1 Introduction
The acrylic esters have been an important chemical group with considerable application in industry and have a wider area of use, not only in the fields like dental applications and synthetic rubber but also in optical plastics and as dielectric coatings [1]. Alcohols play an important role in many chemical reactions due to the ability to undergo self-association with manifold internal structures and are in wide use in industry and science as reagents, solvents and fuels [2]. The solvent effects play an important role in organic reactivity phenomena such as the chemical equilibrium, the rate of chemical reactions, the conversion of the polymerization, and so on. Experimental investigation of properties of organic compounds is of great value in understanding the nature of molecular interaction between the molecules. Hydrogen bonds constitute a very interesting class of intermolecular interactions, which are of extreme importance in many fields of chemistry and molecular biology. The study of the H-bonds of the type O–H····O=C occupies a position of considerable importance as it relates to the study of biopolymers. Thus the study and knowledge of properties of the ternary mixtures of acrylic esters with polar associating liquids (alcohols) in non-polar solvents is expected to provide useful and vital process parameters for efficient design of transesterification processes of industrial interest. Keeping both the industrial and scientific interests in mind, we report here the results of our investigation on the H-bonded complexes of alcohols with acrylic esters in non-polar solvents (carbon tetrachloride and benzene) using dielectric and FTIR spectroscopic measurements. This study is expected to provide better understanding of the nature of molecular orientation processes.
2 Experimental details
2.1 Materials
The alkyl methacrylate monomers (methyl methacryrate (MMA), ethyl methacryrate (EMA) and butyl methacryrate (BMA)) (> 99% pure) used in this investigation were purchased from Aldrich and used without further purification. AnalaR grade carbon tetrachloride, benzene, and alcohols (1-propanol, 1-pentanol, 1-heptanol and 1-octanol) (> 99.5% pure) were purified by standard methods [3]. The physical parameters of all the chemicals used here have been checked against their literature values.
2.2 Dielectric study
According to Higasi's method [4], the individual relaxation time τ(1) is described by
(1) |
(2) |
(3) |
(4) |
The molar free energy of activation, for both dielectric relaxation ΔFτ as well as the viscous flow processes ΔFη have been evaluated using the equations given by Glasstone et al. [5]
(5) |
(6) |
The dielectric constant ε′ and loss ε″ at the microwave frequency of 9.84 GHz were measured using the methods of Roberts and Von Hippel [6] modified by Dakin and Works [7]. The experimental set-up and the procedure employed were the same as reported by Aggarval et al. [8]. The values of ε′ and ε″ so obtained are accurate within ± 1% and ± 5%, respectively. The static dielectric constants (ε0) were measured by heterodyne beat method at 298 K using a commercial instrument, Dipolemeter DM 01 supplied by Wissenschaijftlich Technische Werkstatter, Germany operated at 220 V. Error in the measurement of ε0 is less than ± 0.5%. The refractive indices were measured by an Abbe's refractometer with an accuracy of ± 0.0005. The percentage of deviation in the measurement of refractive index is about ± 0.02%. The viscosities were measured with the help of Oswald's viscometer. The temperature of all the measurements were maintained at 25 ± 0.1 °C using water circulating thermostat.
The proton donors (alcohols) and the acceptors (acrylic esters) under study were separately dissolved at the same molar concentration in the solvents (carbon tetrachloride and benzene). Their dielectric constants were measured separately. Then the two solutions were mixed in different proportions but with the total concentration kept at a fixed value (0.3 mol l–1) and were subjected to the dielectric constant measurements.
2.3 FTIR study
Spectra were recorded with a Nicolet Avatar 360 FTIR spectrometer and a NaCl cell, using 0.1 mm spacer at 298 K in the region of 4000–400 cm–1. The spectrometer possesses autoalign energy optimization and dynamically aligned interferometer. It is fitted with a KBr beam splitter, a DTGS-Detector and Everlgo™ mid-IR source. A base line correction was made for the spectra recorded.
