1 Introduction
Cationic gemini surfactants are compounds that are made of two single alkylammonium monomeric salts linked by a spacer. The nature of the spacer can be very different. It can be a flexible polymethylene chain or a rigid benzene. This linker can also contain some substituted atoms such as oxygen or nitrogen [1–3]. Because of the presence of the spacer that precisely defines the distance between positive nitrogen atoms, the number of gemini molecules that form micelles is much smaller than monomeric ones. Hence, gemini surfactants have much lower critical micelle concentration (cmc) values in comparison to monomeric alkylammonium salts. In addition, the extension of the carbon chain further reduces the cmc value, which is why these compounds significantly reduce surface tension and show an excellent wetting, emulsification, dispersion, and solubilization ability [4–9]. Thanks to the presence of two positively charged nitrogen atoms and a long hydrocarbon chain, gemini surfactants strongly adsorb onto the negatively charged cell wall, which results in high biocidal activity [10–14]. Gemini surfactants also show lower toxicity to aquatic organisms [15–17]. These extraordinary properties of the gemini surfactant are the basis for their potential for very wide applications in personal care products, additives for paints and coatings, biocides, smart materials, drug and gene delivery agents, organic synthesis, phase transfer and micellar catalysts, capping agents for the synthesis of nanoparticles, supramolecular solvents, microemulsion stabilizers, textile and paper processing, enhanced oil recovery, additives for petroleum, and environmental protection [7,18–24]. The low cmc of gemini surfactants implies huge savings in industrial applications [25]. From an ecological point of view, the use of gemini surfactants also entails significant benefits, which are in line with the greenolution idea in chemistry [5,19].
Thanks to the presence of two positively charged nitrogen atoms and a large molecular surface, gemini surfactants are also very efficient corrosion inhibitors. To strengthen the electrostatic adsorption of ammonium cations on a metal surface, which is a key parameter in the inhibition of corrosion, heteroatoms (O, S, N, or P) and π-electron systems can be introduced into the gemini surfactant structure to increase chemical adsorption.
In this study, we investigated the relationship between the alkyl chain length and cmc for gemini surfactants containing an oxygen atom in the spacer, that is, bis(N-alkyl-N,N-dimethylethylammonium)ether dibromides, as potential corrosion inhibitors.
2 Experimental section
2.1 Materials used
Bis(2-bromoethyl)ether (Sage Chemicals, ≥99%), N-butyl-N,N-dimethylamine (Sigma–Aldrich, 99%), N-hexyl-N,N-dimethylamine (Sigma–Aldrich, 98%), N-octyl-N,N-dimethylamine (Sigma–Aldrich, 95%), N-decyl-N,N-dimethylamine (Sigma–Aldrich, ≥90%), N-dodecyl-N,N-dimethylamine (Sigma–Aldrich, 97%), N-tetradecyl-N,N-dimethylamine (Sigma–Aldrich, ≥95%), N-hexadecyl-N,N-dimethylamine (Sigma–Aldrich, ≥95%), N-octadecyl-N,N-dimethylamine (TCI, >90%), acetonitrile (VWR Chemicals, for HPLC), methanol (Avantor, for HPLC), phosphorus pentoxide (VWR Chemicals, technical grade).
2.2 Synthesis of bis(N-alkyl-N,N-dimethylethylammonium)ether dibromides
Most of the bis(N-alkyl-N,N-dimethylethylammonium)ether dibromides, except for bis(N-octadecyl-N,N-dimethylethylammonium)ether dibromide, were synthesized analogously to the method described previously [26]; 1 equiv of bis(2-bromoethyl)ether was mixed with 2 equiv of suitable N-alkyl-N,N-dimethylamine (N-butyl-N,N-dimethylamine for 4-O-4; N-hexyl-N,N-dimethylamine for 6-O-6; N-octyl-N,N-dimethylamine for 8-O-8; N-decyl-N,N-dimethylamine for 10-O-10; N-docecyl-N,N-dimethylamine for 12-O-12; N-tetradecyl-N,N-dimethylamine for 14-O-14; and N-hexadecyl-N,N-dimethylamine for 16-O-16). The reactions were carried out without solvent, at room temperature, with stirring using a magnetic stirrer until the reaction mixture solidified (approx. 2 h). The crude products were crystallized from acetonitrile with a small addition of methanol and dried over P4O10 in a dryer. Bis(N-octadecyl-N,N-dimethylethylammonium)ether dibromide was obtained according to Ref. [27]; 1 equiv of bis(2-bromoethyl)ether and 2 equiv of N-octadecyl-N,N-dimethylamine was refluxed in acetonitrile until the reaction mixture solidified. Then, the crude product was crystallized from a mixture of acetonitrile:methanol (10:1) and dried over P4O10 in a dryer. The general formula and abbreviations for the alkylammonium gemini surfactants obtained are presented in Fig. 1.
