1 Introduction
In the Russian Federation, the radiochemical plant activities are the main sources for man-made radionuclides in the environment. Several radiochemical plants are responsible for spent nuclear fuel and high-level radioactive wastes treatment, including those located at the Southern Ural, Production Association ‘Mayak’ (‘PA Mayak’) and in western Siberia – Krasnoyarsk Mine chemical plant (MCP) and Tomsk Siberian chemical plant (SCP). The radiological effects of these plants are different and result from technical and equipment conditions. ‘PA Mayak’, the first Soviet enterprise on weapon plutonium production, has the most polluted territories due to the erroneous concept of waste storage to the occurrence of some accidents (in 1957 and 1967).
The environmental monitoring in the territories affected by the radiochemical plants activities is one component of the closed nuclear-fuel cycle programme that is under the development in Russia. Nuclear power plants have their own monitoring capabilities, including network of dosimetry laboratories and automated control systems inside a 30-km zone. According to the experts of the Russian Committee for Hydrometeorology (Rosgidromet), the radiological conditions stay stable in Russia during the last years, except in the territories near ‘PA Mayak’. The data on radionuclide contamination in different regions of Russia are given in the Rosgidromet annual report [1]. However, less data on radionuclide speciation and migration in the environment could be found, despite the fact that speciation governs directions, rates and mechanisms of radionuclide migration. The correct estimation of radiological situation as well as its evaluation is impossible without knowledge on the distribution and migration abilities of radionuclides in the environment.
Some data on actinide speciation and migration ability in highly contaminated areas as well as in the territories with contamination slightly higher than the global fallout level are given in this paper.
2 Results and discussion
The redistribution of radionuclides in the landscape environment after the contamination is a major difficulty during radiochemical monitoring. In order to minimize the influence of natural factors affecting radionuclide distribution (landscape, soil and vegetation types) and to compare results from different laboratories, the sampling should be based on comparative geographical methods used in landscape-geochemical research [2]. Despite this approach, which is widely used nowadays, it does not provide reliable data on radionuclide distribution. This is due to the presence of ‘hot’ particles classified by researchers of the Radium Institute (Saint Petersburg) as belonging to two types [3].
The differences between the first- and second-type ‘hot’ particles are as follows.
- • Prevalence. The prevalence of the second-type particles is in the order of 109–1010 particles/km2 and ~100 particles/km2 for the first-type particles;
- • Radionuclide composition. 137Cs is the predominating radionuclide in the first-type particles, while the second-type particles have more various radionuclide compositions. There are particles enriched in an only nuclide (e.g., 137Cs, 241Am, 60Co or 152Eu, etc.) and polynuclide particles, enriched by radionuclide combination (e.g., 137Cs +241Am or 241Am + 152Eu, or 60CO + 152Eu, etc.);
- • Specific activity. The specific activity of the ‘hot’ second-type particles is 4–6 orders of magnitude higher than the average specific activity of the soil, the specific activity of the first-type particles being 2–3 orders of magnitude higher than the specific activity of the second-type ‘hot’ particles;
- • Contribution to the total specific activity of the tidal soil. The radionuclides included in ‘hot’ second-type particles contribute to more than 50% and even about 100% for some radionuclides (e.g., 241Am);
- • Distribution in the vertical profiles of the tidal soils. The ‘hot’ first-type particles are found in the surface horizons of the tidal soils, while second-type particles are present in all horizons of the studied profiles.
The organic matter of soils and bottom sediments plays the major role in the migration ability of many radionuclides, particularly plutonium and americium [6–9], while the migration of radiocaesium and radiostrontium is not dependent on the organic content. Data in Fig. 1 shows that the higher organic content, the lower migration ability of plutonium and higher migration ability of caesium are observed (since its migration is governed by clay minerals). No clear correlation for strontium is observed. This figure deals with diffusion coefficients versus organic content in the case of an ecosystem with rather simple hydrochemical regime, e.g., vertical migration in the Eastern Ural Radioactive Trace (EURT) zone (Fig. 1). The diffusion coefficients are calculated using the Fick equation for diffusion from flat surface to quasi-infinity. Fig. 2 shows a similar case of stationary conditions in reservoirs at PA ‘Mayak’. Plutonium and americium distribution in floodplain soils of the Yenisei River is governed by more complicated mechanisms, as illustrated in Fig. 3. Such distributions cannot be described by models that do not consider different sources of radionuclides and species distribution.
