1 Introduction
Since the pioneering work of Schilt [1], molecules deriving from 1,2,4-triazine or 1,3,5-triazine have been tested for their complexing properties, as well as their use as potent anticancer drugs [2–3].
The determination of several metal ions could be performed by complexation with various organic ligands. The chelating properties of 1,10-phenanthroline toward iron(II) are well known. Recently, Safavi et al. [4] have described the use of 1,10-phenanthroline to determine simultaneously Fe(II) and Fe(III) after reduction with Co(II) with cetyl trimethyl ammonium bromide (CTAB) in micellar conditions. The azo derivatives, e.g. 1-(2-pyridylazo)-2-naphtol (PAN), are sensitive and selective derivatives used to determine spectrophotometrically Ga(III) or In(III) ions and these determinations are easier when using micellar medium obtained with cetyltrimethylammonium bromide (CTMB) as surfactant [5]. In the presence of cetylpyridinium chloride (CPC), pyrimidine azo dyes enable the simultaneous determination of thorium and rare earth metals [6]. In many determinations, the addition of surfactants enhances the sensitivity of titration of many metal cations, even in pharmaceutical products [7].
Many triazines could be complexing agents. 2,4,6-tris(2′-pyridyl)-1,3,5-triazine is used to determine trace amounts of ruthenium(III) after extraction [8]. This complex is used in iron selective electrodes for potentiometric measurements in pharmaceuticals, but this method is not suitable in the presence of ferric ions [9]. Krishnamurti and Huang described a spectrophotometric method for the determination of Fe(II) by complexation with 2,4,6-tris(2′-pyridyl)-1,3,5-triazine in the presence of large amounts (up to 800 mg l–1) of Fe(III) at pH 2.0–2.4 [10]. In this case, the Fe(III) ions are masked by complexing with fluoride before development of the violet Fe(II) triazine complex. The titration is performed spectrophotometrically. In the same way, Berger et al. have described the use of bis(2,2′-bipyridine)(2,4,6-tris(2-pyridyl)triazine) ruthenium(II) complex [11]. The sodium 3-(2-pyridyl)-5,6-diphenyl-1,2,4-triazine-4′,4"-disulphonate (ferrozine) formed a chelate with Fe(II) at pH 5.5 and this complex is useful for determination of iron(II) in the range 0.1–6.0 μg ml–1 by spectrophotometric flow injection analysis [12].
In recent years, interest in surfactants has greatly increased, because they provide a reaction medium in which the sensitivity and the selectivity of numerous reactions are improved. For instance, the complex species formed are generally more stable than those obtained in the absence of micelles [13,14].
A cationic surfactant like cetyl pyridinium chloride enhances the sensitivity of the titration of ferric ions in the presence of thiocyanate ions [15]. In the present work, we have used 2,4,6-trimorpholino 1,3,5-triazine (KD) (Fig. 1 ) as ligand to complex several metal cations. We describe here the results obtained with Fe(III) and we show that the determination of Fe(III) is more accurate when using CPC. In addition this technique allows us to titrate Fe(III) even in the presence of large amounts of Fe(II). This method is simpler than many iron(II) and/or iron(III) titration techniques, using substituted triazines [16 (and references cited therein)].