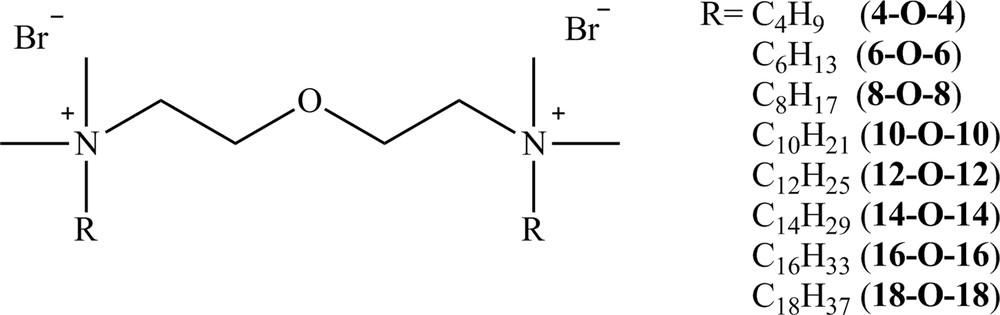
General formula and abbreviations for the as-obtained bis(N-alkyl-N,N-dimethylethylammonium)ether dibromides.
4-O-4: mp 224–225 °C; elemental analysis (%) calcd for C16H38Br2N2O: C 44.25, H 8.82, N 6.45; found C 44.42, H 8.88, N 6.30; 1H NMR (403 MHz, CDCl3): δ 4.14 (d, J = 4.0 Hz, 4H, –OCH2), 3.83 (dd, J = 5.4, 3.7 Hz, 4H, –N+CH2CH2O–), 3.53 (ddd, J = 12.0, 7.5, 3.8 Hz, 4H, –N+CH2–), 3.29 (s, 12H, –N+(CH3)2), 1.74 (m, 4H, –N+CH2CH2–), 1.44 (dd, J = 14.9, 7.4 Hz, 4H, –CH2CH2CH3), 1.01 (t, J = 6.6 Hz, 6H, –CH2CH3); 13C NMR (101 MHz, CDCl3): δ 65.61 (–N+CH2CH2O–), 64.42 (–OCH2–), 63.98 (–N+CH2–), 51.36 (–N+CH3), 24.42 (–N+CH2CH2–), 19.39 (–CH2CH3), 13.41(–CH2CH3); ESI-MS (MeOH): m/z (%) 353 (10) [M–Br]+; 259 (100) [M–2Br–CH3]+; 217 (33) [M–2Br]2+.
6-O-6: mp 220–222 °C; elemental analysis (%) calcd for C20H46Br2N2O: C 48.98, H 9.45, N 5.71; found C 48.82, H 9.50, N 5.50; 1H NMR (403 MHz, CDCl3): δ 4.18 (d, J = 4.2 Hz, 4H, –OCH2), 3.95–3.73 (m, 4H, –N+CH2CH2O–), 3.59-3.44 (m, 4H, –N+CH2–), 3.32 (s, 12H, –N+(CH3)2), 1.86–1.64 (m, 4H, –N+CH2CH2–), 1.50–1.24 (m, 12H, –CH2CH2CH2CH3) 0.90 (t, J = 5.3 Hz, 6H, –CH2CH3); 13C NMR (101 MHz, CDCl3): δ 65.88 (–N+CH2CH2O–), 64.47 (–OCH2–), 63.96 (–N+CH2–), 51.40 (–N+CH3), 31.10 (–CH2CH2CH3), 25.70, 22.55 (–N+CH2CH2–), 22.20 (–CH2CH3), 13.69 (–CH2CH3); ESI-MS (MeOH): m/z (%) 410 (40) [M–Br]+; 315 (100) [M–2Br–CH3]+; 245 (60) [M–2Br]2+.