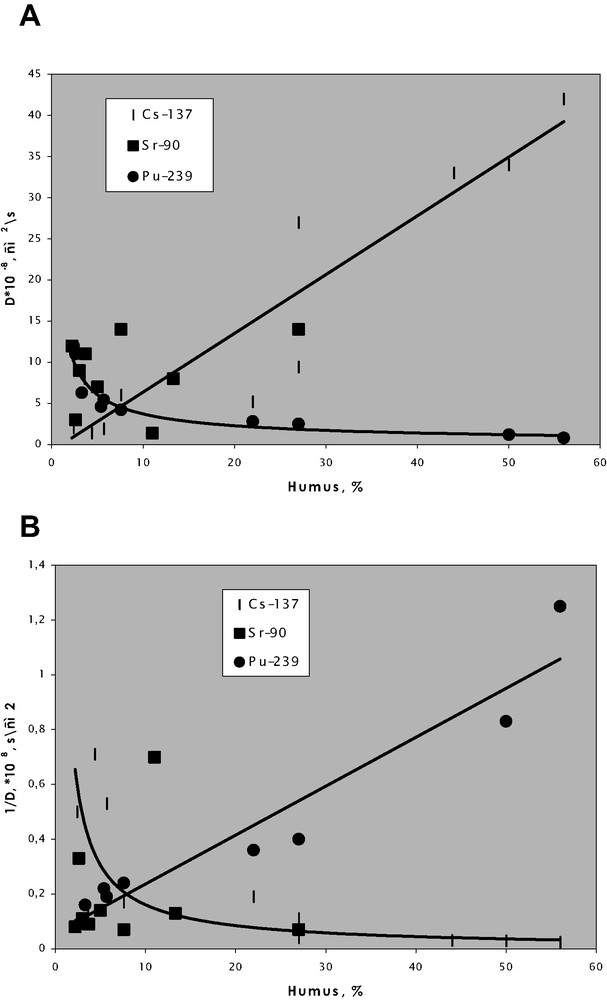
Diffusion coefficients of radionuclides in upper horizons of EURT soils (A) and diffusional resistance (B) of these soils.

Accumulation of 239Pu and 239Am by bottom sediments of basin-10 (PA Mayak) vs the content of organic matter.
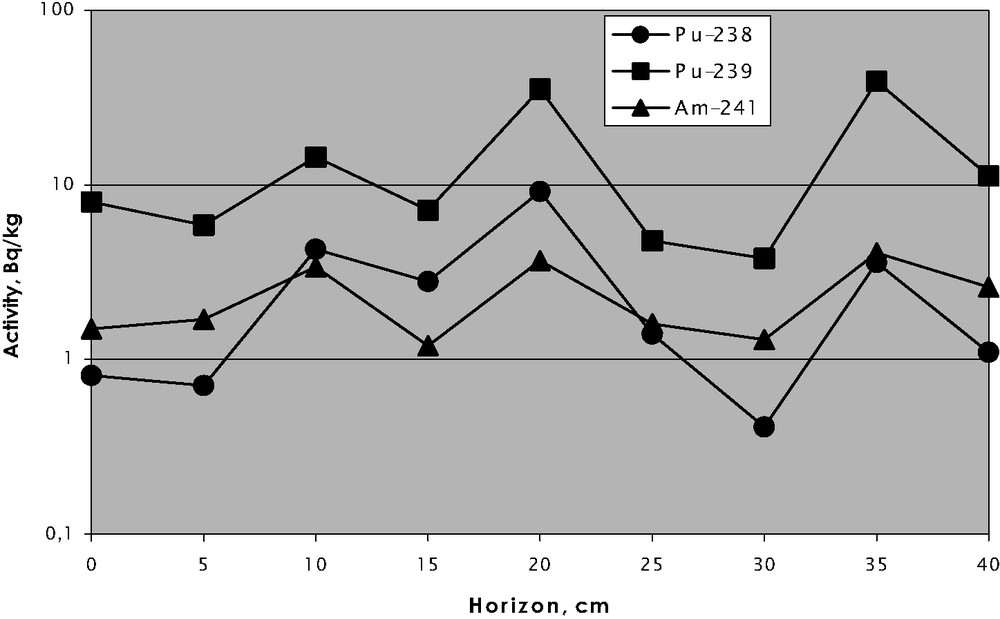
Vertical distribution of Pu and Am in the floodplain soil of the Yenisei River.