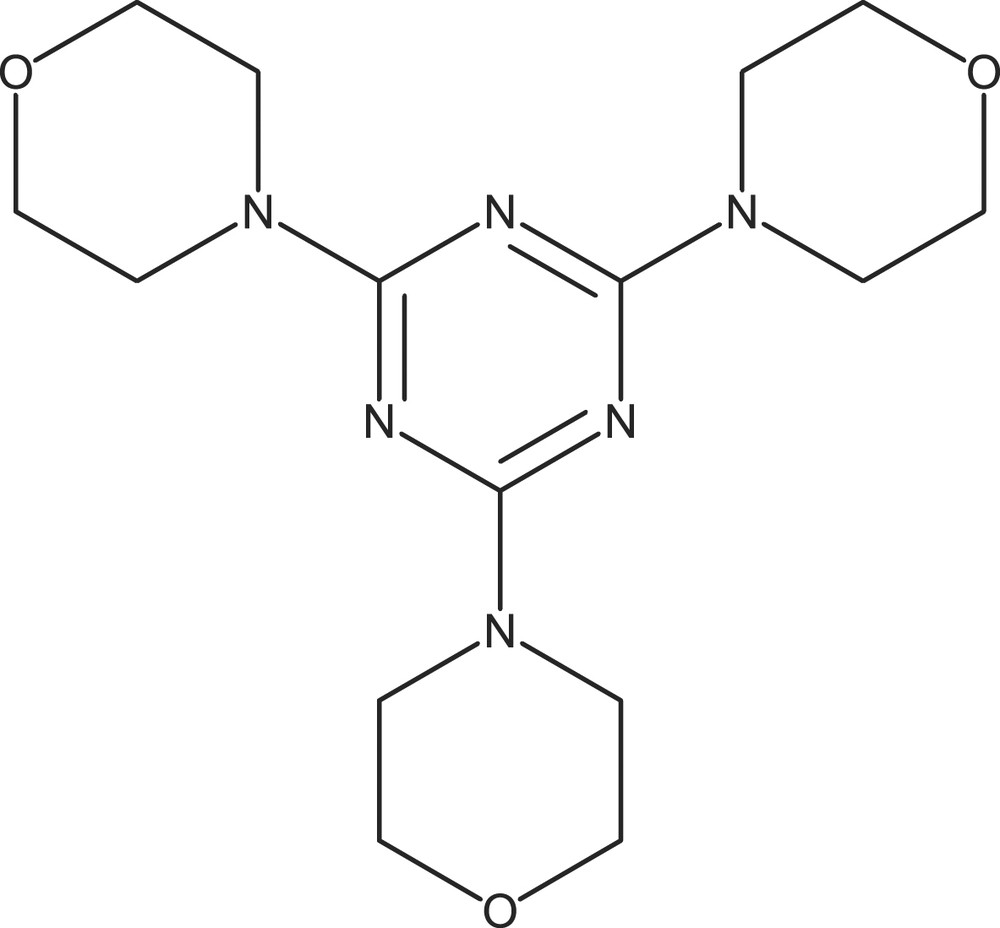
Chemical structure of KD.
KD could complex a large number of metal cations, like Al(III), Fe(II), Fe(III), Co(II), Ni(II), Cu(II), Zn(II), Ce(III), and Pb(II). However, only Fe(III) solutions showed an absorption spectrum in the UV region. The absorbance of the ferric solutions was enhanced when using benzyl dimethyl tetradecyl ammonium chloride (BDTA) or CPC as surfactants.
2 Experimental
2.1 Apparatus
The UV-visible spectra were recorded with UV-1600 PC Shimadzu double beam UV-visible spectrophotometer, with 1-cm optical path quartz cells at 25.0 ± 0.5 °C. pH were measured with an ISKRA MA pH-meter.
2.2 Reagents
In all cases, the solvent was a water-ethanol mixture (50:50; v/v) starting from bi-distilled water and anhydrous ethanol p.a. (Fluka).
2,4,6- trimorpholino 1,3,5-triazine (KD) was synthesised by trimerisation of N-cyano morpholine (Aldrich) according to the procedure described by Cariou et al. [17] and a stock solution (10–3mol l–1) was then prepared. Fe(III) solution (10,0 g l–1) was made by weighing 72 g of ferric nitrate nonahydrate Fe(NO3)3 9 H2O (Riedel de Haën) dissolved in bi-distilled water, added with 2 ml concentrated nitric acid. The solution of CPC (Fischer) at 10–2 mol l–1 was prepared by dissolving 0.8950 g in 250 ml bi-distilled water.