8-O-8: mp 242–243 °C; elemental analysis (%) calcd for C24H54Br2N2O: C 52.75, H 9.96, N 5.13; found C 51.96, H 9.91, N 4.73; 1H NMR (403 MHz, CDCl3): δ 4.35 (bs, 4H, –OCH2), 4.04 (bs, 4H, N+CH2CH2O–), 3.74–3.57 (m, 4H, –N+CH2–), 3.46 (s, 12H, –N+(CH3)2), 1.73 (bs, 4H, –N+CH2CH2–), 1.47–1.19 (m, 20H, –CH2(CH2)5CH3), 0.88 (t, J = 6.9 Hz, 6H, –CH2CH3); 13C NMR (101 MHz, CDCl3): δ 65.76 (–N+CH2CH2O–), 64.56 (–OCH2–), 63.89 (–N+CH2–), 51.58 (–N+CH3), 31.57 (–CH2CH2CH3), 29.18, 28.98, 26.23, 22.83 (–N+CH2CH2–), 22.48 (–CH2CH3), 13.97 (–CH2CH3); ESI-MS (MeOH): m/z (%) 465 (20) [M–Br]+; 371 (100) [M–2Br–CH3]+; 273 (65) [M–2Br]2+.
10-O-10: mp 249–250 °C; elemental analysis (%) calcd for C28H62Br2N2O: C 55.81, H 10.37, N 4.65; found C 56.49, H 10.62, N 4.33; 1H NMR (403 MHz, CDCl3) δ 4.36 (bs, 4H, –OCH2) 4.04 (bs, 4H, N+CH2CH2O–) 3.73–3.58 (m, 4H, –N+CH2–), 3.46 (s, 12H, –N+(CH3)2–), 1.73 (bs, 4H, –N+CH2CH2–), 1.48–1.14 (m, 28H, –CH2(CH2)7CH3), 0.88 (t, J = 6.9 Hz, 6H, –CH2CH3); 13C NMR (101 MHz, CDCl3): δ 65.83 (–N+CH2CH2O–), 64.60 (–OCH2–), 63.96 (–N+CH2–), 51.61 (–N+CH3), 31.74 (–CH2CH2CH3), 29.38, 29.35, 29.25, 29.15, 26.28 (–N+(CH2)2CH2–), 22.87 (–N+CH2CH2–), 22.55 (–CH2CH3), 13.99 (–CH2CH3); ESI-MS (MeOH): m/z (%) 523 (100) [M–Br]+; 428 (53) [M–2Br–CH3]+; 338 (50) [M–2Br–CH3–2C3H6]+; 273 (38) [M–2Br]2+.
12-O-12: mp 248–249 °C; elemental analysis (%) calcd for C32H70Br2N2O: C 58.35, H 10.71, N 4.25; found C 58.48, H 11.19, N 4.15; 1H NMR (403 MHz, CDCl3) δ 4.35 (bs, 4H, –OCH2), 4.04 (bs, 4H, N+CH2CH2O–), 3.63 (dd, J = 17.3, 9.2 Hz, 4H, –N+CH2–) 3.46 (s, 12H, –N+(CH3)2–), 1.73 (bs, 4H, –N+CH2CH2–), 1.45–1.17 (m, 36H, –CH2(CH2)9CH3), 0.88 (t, J = 6.9 Hz, 6H, –CH2CH3); 13C NMR (101 MHz, CDCl3): δ 65.81 (–N+CH2CH2O–), 64.57 (–OCH2–), 63.94 (–N+CH2–), 51.60 (–N+CH3), 31.82 (–CH2CH2CH3), 29.53, 29.46, 29.38, 29.28, 29.26, 26.27 (–N+(CH2)2CH2–), 22.87 (–N+CH2CH2–), 22.60 (–CH2CH3), 14.05 (–CH2CH3); ESI-MS (MeOH): m/z (%) 579 (10) [M–Br]+; 534 (88) [M–2Br–CH3–C2H4]+; 484 (52) [M–2Br–CH3]+; 329 (68) [M–2Br–CH3–C5H10–C6H12]+; 315 (88) [M–2Br–CH3–2C6H12]+, 249 (75) [M–2Br]2+; 236 (100) [M–2Br–C2H4]2+.
