the frozen solvent under reduced temperature and pressure. Then, following a repetitive pattern the freezing of the ceramic slurry results in the growth of upright solvent crystals along the freezing direction and the related rejection of ceramic particles between these crystals (Fig. 6).
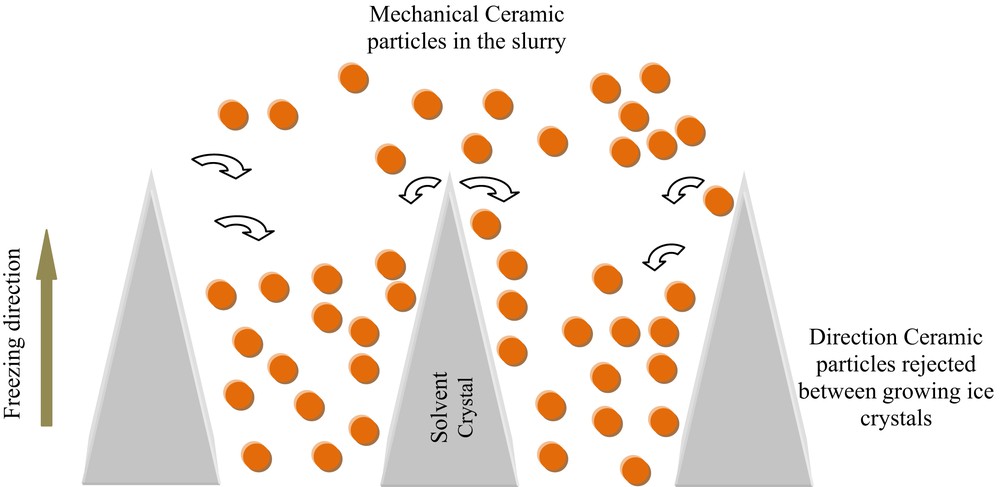
Ice crystals growth during the freezing phase and the related distribution of ceramic particles excluded between the ice crystals.
At the end of these steps, the obtained porous raw membranes showed a main shortcoming that lies in their low mechanical properties. To overcome this defect, a thermal treatment is required at the vicinity of the sintering temperature of the used material, during which the porous structure acquires its consolidation and its walls are densified [48]. This technique allows elaborating ceramic membrane that can be used in very promising applications such as the generation of energy [49].
An interesting work has been performed by Liu et al. [50]. They have used the freeze-casting technique to manufacture porous alumina UF membranes with good performance for anionic dye separation. After calcination at 1150 °C, the elaborated freeze-cast membrane yielded a porous structure of about 33 nm and bending strength of 36.5 MPa. Also, the membrane exhibits high rejection rate of 99.6% toward Direct Red 80 solutions with permeate flux (PF) of about 6.39 × 10−5 m3/(m2 s) at applied pressure of 2 bars.
Another study that introduces the freeze-casting method was conducted by Liu et al. [51]. They have fabricated tubular porous mullite membrane supports, with gradient unidirectional aligned pores. The results show that the characteristics of the ceramic membrane supports can be adjusted by controlling the freezing temperature, mullite powder particle size, and solids loading. Tubular porous mullite supports with pore channel size of 6.5 μm, porosity of 59.66%, and compressive strength of 54.11 MPa were obtained when the freezing temperature, average particle size, and solids loading was −100 °C, 2.8 μm, and 35 vol%, respectively.
3 Membrane classifications
The most popular classifications of a membrane are based on their morphology, geometry, chemical nature, and separation regime [52].
3.1 Classification according to morphology
We could distinguish two types of membranes according to the structures of the different construction materials:
- 1. Symmetric (isotropic) membranes: they are dense or porous, and generally refer to the membrane having a relatively uniform pore size with a homogeneous structure throughout the entire thickness (Fig. 7a) [53]. Several researchers focused their attention on the preparation of isotropic membranes. For example, Issaoui et al. [19] have fabricated symmetric flat cermet supports for membranes from a mixture of kaolin and aluminum, with open porosity reaching 28.5%. Likewise, Zhang et al. [54] have produced a thin tubular isotropic membrane from ceramic powder with mechanical strength of 122 MPa after sintering at 1200 °C.