2.3 Preparation of the mixtures
The complex solutions were prepared by mixing successively KD, Fe(III) and finally surfactant solutions. To prevent from any hydrolysis, the pH was adjusted at 1.6 with the help of 1 mol l–1 HCl solution. The final volume was 25 ml. The absorbance was determined 30 min after mixing the reactants, with water/ethanol as the reference solution.
3 Results and discussion
3.1 Absorption spectra of complex solution
The UV absorption spectra are given in Fig. 2 .

Absorption spectra: 1, KD (4 × 10–5 mol l–1); 2, KD (4 × 10–5 mol l–1) + Fe(III) (8 × 10–5 mol l–1); 3, KD (4.10–5 mol l–1) + Fe(III) (1.6 × 10–4 mol l–1); 4, KD (4 × 10–5 mol l–1) + Fe(III) (3.2 × 10–4 mol l–1); 5, KD (4.10–5 mol l–1) + Fe(III) (6.4 × 10–4 mol l–1) at pH = 1.6.
The KD solution (4 × 10–5 mol l–1) presents an absorption band at 260 nm (ε = 3.25 × 104 l mol–1 cm–1) and a shoulder at 218 nm (ε = 2.12 × 104 l mol–1 cm–1). Iron(III) nitrate solution (5 × 10–5 mol l–1) shows a band located at 202 nm (ε = 3,19.104 l mol–1 cm–1).
When adding Fe(III) solution, with increasing concentration, to KD solutions, we note that a strong absorption is always observed around 200 nm while the band at 260 nm becomes a shoulder and slightly shifts down to 255 nm. In the meantime, a weak absorption is observed at 308 nm as a broad band.
3.2 Effects of surfactants
BDTA and CPC gave similar results. However, as CPC was slightly more efficient, we describe here the results with this surfactant only. The spectra of ternary mixtures with CPC are presented in Fig. 3 . We have also recorded the spectra of mixtures of KD and CPC (not shown on figures) and the UV absorption spectrum of KD is not modified.

Absorption spectra of ternary mixtures: 1, KD (2.10–5 mol l–1); 2, KD (2.10–5 mol l–1) + Fe(III) (2.10–6 mol l–1); 3, KD (2 × 10–5 mol l–1) + Fe(III) (2 × 10–6 mol l–1) + CPC (3.2 × 10–3 mol l–1) at pH = 1.6.
Surfactants are well known for their ability to enhance the water solubility of metal complexes by incorporating them into micelles. This phenomenon was widely studied and it has been proved that, with surfactants, the dielectric constant of the medium ε;R is smaller and the complexes are less dissociated. As they are almost non-ionic species, their solubility as well as their molar absorption coefficients is greater than in the absence of surfactant. The critical micellar concentration of CPC was found to be 0.9 × 10–3 mol l–1 at 25 °C [18]. In our case, the optimal conditions for complexation are obtained with CPC concentrations above the critical micellar concentration of 3.2 × 10–3 mol l–1 at 25 °C.
In water/ethanol solution, the spectrum of KD (2 × 10–5 mol l–1) shows two bands, as indicated above. When adding Fe(III) (2 × 10–6 mol l–1), the spectrum remains quite unchanged: the absorbance slightly increases. The addition of CPC (3.2 × 10–3 mol l–1) leads to higher absorbance and the two bands are modified: the broad band at 248 nm is shifted to 260 nm, with two shoulders at 266 nm and 254 nm respectively. The shoulder around 220 nm becomes a sharp band at 213 nm. In the meantime, the absorbance is 0.7 (260 nm) and 0.6 (213 nm) without surfactant and 1.8 and 1.7 at the same respective wavelengths when CPC is added.