14-O-14: mp 246–247 °C; elemental analysis (%) calcd for C36H78Br2N2O: C 60.49, H 11.00, N 3.92; found C 60.48, H 11.41, N 3.70.1H NMR (403 MHz, CDCl3) δ 4.36 (bs, 4H, –OCH2), 4.04 (bs, 4H, N+CH2CH2O–), 3.71–3.59 (m, 4H, –N+CH2–), 3.46 (s, 12H, –N+(CH3)2), 1.73 (bs, 4H, –N+CH2CH2–), 1.49–1.08 (m, 44H, –CH2(CH2)11CH3), 0.88 (t, J = 6.8 Hz, 6H, –CH2CH3); 13C NMR (101 MHz, CDCl3): δ 65.87 (–N+CH2CH2O–), 64.61(–OCH2–), 64.00 (–N+CH2–), 51.64 (–N+CH3), 31.85 (–CH2CH2CH3), 29.62, 29.60, 29.59, 29.56, 29.48, 29.40, 29.29, 26.31 (–N+(CH2)2CH2–), 22.90 (–N+CH2CH2–), 22.61(–CH2CH3), 14.04 (–CH2CH3); ESI-MS (MeOH): m/z (%) 633 (7) [M–Br]+; 277 (100) [M–2Br]2+; 242 (28) [M–2Br–C2H4–C3H6]2+.
16-O-16: mp 240–242 °C; elemental analysis (%) calcd for C40H86Br2N2O: C 62.32, H 11.24, N 3.63; found C 61.41, H 11.68, N 3.20; 1H NMR (403 MHz, CDCl3) δ 4.36 (bs, 4H, –OCH2), 4.05 (bs, 4H, N+CH2CH2O–), 3.67–3.62 (m, 4H, –N+CH2–), 3.46 (s, 12H, –N+(CH3)2), 1.73 (bs, 4H, –N+CH2CH2–), 1.47–1.10 (m, 52H, –CH2(CH2)13CH3), 0.88 (t, J = 6.9 Hz, 6H, –CH2CH3); 13C NMR (101 MHz, CDCl3): δ 65.92 (–N+CH2CH2O–), 64.59 (–OCH2–), 64.03 (–N+CH2–), 51.57 (–N+CH3), 31.82 (–CH2CH2CH3), 29.61, 29.58, 29.56, 29.54, 29.45, 29.37, 29.25, 26.25 (–N+(CH2)2CH2–), 22.83 (–N+CH2CH2–), 22.58 (–CH2CH3), 14.00 (–CH2CH3); ESI-MS (MeOH): m/z (%) 691 (2) [M–Br]+; 305 (100) [M–2Br]2+.
18-O-18: mp 236–239 °C; elemental analysis (%) calcd for C44H94Br2N2O: C 63.90, H 11.46, N 3.39; found C 64.68, H 12.14, N 3.55; 1H NMR (403 MHz, CDCl3) δ 4.16 (bs, 4H, –OCH2), 3.84 (d, J = 4.2 Hz, 4H, N+CH2CH2O–), 3.57–3.42 (m, 4H, –N+CH2–), 3.29 (s, 12H, –N+(CH3)2–), 1.72 (bs, 4H, –N+CH2CH2–), 1.46–1.03 (m, 60H, –CH2(CH2)15CH3), 0.88 (t, J = 6.7 Hz, 6H, –CH2CH3); 13C NMR (101 MHz, CDCl3): δ 65.65 (–N+CH2CH2O–), 64.56 (–OCH2–), 63.76 (–N+CH2–), 51.37 (–N+CH3–), 31.84 (–CH2CH2CH3), 29.63, 29.61, 29.58, 29.52, 29.48, 29.40, 29.28, 26.31(–N+(CH2)2CH2–), 22.85 (–N+CH2CH2–), 22.60 (–CH2CH3), 14.03 (–CH2CH3); ESI-MS (MeOH): m/z (%) 748 (12) [M–Br]+; 333 (100) [M–2Br]2+.
Fourier transform infrared (FTIR) spectra for the alkylammonium gemini surfactants show typical bands for the stretching asymmetric (νas) and symmetric (νs) vibrations of methyl and methylene groups at 2980–2850 cm−1, as well as bands for the deformation vibration (δ) of these groups at 1470–1355 cm−1 and bands for the vibration of C–O bonds at 1150–1070 cm−1. Increasing the length of the hydrocarbon substituent does not affect the shape of the FTIR spectrum.