The fractionation of organic and inorganic matter in floodplain soils of the Yenisei River indicates that plutonium is mostly associated with low mobile organic group, in contrast to americium (Fig. 4). This could explain that the concentration of plutonium decreases greatly with distance from MCP if compared to americium (Fig. 5).

Percentage of Pu and Am in various groups of organic matter of the flood-land soils of the Yenisey River.

Content of radionuclides in bottom sediments of the Yenisei River vs the distance from the discharge location (MCP).
The redox state of elements appears to be a key factor for the actinide behaviour in the environment. This factor determines kinetics and thermodynamics of co-precipitation, sorption on active minerals, complex, and colloid formation. The data on plutonium oxidation state determination in environmental samples is rather limited. Several methods could be used, including those proposed by G. Choppin, based on TTA extraction [10]. However, this method has two potential disadvantages: need of pH adjustment to 4.0–5.0 and TTA reducing properties. We proposed a membrane extraction method for plutonium redox speciation [11], which seems to provide reliable quantitative separation, with minimal effect on the original oxidation state distribution.
The pentavalent plutonium compounds are the most soluble among other oxidation forms, which is demonstrated by data presented in Table 1 for the Yenisei River floodplain soils. Only about 1% of total plutonium is leached by water, but 80% of it is found in the Pu(V) form. The share of Pu(V) in exchangeable fraction (extracted by acetate) reaches 55%. However, according to our data and those published earlier, if plutonium is found in pentavalent form, it is easily reduced upon sorption to minerals (see Fig. 6). As it is shown in [12], fast plutonium-sorption kinetics on the goethite (α-FeOOH) surface is due to reduction on the mineral surface, which contains Fe2+.
Speciation of plutonium in various soil leaches
Water leach | ||||||
Fraction | After 0.2-μm filter | After 10-kDa filter | ||||
Oxidation state | IV | V | III + VI | IV | V | III + VI |
Pu (% of total in fraction) | 14 | 79 | 7 | 12 | 82 | 6 |
Pu (% of total in soil sample) | 0.23 | 1.3 | 0.12 | 0.08 | 0.6 | 0.04 |
1 M CH3COO leach | ||||||
Fraction | After 0.2-μm filter | After 10-kDa filter | ||||
Oxidation state | IV | V | III + VI | IV | V | III + VI |
Pu (% of total in fraction) | 39 | 55 | 6 | 30 | 61 | 9 |
Pu (% of total in soil sample) | 2.4 | 3.4 | 0.4 | 1.3 | 2.6 | 0.4 |
1 M HCl leach after 0.2-μm filter | ||||||
Pu | Am | |||||
Oxidation state | IV | III + V + VI | IV | III + V + VI | ||
Ac (% of total in fraction) | 73 | 27 | — | 100 | ||
Ac (% of total in soil sample) | 25.0 | 9.3 | 49.4 |
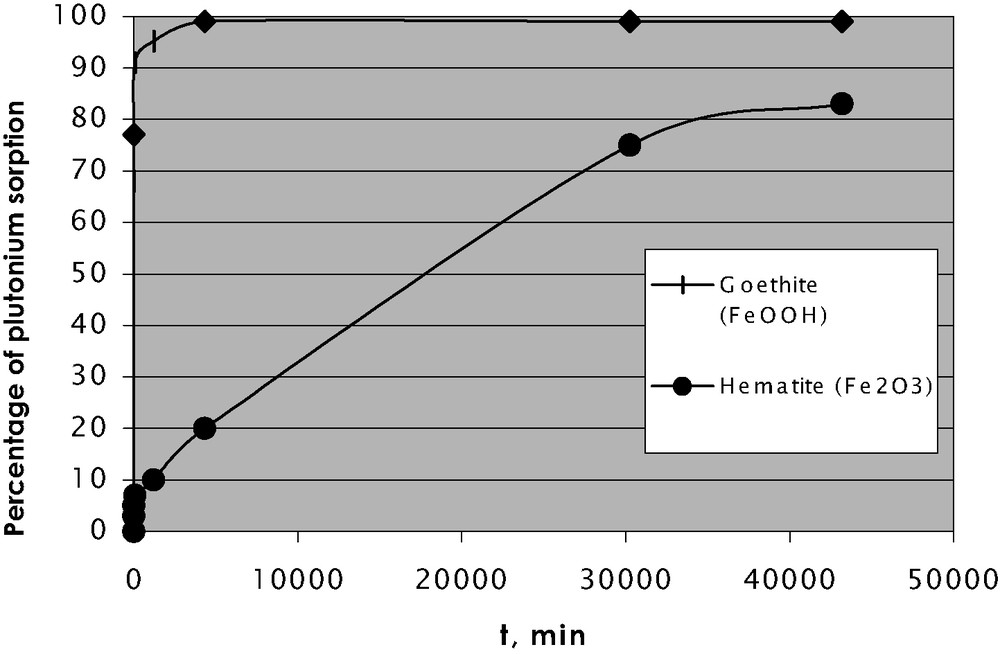
Kinetics of plutonium (V) sorption on goethite and hematite.