- 2. Asymmetric (anisotropic) membranes that present a pore size gradation decreasing toward the surface. This type can also be divided into two subgroups:
- - Membranes prepared from the same material exhibit gradation of the pore size from one side to another. Among recent work on this type, we can quote Rekik et al. [55] who have developed a tubular asymmetric UF membrane from kaolin, exhibiting average pore sizes of about 1 μm and 11 nm for the support and the active layer, respectively, with hydraulic permeability value of 78 L/(h m2 bar). In another study, Hubadillah et al. [56] have prepared low-cost flat ceramic supports from kaolin, with pore size ranging from 0.38 to 1.05 μm, porosity of 27.7%, and mechanical strength of 98.9 MPa.
- - Composite membranes or skinned membranes are those formed by deposition of thin film with necessary characteristics onto another porous film that usually acts as a support [57]. They also have an active layer on the side of the membrane with much smaller pores than the rest of the membrane (Fig. 7b). Among the recent publications, Almandoz et al. [58] have fabricated tubular composite membranes from natural aluminosilicates (clay, feldspar, quartz, bentonite, and alumina) by depositing a thin active layer on the support. After sintering at 1200 °C, the composite membranes showed good structural characteristics, hydraulic permeabilities between 10 and 274 L/(h m2 kPa), porosities close to 50%, and pore diameter ranging from 0.08 to 0.55 μm for the filtration layer.

Symmetric (a) and asymmetric (b) membranes.
3.2 Classification according to the membrane geometry
Ceramic membranes can be categorized according to their geometry into two main groups: first, flat sheet membranes, which are used in the construction of flat sheet, disc, spirally wound, plate, and frame modules. Second, cylindrical membranes, which are used in cylindrical fiber modules. On the basis of the dimensional differences, the following types of cylindrical membranes may be distinguished [59]:
- • Tubular membranes with an internal diameter larger than 10 mm.
- • Capillary membranes with an internal diameter between 0.5 and 10 mm.
- • Hollow fiber membranes with a diameter smaller than 0.5 mm.
3.3 Classification of membranes according to the separation mechanism
Membrane separation processes are dependent on the size of the separated element and separation mechanisms. Generally, there are three membrane separation mechanisms [60]:
- • Sieving effect mechanism: a very similar phenomenon occurring in the normal filters. This mechanism separation is based on the difference in the particle size, meaning the pore size of the membrane should be smaller than the particle size, which is to be separated. Expressions like macrospores, mesopores, and micropores are used to describe the pore size in the membrane for MF, UF, and nanofiltration (NF).
- • Solution diffusion mechanism: this is related to the difference in solubility or diffusion coefficient of each component in the membrane, this principle is used in operations like reverse osmosis (RO) and requires a dense membrane.
- • Electrochemical mechanism: this is based on the difference in the charges of the mixture components to be separated. Generally, nonporous ion-exchange membranes are used in operations like electrodialysis.
3.4 Classification according to the chemical nature
Membranes are classified according to their chemical nature, that is, organic, inorganic, and organic/inorganic hybrids. Organic membranes made from polymers such as polyamide and polysulfone derived from cellulose have been known for a long time. These membranes are not stable at high temperature and are sensitive to oxidizing agents [60]. However, they may undergo sterilization with steam without altering their structure.
For the inorganic membranes, they are composed entirely of mineral materials (ceramic, glass, silica, etc). These types of membranes present several advantages, for instance, they are much more thermally and chemically stable than organic membranes and show both wide tolerance to pH and high resistance to chemical degradation. Table 1 summarizes a comparison between organic and inorganic membranes in terms of their material characteristics, advantages, and disadvantages.
Comparison between organic and inorganic membranes [61].
Properties | Organic membranes | Inorganic membranes |
Material | Rubbery or glassy type membranes based on the operating temperature | Inorganic materials, i.e., glass, ceramic, silica, etc. |
Characteristic | Rigid in glassy form and flexible in rubbery state | Chemically and thermally stable, mechanically robust, operational under harsh feed condition |
Advantages | Cost-effectiveness, good selectivity, easy processability | Withstand harsh chemical cleaning, ability to be sterilized and autoclaved, high temperature (up to 500 °C), and wear resistance, well-defined and stable pore structure, high chemical stability, long life time |
Disadvantages | Fouling, chemically nonresistant, limited operating temperature and pressure, short life time | Fragile, rigid |
4 Critical industrial applications of ceramic membranes
Over the past few years, membrane technology has gained grounds and found wide-ranging applications across a whole range of industries. Ceramic membrane systems are not only chosen as a filter but for other capabilities, namely their broader ability to coagulate, precipitate, or oxidize and eliminate chemicals. For this reason, specific applications and developments have fueled its growth and provided the seeds for subsequent opportunities. The first large-scale commercial success of porous ceramic membranes has been applied in the food and beverage industries [62–64]. However, different areas such as biotechnology, pharmaceutical, petrochemical, water treatment industries, environmental problem, and many more industrial applications have been reported in the literature [65,66]. The following sections provide more information on the possible areas of ceramic membrane applications.