2.3 Analytical methods
2.3.1 1H and 13C NMR spectroscopy
All NMR spectra for the synthesized products were measured using a Varian model VNMR-S 400 MHz operating at 403 and 101 MHz for 1H and 13C, respectively. The 13C and 1H chemical shifts were measured in CDCl3 with TMS as an internal standard.
2.3.2 FTIR spectroscopy
The FTIR (KBr) spectra for the synthesized products were measured using an FTIR Spectrometer JASCO model FT/IR-4700 type A.
2.3.3 Electron spray ionization mass spectrometry
The electron spray ionization (ESI) mass spectra were recorded using a ZQ Waters Mass Spectrometer. The sample solutions were prepared in methanol. The ESI-MS spectra were recorded at a cone voltage of 30 V.
2.3.4 Elemental analysis
The measurements were carried out on an FLASH 2000 elemental analyzer with a thermal conductivity detector.
2.4 Conductivity measurement
The cmc values were determined conductometrically using a Conductivity Meter Elmetron CC-505. The apparatus was calibrated using a standard (147 μS/cm in 298.15 K). All solutions were prepared using double-distilled water. Conductivity measurements were performed at temperature of 298.15 K. The conductometric titration was repeated at least three times for each surfactant.
2.5 Semiempirical calculations
PM5 (parameterized model number 5) calculations were performed using the WinMopac 2003 program.
3 Results and discussion
3.1 Synthesis
Bis(N-alkyl-N,N-dimethylethylammonium)ether dibromides, except for bis(N-octadecyl-N,N-dimethylethylammonium)ether dibromide, were synthesized at room temperature, without solvent in a relatively short time. The method of synthesis used allows one to obtain products with very good yields without waste. This kind of product preparation is in accordance with the greenolution idea. Bis(N-octadecyl-N,N-dimethylethylammonium)ether dibromide was obtained by the use of classical nucleophilic substitution in acetonitrile with good yield. The purities for all of the gemini surfactants obtained were confirmed by 1H NMR, 13C NMR, FTIR, and ESI mass spectrometry and elemental analysis.
3.2 Aggregation behavior
The cmc values for all synthesized bis(N-alkyl-N,N-dimethylethylammonium)ether dibromides (m-O-m) were obtained by conductometric titration at 298.15 K.
The relationship of the specific conductivity (κ) on the concentration of surfactants creates two lines with different slopes. The straight line with higher inclination reflects behavior before micellization, whereas the line with a smaller slope reflects the process of micellization. The intersection of lines obtained as a result of linear regression defines cmc (Fig. 2) [6,28,29].
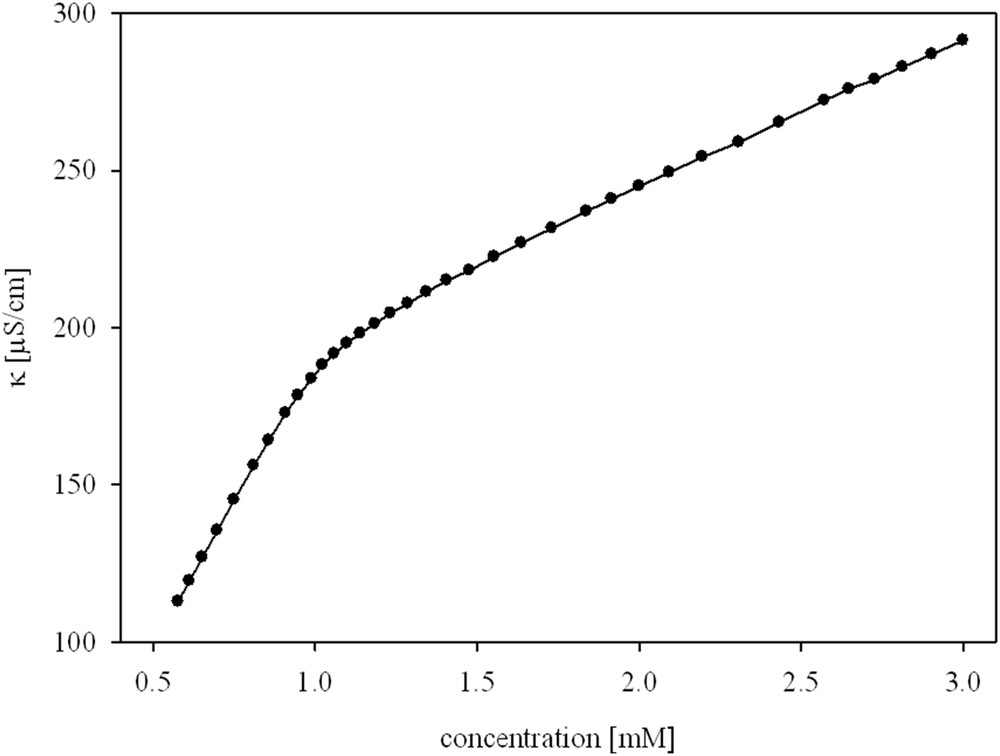
A plot of specific conductivity (κ) for the aqueous solution of 12-O-12 versus its concentration.