3 Results and discussion
The values of static dielectric constant (ε0), dielectric constant at angular frequency (ε∞), dielectric constant (ε′), dielectric loss (ε″), relaxation time τ(1) due the individual rotation of the molecule, relaxation time τ(2) due the whole or overall rotation of the molecule, the most probable relaxation time τ(0), activation energy ΔFτ due to dielectric relaxation process and activation energy ΔFη due to viscous flow of proton donors (1-propanol, 1-pentanol, 1-heptanol and 1-octanol) with proton acceptors (MMA, EMA and BMA) in carbon tetrachloride and benzene at 298 K have been provided in Tables 1–12. A perusal of Tables 1, 3, 5, 7, 9, 11 shows that, it is noticed that the value of ε′ and ε″ is found to be decreased by increasing number of carbon atoms in the chain for all the systems studied. This trend could be attributed to the decrease in the number of dipoles in the complex, which may lead to a decrease in the molar volume of the rotated molecule [9].
Values of static dielectric constant (ε0), dielectric constant at angular frequency (ε∞), dielectric constant (ε′) and dielectric loss (ε″) for alcohols with methyl methacrylate (MMA) in CCl4
Alcohols | Molar ratio, Alcohol:Ester | ε′ | ε″ | ε0 | ε∞ |
1-Propanol | 1:3 | 2.5927 | 0.2634 | 2.8952 | 2.2206 |
1:2 | 2.6165 | 0.2848 | 3.0124 | 2.2292 | |
1:1 | 2.6208 | 0.3179 | 3.1566 | 2.2391 | |
2:1 | 2.6141 | 0.2897 | 3.0111 | 2.2291 | |
3:1 | 2.5914 | 0.2646 | 2.8948 | 2.2215 | |
1-Pentanol | 1:3 | 2.5212 | 0.2125 | 2.8215 | 2.2484 |
1:2 | 2.5412 | 0.2422 | 2.9311 | 2.2527 | |
1:1 | 2.5554 | 0.2709 | 3.0715 | 2.2602 | |
2:1 | 2.5418 | 0.2435 | 2.9324 | 2.2521 | |
3:1 | 2.5215 | 0.2117 | 2.8221 | 2.2495 | |
1-Heptanol | 1:3 | 2.4775 | 0.1822 | 2.7705 | 2.2549 |
1:2 | 2.4946 | 0.2044 | 2.8879 | 2.2603 | |
1:1 | 2.5108 | 0.2306 | 3.0125 | 2.2673 | |
2:1 | 2.4957 | 0.2026 | 2.8828 | 2.2608 | |
3:1 | 2.4793 | 0.1835 | 2.7759 | 2.2554 | |
1-Octanol | 1:3 | 2.4489 | 0.1504 | 2.7228 | 2.2574 |
1:2 | 2.4607 | 0.1711 | 2.8235 | 2.2611 | |
1:1 | 2.4874 | 0.2019 | 2.9718 | 2.2705 | |
2:1 | 2.4623 | 0.1735 | 2.8249 | 2.2616 | |
3:1 | 2.4491 | 0.1516 | 2.7252 | 2.2569 |
Values of dielectric relaxation time and molar free energies of activation (ΔFτ, ΔFη) for alcohols with ethyl methacrylate (EMA) in benzene at 298 K