3.3 Kinetic study
The variations of the absorbance for both binary and ternary complexes are given in Fig. 4 . It is noticed that the maximal absorbance at 250 nm (binary complex) and 260 nm (ternary complex) is observed for a 20- to 30-min reaction time. Consequently, all measurements were done 30 min after adding last reactant at 25 °C.
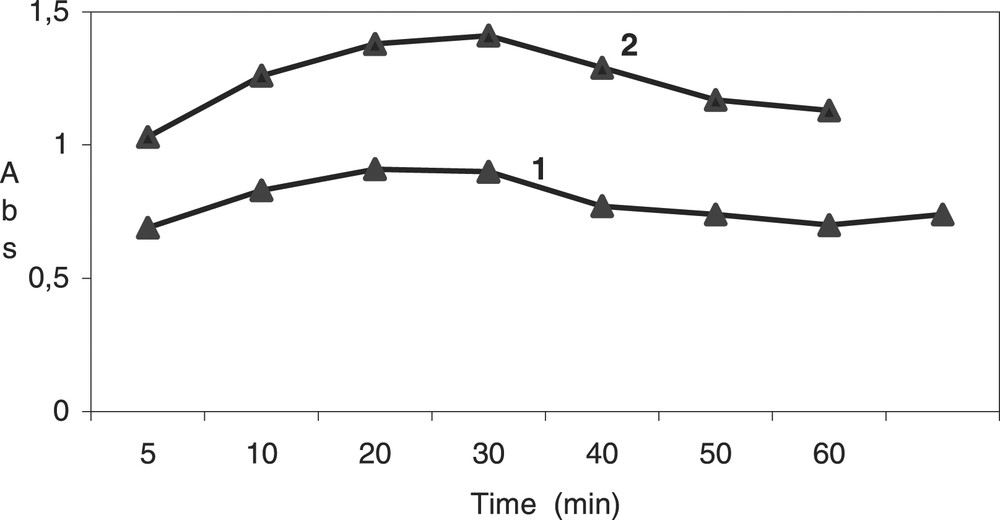
Absorbance variations vs time; 1, ternary complex at 260 nm; 2, binary complex at 250 nm.
3.4 Composition of binary and ternary complexes
We have determined the composition of the complexes with the help of Job’s method [19,20]. The Job’s plots at 250 nm (binary system) and 260 nm (ternary system) for KD, Fe(III) and CPC are given in Fig. 5 . The binary complex is 1:1 species (maximum at 0.5 for the concentration ratio ), while the ternary one is 1:3:8 species (maximum at 0.25 for the same ratio).
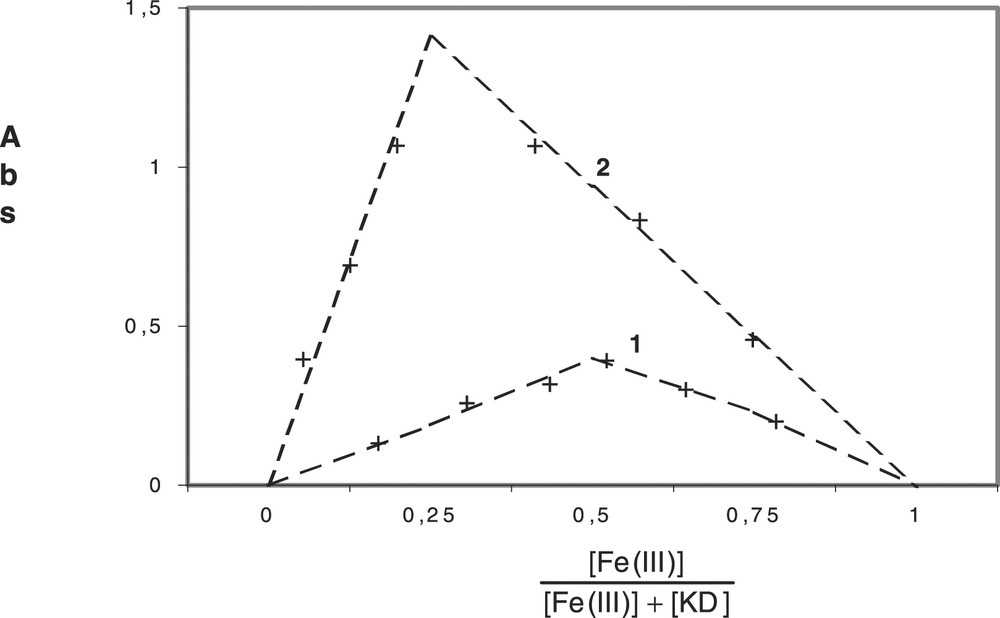
Job’s plot at 250 nm for binary system and 260 nm for ternary system: 1, KD (0.4 × 10–4 mol l–1) + Fe(III) (0.2.10–4 mol l–1); 2, KD + Fe(III) + CPC (3.2 × 10–3 mol l–1).