The values for the straight line slopes depicting the micelle and monomer regions, respectively, allow one to estimate the degree of dissociation for the micelles (degree of ionization) (α) and the degree of counterion association for the micelle (counterion binding parameter) (β) [29,30]. These parameters reflect the ability of the counterion binding on the micelles. The bigger the α, the smaller the β [31,32].
The micellization process for surfactants is thermodynamically controlled and can be expressed by the free Gibbs energy of micellization (ΔGomic) [33]. The experimental values for cmc, α, β, and ΔGomic for the investigated gemini surfactants are given in Table 1. The negative values of ΔGomic clearly show that the micellization process is spontaneous. The longer the alkyl chain, the greater the tendency to form micelles.
Aggregation parameters for the bis(N-alkyl-N,N-dimethylethylammonium)ether dibromides.
Surfactant | cmc (mM) | α | β | ΔGomic (kJ/mol) |
4-O-4 | 158.5 ± 3.5 | 0.69 | 0.31 | −11.8 |
6-O-6 | 81.1 ± 2.0 | 0.66 | 0.34 | −12.2 |
8-O-8 | 21.1 ± 0.9 | 0.66 | 0.34 | −17.8 |
10-O-10 | 6.59 ± 0.16 | 0.49 | 0.51 | −26.9 |
12-O-12 | 1.047 ± 0.006 | 0.29 | 0.71 | −42.9 |
14-O-14 | 0.201 ± 0.002 | 0.42 | 0.58 | −47.3 |
16-O-16 | 0.032 ± 0.002 | 0.56 | 0.44 | −49.9 |
18-O-18 | 0.033 ± 0.001 | 0.42 | 0.58 | −56.9 |
The cmc values for the tested gemini alkylammonium surfactants are significantly lower than the values for the monomeric counterparts (Fig. 3) [34]. There are many publications describing the relationship between the alkyl chain length and cmc for gemini surfactants with different spacers, such as the ethylene group [35–38], three methylene group [39], tetramethylene group [40], hexamethylene group [35,41,42], octamethylene group [43], spacers functionalized with hydroxy or amino groups [39,42,44], aromatic spacers [2,45], and spacers with an unsaturated bond [40]. To our knowledge, there is no systematic report concerning cmc values for alkylammonium gemini bromides with an ether group in the spacers. There are only a few data for 12-O-12 [46–50] and 16-O-16 [51]. Our results are in good correlation with those published by Wettig et al. [50] and Wang et al. [49], where for gemini 12-O-12, cmc, α, and ΔGomic are 1.02 mM, 0.25, −42.7 kJ/mol [50], and 1.35 mM, 0.22, −47.34 kJ/mol [49], respectively.