4.1 Food processing industry
Membrane processes are used to replace conventional processing methods. They can be considered as innovative methods for the production of requisite ingredients for the new food product development or their improvement [67].
Generally porous ceramic membranes based on membrane processes driven by pressure namely MF, UF, NF, and RO have been widely used. Two main classes of applications can be distinguished: clarification and concentration of suspended particles, molecules, and microorganisms from soluble components. In what follows the main applications of membrane processing in food processing are presented.
4.1.1 Dairy processing industry
Ceramic membrane filtration in the food industry is mainly applied in the dairy processing [68]. This process, which is considered as the first membrane processing at an industrial scale, appeared since the early 1960s [69]. Dairy industry is based on applicability of membrane processes driven by pressure (MF, UF, NF, and RO). As shown in Fig. 8, all major components of milk can be separated [70]. Raw milk may be defined as polydisperse system with suspended particles and soluble molecules, such as fat globules, casein micelles, whey proteins, lactose, and minerals. These compounds may have different charges and sizes; therefore, their separation by membrane processes can be feasible [71]. Generally, MF with ceramic membranes is the most adopted technique for milk processing. It can be used for the concentration of milk and whey and also for the elimination of bacteria [71]. Experiments have also been conducted on milk protein concentration. Almandoz et al. [58] have fabricated tubular composite MF membranes from natural aluminosilicates (already cited previously) and tested for goat milk pasteurization. The obtained results show a high bacterial removal (87–99%).
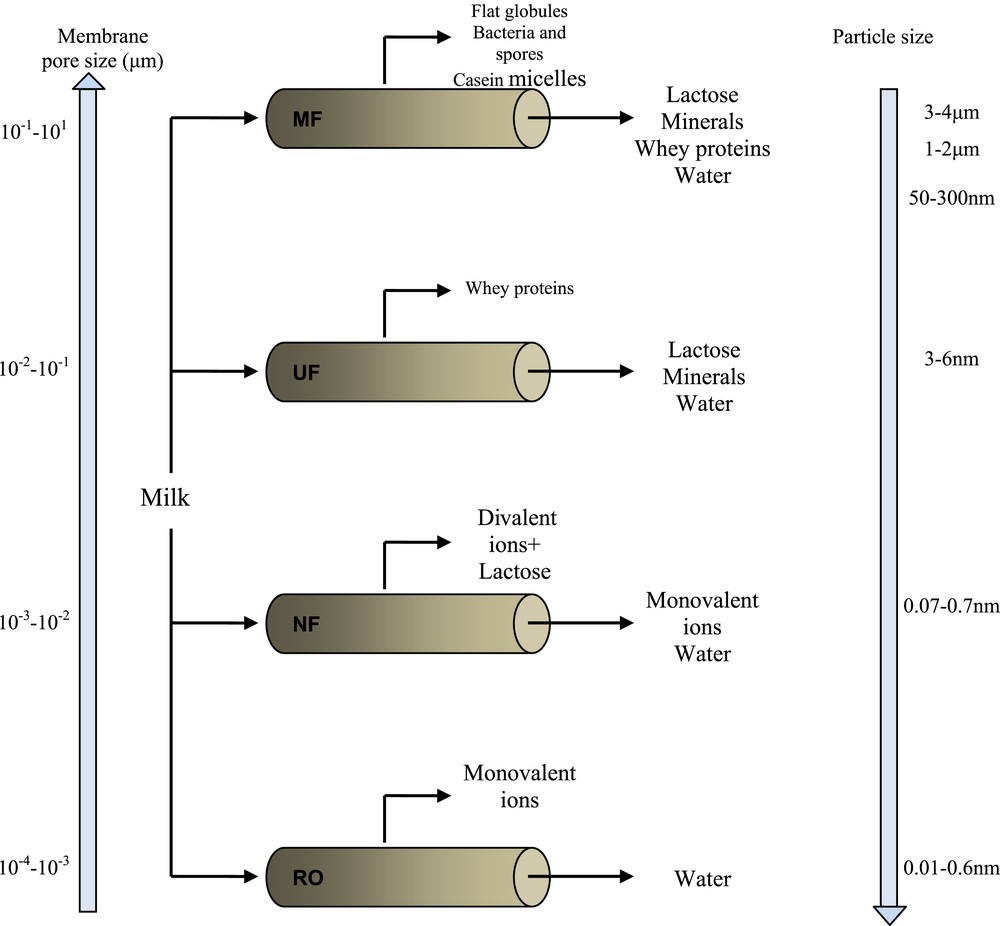
Milk processing with membrane technology [70].