Alcohols | Molar ratio Alcohol:Ester | Relaxation time | ΔFτ (kJ mol–1) | ΔFη (kJ mol–1) | ||
τ(1) | τ(2) | τ(0) | ||||
ps | ps | ps | ||||
1-Propanol | 1:3 | 15.48 | 21.25 | 18.14 | 12.10 | 13.65 |
1:2 | 16.54 | 22.82 | 19.43 | 12.27 | 13.89 | |
1:1 | 18.38 | 29.62 | 23.33 | 12.92 | 14.67 | |
2:1 | 16.57 | 22.79 | 19.43 | 12.27 | 13.91 | |
3:1 | 15.62 | 21.15 | 18.18 | 12.09 | 13.62 | |
1-Pentanol | 1:3 | 19.18 | 26.41 | 22.50 | 12.64 | 14.31 |
1:2 | 20.22 | 29.17 | 24.28 | 12.88 | 14.56 | |
1:1 | 22.43 | 33.05 | 27.23 | 13.19 | 14.98 | |
2:1 | 20.24 | 30.50 | 24.85 | 12.99 | 14.60 | |
3:1 | 19.20 | 26.42 | 22.52 | 12.64 | 14.34 | |
1-Heptanol | 1:3 | 22.87 | 30.42 | 26.37 | 12.99 | 14.67 |
1:2 | 23.67 | 32.89 | 27.90 | 13.18 | 14.86 | |
1:1 | 25.81 | 38.97 | 31.71 | 13.60 | 15.25 | |
2:1 | 24.40 | 32.26 | 28.06 | 13.13 | 14.80 | |
3:1 | 22.94 | 30.35 | 26.39 | 12.98 | 14.65 | |
1-Octanol | 1:3 | 24.81 | 32.91 | 28.57 | 13.18 | 14.92 |
1:2 | 26.51 | 35.02 | 30.47 | 13.33 | 15.04 | |
1:1 | 28.28 | 41.38 | 34.21 | 13.75 | 15.48 | |
2:1 | 26.57 | 35.06 | 30.52 | 13.34 | 15.07 | |
3:1 | 24.84 | 32.98 | 28.62 | 13.19 | 14.95 |
Values of static dielectric constant (ε0), dielectric constant at angular frequency (ε∞), dielectric constant (ε′) and dielectric loss (ε″) for alcohols with butyl methacrylate (BMA) in benzene
Alcohols | Molar ratio Alcohol:Ester | ε′ | ε″ | ε0 | ε∞ |
1-Propanol | 1:3 | 2.5248 | 0.2061 | 2.8237 | 2.2905 |
1:2 | 2.5395 | 0.2307 | 2.9064 | 2.2929 | |
1:1 | 2.5455 | 0.2692 | 3.0628 | 2.2948 | |
2:1 | 2.5391 | 0.2314 | 2.9075 | 2.2933 | |
3:1 | 2.5253 | 0.2072 | 2.8244 | 2.2916 | |
1-Pentanol | 1:3 | 2.4546 | 0.1735 | 2.7552 | 2.2944 |
1:2 | 2.4643 | 0.1916 | 2.8247 | 2.2968 | |
1:1 | 2.4809 | 0.2255 | 2.9522 | 2.2982 | |
2:1 | 2.4654 | 0.1928 | 2.8283 | 2.2971 | |
3:1 | 2.4563 | 0.1746 | 2.7594 | 2.2955 | |
1-Heptanol | 1:3 | 2.4263 | 0.1547 | 2.7328 | 2.2961 |
1:2 | 2.4315 | 0.1724 | 2.7969 | 2.2982 | |
1:1 | 2.4416 | 0.1948 | 2.9189 | 2.3004 | |
2:1 | 2.4323 | 0.1729 | 2.7996 | 2.2991 | |
3:1 | 2.4271 | 0.1554 | 2.7365 | 2.2972 | |
1-Octanol | 1:3 | 2.4024 | 0.1226 | 2.6612 | 2.2985 |
1:2 | 2.4126 | 0.1443 | 2.7328 | 2.3013 | |
1:1 | 2.4225 | 0.1682 | 2.853 | 2.3036 | |
2:1 | 2.4123 | 0.1452 | 2.7357 | 2.3029 | |
3:1 | 2.4034 | 0.1235 | 2.6653 | 2.2991 |