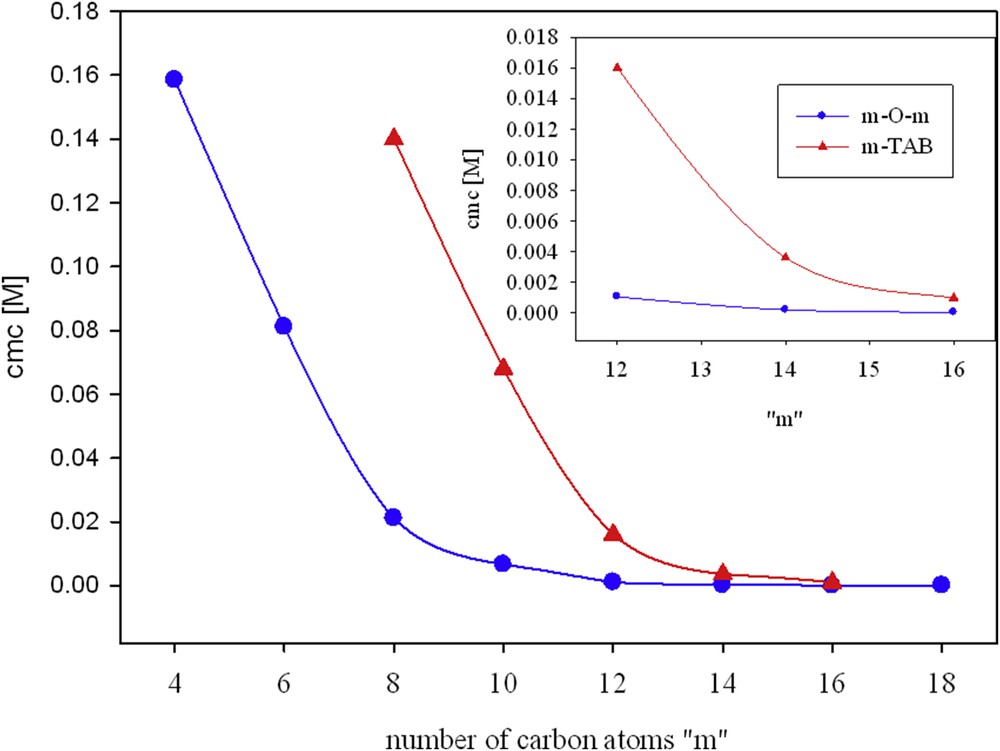
Variation in cmc of cationic surfactants with alkyl chain carbon atoms “m”: (Image 1) tested gemini surfactants “m-O-m” (Image 1) N-alkyl-N,N,N-trimethylammonium bromides (m-TAB) [34].
The elongation of the alkyl chain in the surfactant, especially in the gemini surfactant, causes a decrease in the cmc values. This is because of less large elements being needed to create the micelle structure [2,36,40–42,45].
From comparing the cmc values for the m-O-m surfactants with conventional m-s-m compounds (Table 2.), it follows that the modification of the surfactant spacer by the introduction of an oxygen atom causes only slight changes in the values of cmc. In some cases, m-O-m surfactants have lower cmc than the corresponding m-6-m. This is probably because the presence of a hydrophilic group in the linker reduces the Coulombic repulsion between the head groups and gemini surfactants show a better-packed micelle [4,49]. According to Li et al.[41], the relationship between log cmc and the number of carbon atoms in a hydrophilic chain decreases linearly (see Fig. 4).
The cmc values for cationic gemini surfactants with different spacers and with increasing hydrocarbon substituent length.
m | cmc (mM) | |||||
m-O-ma | m-2-mb | m-3-mc | m-4-md | m-6-me | m-8-mf | |
8 | 21.1 | 55 | – | 56 | 25 | 50 |
10 | 6.59 | 5.2 | – | 8.78 | 8.2 | 5.8 |
12 | 1.047 | 1.06 | 0.98 | 0.93 | 1.2 | 0.80 |
14 | 0.201 | – | 0.11 | 0.135 | 0.15 | 0.018 |
16 | 0.032 | 0.04 | 0.015 | 0.0422 | 0.048 | 0.038 |
18 | 0.033 | – | – | 0.0419 | – | 0.0115 |
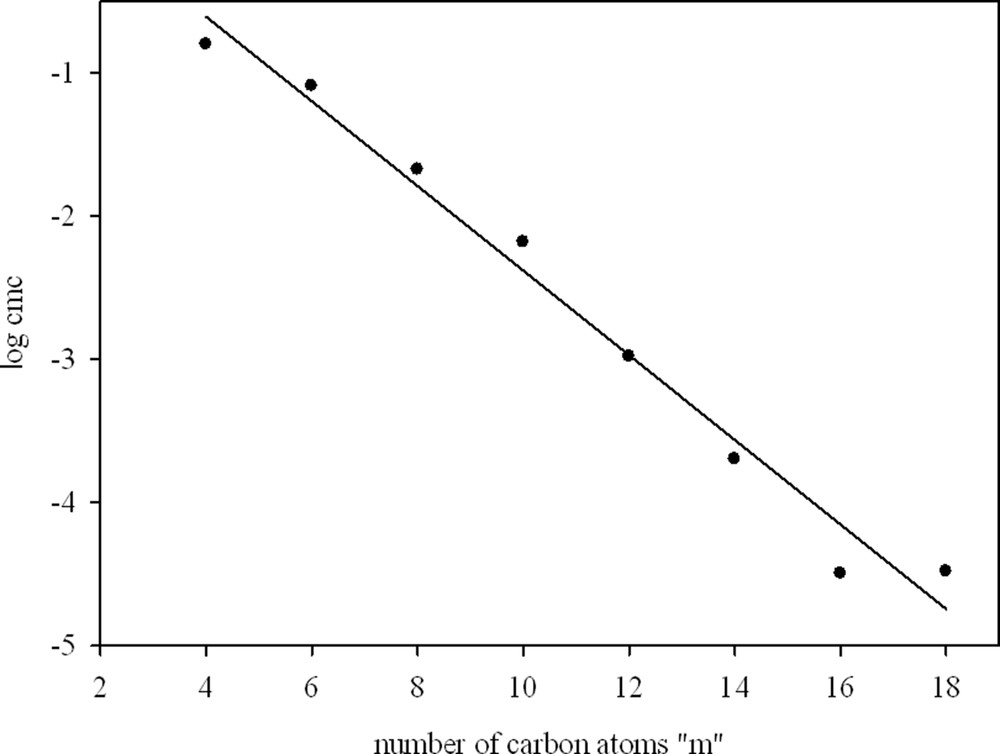
Variation in log cmc with the number of carbon atoms in the hydrocarbon chain for m-O-m gemini surfactants.
Changes in the spacer length also involve slight changes in cmc values. Between the cmc of geminis with short (s = 2) and long (s = 16) spacers, there is a difference of one order of magnitude [52,53]. The cmc values of cationic gemini surfactants are also affected by the type of anion. Conversion of the chloride ion to bromide slightly reduces cmc. For bromides, 12-O-12 and 18-O-18 cmc values are 1.047 and 0.033 mM, whereas for analogous chlorides these values were reported to be 1.72 and 0.044 mM, respectively [54].