Likewise, Erdem et al. [72] have prepared a ceramic composite membrane based on alumina powder and tested for whey protein separation. The obtained membrane has shown good separation properties, with relatively high protein content and low lactose retention of about 80% and 7%, respectively.
Also Popović et al. [73] have investigated the performance of tubular ceramic membranes made of alumina during the filtration of whey reconstituted solution, showing a PF reduction above 90%.
4.1.2 Protein separation and concentration
Protein concentration and separation is another sector for low-cost ceramic membrane application in food processing, namely soymilk, fish, animal blood, egg, and gelatin solutions [71,74,75]. Ishak et al. [76] have studied protein separation and purification using hydrophilic modification of alumina membranes. They changed the character of alumina (membrane active layer) from hydrophilic to superhydrophilic using graphene derivatives. The obtained superhydrophilic ceramic membrane surface has a great impact on anticlogging and PF properties, and therefore it improves protein separation and purification process performances.
Kuca and Szaniawska [77] have investigated the performance of separation processes by using a 150 kDa ceramic membrane based on (Al2O3/TiO2 and ZrO2) for the recovery of proteins from the fish industry effluents. They showed that membrane process does not only reduce the biochemical oxygen demand and chemical oxygen demand (COD) from the fish industry salted wastewater but also allows the recovery of high protein content (81%).
Biron et al. [78] characterized tubular mullite ceramic membranes and then applied them in the separation of proteins. Depending on the filtration test of a protein solution composed of trypsin and albumin from egg and bovine serum, protein retention rates were 46%, 76%, and 89%, respectively.
Yang et al. [79] have used low-cost ceramic MF membranes elaborated from attapulgite, for the purification of cellulose fermentation fluid to improve the quality of cellulase products. They focused on the effect of the operating conditions and the membrane microstructure on the decline in membrane flux. Results have shown that the appropriate operating conditions were as follows: T = 30 ± 3 °C, ΔP = 0.10 MPa, and flow velocity = 4 m/s. Therefore, the attapulgite MF membrane having a mean pore size of 0.12 μm is appropriate for the treatment of cellulase fermentation fluid.
4.2 Beverages and drinking water
The scope and frequency of beverage and drinking water analyses are regulated in most countries. Besides, sensory testing and microbiological analysis compliant with limit values for impurities are most crucial. Among these methods, we find the application of membrane processes whose objective is to eliminate precipitates, microorganisms, and suspended particles.
4.2.1 Potable water
Water sustains human life and its purity cannot be compromised at any cost. However, conventional water treatment processes including filtration, sedimentation, coagulation, and flocculation are not very effective in the production of high-quality drinking water. Membrane filtration has been integrated in this area for meeting these stringent regulations. The production of drinking water by the membrane process using a ceramic membrane has been carried out since 1984 [80]. It is mostly used for removing precipitates, turbidity, suspended particles [81], and then virus and bacteria from surface waters, such as rivers and lakes, when using a small-scale membrane treatment system [82–85].
The production of drinking water using ceramic filtration membranes has recently attracted much interest. For example, Alami-Younssi et al. [86] have shown that nitrate ions can be removed from soft drinking water by NF through an alumina membrane. Nevertheless, the poor penetrability of NF membrane has been a constraint for industrial applications.