Influence of natural-water composition on the actinide oxidation state can be shown by the example of groundwaters of ‘PA Mayak’ and strata waters of SCP. These selected under-investigation zones are characterized by different actinide migration conditions. The ground waters within the Karachai (Mayak) zone are weakly oxidizing, with appreciable carbonation not far from the source [13]. The stratum waters of the SCC radioactive waste pump-in site are determined by reducing conditions and rather low carbonation.
The results obtained are displayed in Tables 2 and 3. The data in these tables are averaged according to the results of three series of membrane-extraction experiments.
Oxidizing forms of the actinides found in the Mayak ground waters, percentage of the total content of an element in a sample
Number of samples | U(IV) | U(VI) | Np(IV) | Np(V) | Pu(III) | Pu(IV) | Pu(V) | Pu(VI) | Am(III) a |
1 | – | 100 | – | 100 | – | 54 | 37 | 9 | 100 |
2 | – | 100 | – | 100 | – | 49 | 44 | 7 | 100 |
3 | – | 100 | – | 100 | – | 60 | 40 | – | 100 |
4 | – | 100 | – | 100 | – | 67 | 33 | – | 100 |
a Determined to control correctness of liquid membranes functioning.
Oxidizing forms of the actinides found in the SCP stratum waters, percentage of the total content of an element in a sample
N | U(IV) | U(VI) | Np(IV) | Np(V) | Pu(III) | Pu(IV) | Pu(V) | Pu(VI) | Am(III) a |
1 | 53 | 47 | 59 | 41 | 5 | 85 | 10 | – | 100 |
2 | 64 | 36 | 76 | 24 | – | 100 | – | – | 100 |
3 | 57 | 43 | 72 | 28 | 4 | 96 | – | – | 100 |
4 | 39 | 61 | 55 | 45 | 9 | 91 | – | – | 100 |
a Determined to control correctness of liquid membranes functioning.
Obviously, uranium and neptunium have no alternative U(VI) and Np(V) form in the ‘PA Mayak’ groundwaters. Plutonium takes the same way as the sum of Pu(IV) and Pu(V) does. The main difference from the literature data with respect to surface waters consists in the absence of reliably identified Pu(VI) [14].
The actinide distribution on oxidizing forms in the SCC stratum waters (Table 3) is more complicated than for the Mayak groundwaters (Table 2). The prevalence of lower oxidizing states of the actinides is appreciable. However, only Pu(IV) appears to be the dominant form of plutonium. For uranium and neptunium, a share of forms whose behaviour is similar to U(VI) and Np(V) makes from 20 up to 40%.
Colloid transport is considered as one of the effective mechanisms of actinide migration in underground systems, especially in reducing conditions and when significant amounts of complexing substances are absent [15]. However, the nature of the colloids at the under-investigation depth condition remains unknown so far.
The data on the actinide distribution on the size of particles for the Mayak groundwaters are presented in Fig. 7 (by the example of well #176). The analysis of speciation data (10 wells) allows us to draw the following conclusions.
- • (i) The actinide (even plutonium) content in 450-nm particles is abnormally low (in comparison with the surface waters) [9], and the distribution in various fractions is obviously of a casual nature. It can be explained by the low content of high-molecular humic substances in rather large-depth groundwaters (80–100 m).
