1 Introduction
Numerous papers have been devoted to the study of metal insertion into porphyrins 〚1〛 and the mechanism of this reaction has been the subject of a lot of controversial debate 〚2–5〛. The rate of metal insertion into planar porphyrins is very much slower than into open chain ligands; this is because planar porphyrins must largely deform in order to accept the incoming metal ions. Thus, some distorted porphyrins have been synthesised by introducing a methyl group on the pyrrole nitrogen 〚6, 7〛. In this case, the incorporation rate of metal ions is greatly enhanced. Similarly, when β pyrrolic positions are occupied by voluminous substituents like bromine atoms or phenyl groups 〚8, 9〛, the porphyrin ring is subjected to some distortion and may adopt a saddle shape. In this latter case, it was demonstrated that such a β substituted porphyrin can form a stable complex with lithium ions, contrary to planar porphyrins that cannot form stable dilithium salts 〚10, 11〛. However, these lithium complexes could very easily lead to divalent cationic porphyrin, but no kinetic studies of this reaction have been reported yet 〚9, 12〛.
On the other hand, it was recently demonstrated 〚13, 14〛 that the deformation of the planar mesoporphyrin IX induced by an antibody increased the rate of incorporation of Zn2+ ions. Thus, it was anticipated that, if the lithium ions were to accommodate with a distorted porphyrin, they would be able to play the role of the antibody and form a transient intermediate that could enhance the rate of metallation of a planar porphyrin by a divalent cation.
For the purpose of this study, we chose to examine the kinetics of metallation of the planar tetratolylporphyrin by copper ions in an aprotic solvent, in the presence of a soluble lithium salt such as LiClO4.
2 Experimental
2.1 Reagents and solvents
Dichloromethane (Prolabo) was distilled twice on calcium hydride. Tetratolylporphyrin (H2TTP) was purified by preparative TLC (silica) using dichloromethane as eluent. Copper acetyl acetonate (Aldrich) was used as received. LiClO4 (Acros) was used as received.
2.2 Measurements
The reactions were monitored on a Hewlett Packard 845LA diode array UV–visible spectrophotometer with a thermostated cell compartment maintained within ±0.1 °C. The reactions were run under pseudo-first-order kinetic conditions at 30 °C, with the metal ion at a large excess over the free-base porphyrin concentration.
The formation of metalloporphyrin was carried out in 1 cm quartz cells by mixing a porphyrin solution and a metal ion solution pre-equilibrated at the reaction temperature. Then the absorbances at 516 nm were automatically recorded at 10 s intervals over a period of 600 s.
Pseudo-first-order plots showed good linearities and conditional first order rate constants were obtained from the slopes of such straight lines.
3 Results and discussion
3.1 Kinetics of metal ion incorporation into H2TTP
The kinetics of the reaction of H2TTP with copper II acetyl acetonate in CH2Cl2 at 30 °C was studied. The rate for the formation of metalled porphyrin is of first order with respect to porphyrin.
It is known 〚15, 16〛 that in non-polar solvents like CH2Cl2, copper acetyl acetonate undergoes dimerisation and that the concentration of the available monomer, which is the only reactant species, is given by:
When plotted against 〚Cu(acac)2〛m, the values of ka give a straight line (R2 = 0.945) (Fig. 1) of slope ki. Then the general kinetics of the metallation in CH2Cl2 is:

TTP in CH2Cl2 without Li+.
3.2 Kinetics of metal ion incorporation in the presence of lithium perchlorate
Before studying the effect of lithium salt on the kinetics of metallation, we tested that this salt did not affect the spectrum of H2TTP for the Q bands considered in the absorbance measurements. So, in a first series of experiments we studied the rate of metallation at constant 〚Cu(acac)2〛m = 3.8 × 10–4 mol L–1 for different lithium concentrations.
At a constant concentration of 〚Cu(acac)2〛m, ka increased linearly (R2 = 0.973) with Li+ concentration (Fig. 2).
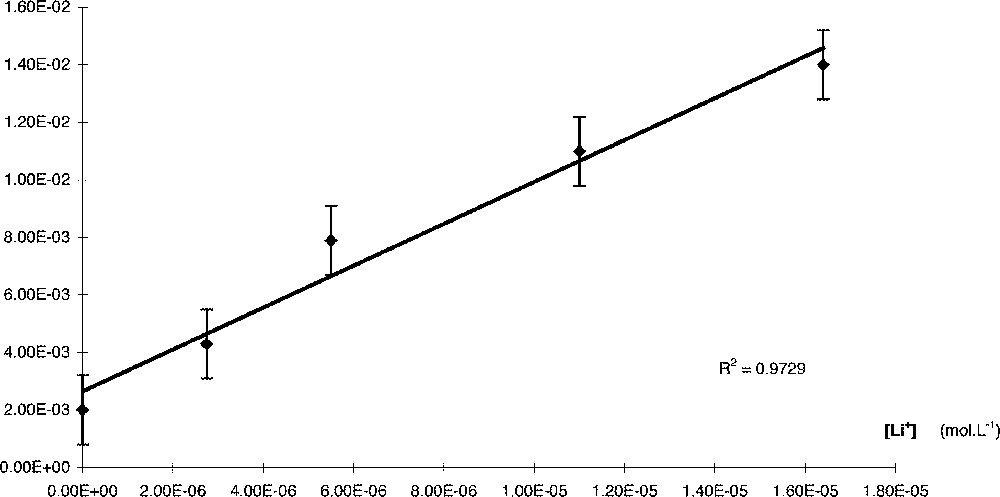
Kobs = f(Li+).
If we assume that lithium (Li) and porphyrin (P) species are in fast equilibrium with the complex (PLi〛):
If we include to this mechanism the non-catalytic step:
kobs is linear when plotted again 〚Li〛 (Fig. 2). The slope of this straight line gives kcat:
In a second series of experiments, kinetics were conducted at various concentrations of Cu in CH2Cl2 saturated with LiClO4 (C = 1.22 × 10–3 mol L–1). In this case, kobs plotted against 〚Cu〛 (Fig. 3) gives a straight line (R2 = 0.950) of equation:

TTP in a saturated CH2Cl2 solution of Li+.
This curve shows that in these conditions the reaction is very fast and cannot be performed at high concentrations of 〚Cu〛: in the presence of lithium, the value of kobs is 535 fold that without catalyst.
In summary, we have found that, in aprotic media, metallation of the free-base form of tetratolylporphyrin can be enhanced in presence of Li salts. Such behaviour confirms the effect of the distortion of the macrocycle on the kinetics of metallation. Besides the fundamental interest, we have established a new method for fast metallation of porphyrins. Metal studies on the incorporation of other metals are under investigation in our laboratory.
Acknowledgements
The authors are grateful to Prof. M. Guilloton for his help in the preparation of this manuscript.