MF using ceramic membranes was applied to reduce trihalomethane precursors from drinking water, remove turbidity, and to act as disinfectant at the same time [6]. In the same vein, Ozdemir [87] has produced safe and quality potable water using the UF system through a porous tubular ceramic membrane based on alumina. The treatment of raw water has led to removing nearly 75% and 85% of ferrous and turbidity contaminants, respectively.
Moreover, seawater desalination represents an increasingly important solution to the rising drinking water scarcity afflicting many of the world's regions. On the basis of this approach, ceramic membranes are often used because of their high flux and stability. Zhu et al. [88] have investigated the desalination of seawater solution (0.3 wt% total dissolved solids) using zeolite membranes, which reached a high rejection (>93%) for all major seawater ions.
In another study, Gazagnes et al. [89] have desalinated sodium chloride solutions and seawater using a hydrophobic zirconia membrane (grafted with perfluorodecyltriethoxysilane). A high salt rejection rate (>95%) has been reached.
4.2.2 Juice clarification and concentration
Fruit juice clarification is one of the oldest separation processes using ceramic membranes [90]. This process can replace the conventional separation, thanks to its speed, performance, labor reduction, as well the operation and energy cost reduction. Comparing polymeric and ceramic membranes in orange and lemon juice clarification, Capanelli et al. [91] have concluded that ceramic membranes made from alumina as low-cost material could be more energy efficient than polymeric ones for industrial applications, giving higher PFs at a lower Reynolds number.
Nandi et al. [92,93] have studied the filtration of mosambi and orange juices using a low-cost ceramic membrane based on kaolin. They found that after MF test, essential properties such as a total amount of soluble constituents of the juice, pH, acidity, and juice density were almost unaffected. Although a significant improvement in other juice characteristics was observed like color, clearness, and alcohol insoluble solid. The obtained clarified juice can maintain its quality even for 30 days when refrigerated. Qin et al. [94] have used three different low-cost fly ash precursor inorganic membranes for the clarification of centrifuged kiwi fruit juice. The evaluation of the permeation characteristics proved that 1.25 μm was the optimal pore diameter of membranes used in the clarification of kiwi fruit juice.
Emani et al. [95] have synthesized low-cost inorganic MF membranes based on kaolin by the dry compaction method for the clarification of mosambi juice. Optimum fabrication requirements have shown that the MF performance of the membrane fabricated at 49 MPa is highly satisfactory for this application. A membrane flux of about 90 to 44 × 10−6 m3/(m2 s) at 206.7 kPa has been attained. The enzyme-treated centrifuged juice presents negligible alcohol insoluble solid content.
4.3 Alcoholic beverages
For many years, ceramic membranes have been used in a large range beverage production. Experiments have shown that this can be extended to the clarification and sterilization of alcoholic beverages. For instance, Youzhi et al. [96] have filtered vinegar using low-cost tubular ceramic membranes based on alumina. Optimization of operation parameters shows that a membrane with a pore size of 0.1 μm operating at room temperature under a transmembrane pressure of 0.14 MPa and a cross-flow velocity of 2 m/s is suitable for clarifying vinegar with high quality and low-haze potential even for 2 years.
4.4 Environmental applications
Over the past few years, extensive research was carried out to develop low-cost ceramic membranes with high performance for different environmental applications, such as the treatment of dairy industry wastewater, oily wastes, textile sludge, and other industrial wastewaters. In fact, the use of ceramic membranes in the field of wastewater treatment is still limited because of their higher cost [97], as it is approximately 10 times higher than that of polymer membranes [98]. Hence, the need for a low-cost material for the fabrication of ceramic membrane is needed.
Membrane process can be used for reducing the amounts of wastewater polluting the environment and also for the recovery of valuable substances from sewage. Accordingly, new high quality porous ceramic membranes elaborated from low-cost materials have been developed to improve filtration efficiency, decrease energy usage, and lower the amount of time lost on maintenance. Numerous studies concerning wastewater treatments have been elaborate. For example, Kumar et al. [99] have investigated the treatment of wastewater generated by a local dairy industry using a novel low-cost tubular ceramic membrane. They have manufactured a porous tubular ceramic membrane via extrusion of natural clay paste, with 53% porosity, 0.309 μm pore size, and 5.93 × 10−7 m3/(m2 s kPa) of hydraulic permeability. Their results obtained from tangential MF process have shown that an increase in cross flow rate and applied pressure lead to a decrease in the percentage of COD removal up to 91% (135 mg/L) in the permeate stream with a flux of 2.59 × 10−6 m3/(m2 s), which is well within the permissible limit for wastewater discharge into the environment. These results prove the potential suitability of the fabricated ceramic membrane in dairy wastewater treatment to attain acceptable limit (<200 mg/L) of the permeate stream.