- • The relative actinide content in 3000 up to 30 000 molecular-weight particles increases in the following succession: U < Np < < Am + Cm < Pu. Besides, uranium and plutonium shares in a filtrate (a solution with the content of particles of less than 3000) decrease from 93 up to 83% and from 29 up to 9%, respectively, with a distance from the pollution source. For americium and neptunium, such tendency is not found. The intensification of the colloid formation for uranium and plutonium may qualitatively be explained by the total carbonate content decrease. In fact, for neptunium and transplutonium trivalent elements, this factor plays a smaller role, especially in the presence of organic substances. However, the strict correlation between the carbonate content and the actinide retention degree by the filters is not found.
- • The effect of the phosphate content on retention degree of the actinides is not found, possibly because the increased content of the former is observed in some wells only.
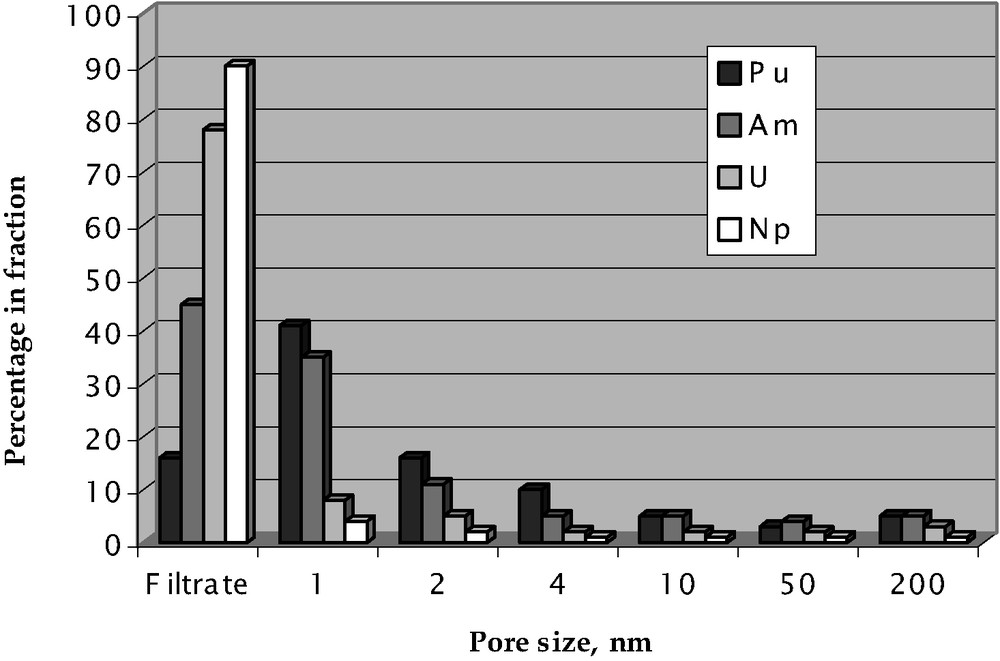
Membrane fractionation of particles of the groundwaters near Karachay.
3 Conclusions
Thus, it is possible to assume that the most mobile actinide forms in the under-investigation system appear to be negatively charged carbonate complexes having the following composition: Ac(VI)O2(CO3)34– and Ac(V)O2(CO3)23–.
Detection of Pu(V) forms modifies substantially the initial ideas about the primary colloid transport of this element. In spite of the fact that only 10 to 30% of plutonium is found in a true solution, these forms certainly are considerably more mobile and the colloid-facilitated transfer becomes dominant, obviously only in strong reducing conditions.
Ac(V) may partly be of cationic forms [16] and be sorbed on the enclosing rocks, though having not high sorption factors (in comparison with the lower oxidizing states). However, the processes of precipitation and sorption for Ac(VI) play a prior part. The last statement proves to be true that the ratio of 234U/237Np technogenic isotopes decreases in 50 times with 15-km distance from the source in the under-investigation groundwaters at the ‘PA Mayak’ site.
The dissolved organic substance has a certain effect on Am(III) and Cm(III) migration intensity in the under-investigation conditions. The metal-fulvic complexes including appreciable amounts of americium are mobile enough, though the last factor depends essentially on the environmental concentration conditions. Therefore, for Ac(III), it is necessary to take into account the transport of both carbonate and organic complexes in these ecosystems.