The dissociation degree for the micelles (α) is in general higher than that reported for the monomeric counterpart [35]. As seen in Table 1, α initially decreases with increasing chain length, reaching the lowest value for 12-O-12, and then increases with the elongation of the alkyl chain. A similar relationship can also be observed for m-6-m geminis [35]. The small value of α is caused by the stronger binding of the counterion to the micelles, suggesting closer packing of the head groups and higher surface charge density at the micelle–solution interface [55].
3.3 Semiempirical calculations
PM5 semiempirical calculations were performed using the WinMopac 2003 program [56–58]. A representative compound, 8-0-8, is shown in Fig. 5. The final heat of formation (HOF) for all of the compounds is presented in Table 3. The data from Table 3 clearly show that the values for the HOF decrease with increasing length of the alkyl chain. The lowest HOF values for the bis-(N-alkyl-N,N-dimethylethylammonium)ether dibromides are observed for the longest alkyl chain. A similar relationship was observed in our previous work [16].

Molecular model of 8-O-8 calculated by the PM5 method.
HOF (kcal/mol) for the gemini surfactants.
Compound | HOF (kcal/mol) |
4-O-4 | −63.7817 |
6-O-6 | −86.0340 |
8-O-8 | −122.6361 |
10-O-10 | −144.8594 |
12-O-12 | −166.8561 |
14-O-14 | −189.4630 |
16-O-16 | −211.1746 |
18-O-18 | −233.5934 |
4 Conclusions
A series of eight bis(N-alkyl-N,N-dimethylethylammonium)ether dibromides were synthesized without solvent, according to the greenolution idea. The chemical structures of the novel compounds were confirmed by 1H and 13C NMR, FTIR, and ESI-MS. The cmc values were determined by conductivity measurements. The cmc values decrease gradually as the alkyl chain length increases. It was demonstrated that the length of the hydrocarbon moiety governs the cmc of the geminis. It was also shown that geminis with the longest alkyl chain have the lowest free Gibbs energy for micellization, and the lowest HOF, that is, they are the most stable.
Acknowledgments
This work was supported by the National Center for Research and Development (Poland; TANGO1/266340/NCBR/2015).