The main reason for wastewater treatments using a ceramic membrane elaborated from low-cost materials has been reported by Parma and Chowdhury [100]. They have prepared circular disc-shaped membranes from locally available clay (red mud with major constituents of silicon dioxide (SiO2), aluminum oxide (Al2O3), and sodium oxide (Na2O)) by paste casting method. MF tests have shown that the resulting porous ceramic membranes are convenient for oily wastewater treatment. The ones sintered at 800 °C have reached a maximum rejection rate of 53% for crude oil from crude oil–water emulsion.
Abbasi et al. [101] have synthesized ceramic membranes from kaolin clay and studied their performances in MF. It was shown that mullite ceramic membrane manufactured by extruding and calcining kaolin clay can be used as an advanced method for the treatment of oily wastewaters. Thus, the MF is a feasible and advantageous method for the treatment of desalted wastewater effluent. According to the obtained results, the ceramic membrane reaches high PF and rejection, with the following experimental relationships: PF increases with increasing volumetric flow rate, temperature, and pressure; however, it decreases with increasing oil concentration. Rejection rises a little with increasing pressure, salt concentration, and oil concentration, but it drops with growing volumetric flow rate and temperature. The results also show that the MF treatment is very effective in the reduction of total organic carbon (TOC), total suspended solids, and turbidity. Investigating the effects of different operating parameters leads to select the best operating conditions employing from 250 up to 3000 ppm of condensate gas in water emulsions as synthetic feed. The obtained reduction of TOC was greater than 94%.
In another work, Abbasi et al. [102] have studied the effect of adding powdered activated carbon to mullite, on the one hand, and mullite–alumina mixture (50/50 wt%) on the other hand on TOC reduction. Their experimental results prove that the addition of high concentration (1200 ppm) powdered activated carbon enhances the elimination of TOC from 93.8% to 97.4 % and from 89.6% to 92.4 % for mullite and mullite–alumina MF membranes, respectively.
Shokrkar et al. [103] have carried out experimental and modeling studies on the separation of oil from industrial oily wastewaters using mullite ceramic membranes manufactured from kaolin clay and α-alumina powder. The use of mullite ceramic membrane resulted in high TOC and COD elimination corresponding to 94% and 89%, respectively.
In another work, Nandi et al. [104] have treated oily wastewater using a low-cost ceramic membrane made from inorganic precursors, such as kaolin, feldspar, quartz, boric acid, sodium carbonate, and sodium metasilicate. MF tests of synthetic oil–water emulsions with an initial oil concentration of 250 mg/L show that the membrane can reach 98.8% oil rejection efficiency and 5.36 × 10−6 m3/(m2 s) PF after 1 h of filtration performed at 68.95 kPa transmembrane pressure. Therefore, this ceramic membrane may be pertinent in the treatment of oil–water emulsions to yield permeate streams that can meet stricter environmental legislations (<10 mg/L).
In the same vein, textile, paper, dyestuff, and laundry industries use large amounts of water and chemicals for wet processing, and therefore generate several types of pollutants arising from the raw materials themselves and also from the residual chemical reagents used for processing [105].
Because of the increasingly stringent restrictions on mineral and organic contents of industrial effluents, it is necessary to eliminate the pollution load from wastewater before it is discharged to the environment. Several works have been conducted for the treatment of colored wastewater using a low-cost ceramic membrane. For instance, Jedidi et al. [22] have elaborated tubular ceramic membranes based on coal fly ash mineral. The obtained filters were used in the treatment of dyes present in wastewater resulting from the wash baths of the textile industry. Table 2 presents the results of MF using the elaborated ceramic membrane compared with a commercial alumina membrane. It has been found that MF results using a fly ash membrane proved to be effective as that obtained with an alumina membrane in removing the COD, turbidity, and color from wastewater. The conductivity as well as the turbidity and the absorbance of the solution were a little less than the one obtained with the commercial membrane, whereas the COD concentration was a little bit higher. This can be explained by the selectivity of the fly ash membrane, which can be different from the commercial one because of specific surface properties, like electrostatic interactions that can be different than the properties of the commercial membrane because of their chemical composition.