3.5 Stability constants of the complexes
We have calculated the apparent stability constant of the complexes at 25 °C, using Job’s method of continuous variations and molar ratio method [19]. The complexes are highly stable and their approximate apparent stability constants are 3 × 109 for the binary complex and 6 × 1017 for the ternary one [19].
3.6 Limits for concentration
The Beer’s law is verified up to 1.2 μg ml–1 for Fe(III) without surfactant and 2.4 μg ml–1 when CPC is added. On the opposite side, the lowest Fe(III) concentrations are 0.5 μg ml–1 and 0.1 μg ml–1 respectively. All results are obtained with sufficient accuracy, since 10 replicate analyses of 0.5 μg ml–1 Fe(III) solutions lead to a variation coefficient of less than 1%. These values are lower than that indicated by Safavi et al. [4], 1 μg ml–1, but their technique may be used in various cases like blood or water. When using ferredoxine [12], the detection limit could be as low as 0.001 μg ml–1, but this method is costly because of the use of normal or reverse flow injection analysis.
3.7 Interferences with other metal cations
Table 1 summarises all foreign substances tested for potential interferences with the ternary system KD–Fe(III)–CPC. Each limit is the mean value of at least three determinations. Among metal ions, only Ce(III), with a 5-μg ml–1 limit could interfere with Fe(III). EDTA shall not be present when using KD and CPC to titrate Fe(III), as its concentration should be lower than 10 μg ml–1. EDTA is known to be the most important interfering foreign substance in many analyses [5,12].
Influence of foreign various species on Fe(III) ions (0.5 μg ml–1) detection at 25 °C: tolerance limits (±5.0%).
Species | Limit (μg ml–1) |
KNO3 | 5000 |
CH3COONa | 4500 |
NaCl | 4000 |
Na2SO4 | 4000 |
KH2PO4 | 3600 |
Al(III) | 400 |
Cr(III) | 330 |
Cu(II) | 320 |
Ni(II) | 300 |
Zn(II) | 200 |
Cd(II) | 200 |
KCN | 150 |
Fe(II) | 150 |
EDTA | 10 |
Ce(III) | 5 |
We may remark that it is possible to detect even iron(III) cation traces (e.g., 0.5 μg ml–1) in the presence of a large excess of ferrous ions (up to 150 μg ml–1).
4 Conclusion
The ferric ions could be detected in the range 0.5–1.2 μg ml–1 using KD as ligand. When the cationic surfactant CPC is added, Fe(III) could be titrated from 0.1 to 2.4 μg ml–1. Only Ce(III) ions and EDTA could interfere and other ions or molecules may be present without causing any interference; this technique could be of high interest in water analysis. In fact, very few papers described a specific, simple and cheap method to determine Fe(III) in the presence of Fe(II), mainly in biological systems [16].
Acknowledgements
This work was supported by grants from the ‘Comité mixte d’évaluation et de prospective de coopération inter-universitaire franco-algérienne’ (CMEP).