Results of the effluent before and after MF at 1 bar: using ash and commercial alumina membranes [22].
Sample | Conductivity (μs/cm) | Turbidity (NTU) | COD (mg/L) | Absorbance at 600 nm |
Raw effluent | 6.16 | 45.5 | 3440 | 0.104 |
Fly ash membrane permeate | 5.38 | 0.58 | 880 | 0.010 |
Alumina membrane permeate | 5.6 | 0.62 | 834 | 0.013 |
Issaoui et al. [19] have prepared porous flat cermet membranes from kaolin and aluminum powders. The membranes were then tested for the filtration of an indigo blue solution. The dead-end filtration results show that it is possible to completely remove the color of the initial solution (containing blue dye), with a significant retention of dyes in the concentrate and a complete discoloration of the solution (Fig. 9).
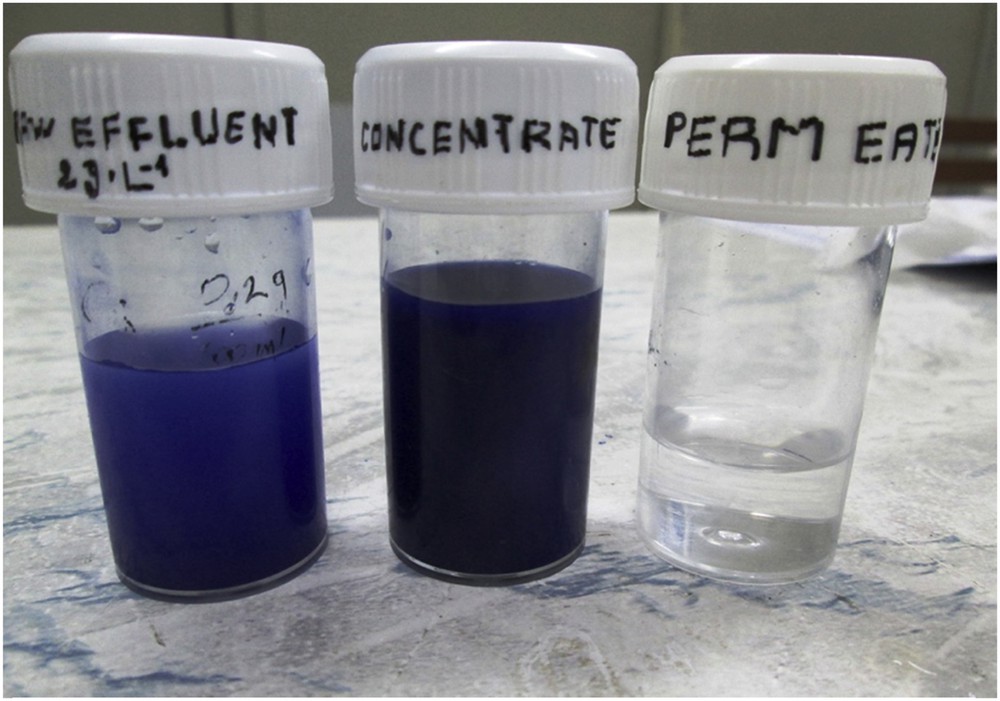
Results of decolorization obtained after the filtration of an aqueous solution containing the Indigo blue dye (T = 25 °C, P = 6 bar): permeate solution (on the right), retentate (in the middle), and the initial solution (on the left) [19].
Silva et al. [106] have used inexpensive raw materials, namely, kaolin and ball clay to synthesize tubular ceramic membranes and used them in the treatment of textile industry wastewater. Their results show the effectiveness of the elaborated ceramic membranes with a decrease in turbidity and discoloration, reaching approximately 100% of rejection of the solid particles.
In the same context, Nasir and Faizal [107] have investigated an alternative treatment for removing cadmium from pulp industry effluent using ceramic membranes elaborated from a mixture of 87.5% natural clay, 10% rice bran, and 2.5% iron powder. The results show that these membranes were able to reduce cadmium concentration up to 99%.
In another study, Han et al. [108] have prepared ceramic membranes from dewatered sludge, fly ash, and clay. Water filtration tests showed that heavy metals were not leached during the filtration process, which proved that the obtained ceramic filters were safe for wastewater treatment. This was associated with the solidification of heavy metals in the form of metallic oxides during the calcination process. Other low-cost tubular composite ceramic membranes have been fabricated by Almandoz et al. [58] using natural aluminosilicates (previously mentioned). Their investigations have shown that composite tubular membranes were suitable for wastewater treatment. The obtained results have shown an insoluble residue rejection of 100% and high bacterial removal ranging from 87% to 99% of slaughterhouse wastewater treatment and goat milk pasteurization.
For their part, Hasan et al. [109] have developed a low-cost membrane bioreactor (MBR) made of 80% clay soil and 20% rice bran. They showed that the obtained hollow cylindrical ceramic filters would be appropriated for wastewater treatment, removing arsenic from groundwater.
Further research in the field of environmental protection using membranes has been conducted, for example, Zorpas [110] investigated the combination of MBR and chemical oxidation process for the treatment of household heating wastewater. The results prove that the immersed hollow fiber module (with a nominal pore size of 0.04 μm, a total membrane surface area of 0.047 m2, and made from polyvinylidene difluoride) combined with a reactor was found to be a more promising process than the application of Fenton oxidation technique for the effluent discharge with the least environmental impact.
In the same vein, Aileen and Albert [111] have studied modeling MBR systems, specifically for municipal wastewater treatment, which can yield high-quality water with reported removal percentages of 95%, 98%, and >99% for COD, biochemical oxygen demand, and total suspended solid, respectively.
Also, Zhang et al. [112] have used a flat stainless steel membrane with homogeneous and tighter pore size in an experimental submerged MBR for the treatment of synthetic domestic sewage. The used membrane was quite effective in the elimination of organic substances and COD removal efficiency achieving 97%. Thus, the use of stainless steel membrane in a MBR for wastewater treatment is economical.
5 Ceramic membrane cost
Industrially, the competitive aspect of membrane technology lies in its cost. Indeed, some conventional ceramic membranes available for industrial scale operation are expensive, including manufacturing and raw material costs. An α-alumina porous tubular ceramic membrane with average pore diameters varying from 1000 to 6000 nm costs between $500 and $1000/m2 (Céramiques Techniques, 2007).
Although, an approximate cost of other fabricated ceramic membranes based on kaolin and other suitable low-cost materials (quartz, sodium carbonate, calcium carbonate, boric acid, and sodium metasilicate) with average pore diameters ranging from 550 to 810 nm was estimated to be $130/m2 [36].
In another study, Nandi et al. [104] have used low-cost ceramic membranes made from inorganic precursors such as kaolin, quartz, feldspar, sodium carbonate, boric acid, and sodium metasilicate with an estimated cost of $220/m2 for the treatment of oily wastewaters.
Likewise, Kumbhaj et al. [113] have prepared low-cost flat ceramic support for membranes from natural clay, kaolin, and small amount of binding materials (sodium carbonate, sodium metasilicate, and boric acid) with an average pore diameter of 4000 nm. The total membrane fabrication cost (including pressing and sintering cost) was estimated to be $25.5/m2.
6 Conclusions
The filtration performance is almost always a question of optimization and balancing between the desired separation efficiency and the PF needed for the process to be economically interesting. As the literature review showed, the manufacturing process of ceramic membranes from cheap materials is related to its natural abundance or its low-cost availability.
Including some fundamentals of membranes and intrinsic characteristics, ceramic membranes with high performances can be fabricated using different methods, including slip casting, tape casting, pressing method, extrusion, and freeze-casting method.
Lately, ceramic membranes have become competitive as compared with polymeric membranes as they offer unique material and performance advantages. The possibility of using low-cost materials for the elaboration of ceramic membranes allows their implementation in cost-sensitive fields.
According to the literature, ceramic membranes can be used as filters in food and beverage processing industries, especially for sensitive sectors such as protein separation and concentration, juice clarification, alcoholic beverages, drinking water, and several other environmental problems, especially wastewater treatment. These membranes present very good mechanical properties, high permeabilities, and their cost can be moderated by the use of low-cost materials. Ceramic membranes are still under development, especially the ones that are made from clays and ash. The development of new ceramic membrane generation for environmental and food applications is expected.