Values of dielectric relaxation time and molar free energies of activation (ΔFτ, ΔFη) for alcohols with butyl methacrylate (BMA) in benzene at 298 K
Alcohols | Molar ratio Alcohol:Ester | Relaxation time | ΔFτ (kJ mol–1) | ΔFη (kJ mol–1) | ||
τ(1) | τ(2) | τ(0) | ||||
ps | ps | ps | ||||
1-Propanol | 1:3 | 17.23 | 24.49 | 20.54 | 12.45 | 14.16 |
1:2 | 18.18 | 26.86 | 22.10 | 12.68 | 14.35 | |
1:1 | 20.81 | 32.45 | 25.99 | 13.15 | 14.87 | |
2:1 | 18.30 | 26.88 | 22.18 | 12.68 | 14.38 | |
3:1 | 17.37 | 24.38 | 20.58 | 12.44 | 14.13 | |
1-Pentanol | 1:3 | 22.91 | 29.26 | 25.89 | 12.89 | 14.57 |
1:2 | 23.93 | 31.76 | 27.57 | 13.09 | 14.72 | |
1:1 | 25.32 | 35.29 | 29.89 | 13.35 | 15.19 | |
2:1 | 23.94 | 31.78 | 27.58 | 13.09 | 14.76 | |
3:1 | 22.94 | 29.31 | 25.93 | 12.89 | 14.59 | |
1-Heptanol | 1:3 | 26.68 | 33.46 | 29.88 | 13.22 | 14.83 |
1:2 | 28.82 | 35.79 | 32.12 | 13.39 | 15.02 | |
1:1 | 30.21 | 41.37 | 35.35 | 13.75 | 15.48 | |
2:1 | 28.94 | 35.87 | 32.22 | 13.39 | 15.05 | |
3:1 | 26.89 | 33.62 | 30.07 | 13.23 | 14.86 | |
1-Octanol | 1:3 | 28.91 | 35.65 | 32.10 | 13.38 | 15.06 |
1:2 | 30.84 | 37.47 | 34.00 | 13.50 | 15.24 | |
1:1 | 32.80 | 43.22 | 37.65 | 13.86 | 15.69 | |
2:1 | 31.80 | 37.61 | 34.58 | 13.51 | 15.28 | |
3:1 | 28.96 | 35.81 | 32.21 | 13.39 | 15.10 |
Values of dielectric relaxation time and molar free energies of activation (ΔFτ, ΔFη) for alcohols with methyl methacrylate (MMA) in CCl4 at 298 K
Alcohols | Molar ratio Alcohol:Ester | Relaxation time | ΔFτ (kJ mol–1) | ΔFη (kJ mol–1) | ||
τ(1) | τ(2) | τ(0) | ||||
ps | ps | ps | ||||
1-Propanol | 1:3 | 15.65 | 19.39 | 17.42 | 11.87 | 13.54 |
1:2 | 16.06 | 23.47 | 19.42 | 12.34 | 14.03 | |
1:1 | 18.27 | 28.46 | 22.80 | 12.82 | 14.56 | |
2:1 | 16.47 | 23.14 | 19.52 | 12.31 | 14.00 | |
3:1 | 15.84 | 19.36 | 17.52 | 11.87 | 13.58 | |
1-Pentanol | 1:3 | 19.41 | 23.86 | 21.52 | 12.38 | 14.01 |
1:2 | 20.39 | 27.18 | 23.54 | 12.71 | 14.46 | |
1:1 | 22.07 | 32.17 | 26.64 | 13.12 | 14.89 | |
2:1 | 20.38 | 27.09 | 23.49 | 12.70 | 14.43 | |
3:1 | 19.42 | 23.98 | 21.58 | 12.40 | 14.05 | |
1-Heptanol | 1:3 | 22.84 | 27.16 | 24.90 | 12.70 | 14.42 |
1:2 | 23.58 | 32.49 | 27.68 | 13.15 | 14.81 | |
1:1 | 25.03 | 36.74 | 30.32 | 13.45 | 15.07 | |
2:1 | 23.27 | 32.26 | 27.40 | 13.13 | 14.78 | |
3:1 | 22.78 | 27.29 | 24.94 | 12.72 | 14.46 | |
1-Octanol | 1:3 | 24.51 | 30.75 | 27.46 | 13.01 | 14.84 |
1:2 | 25.87 | 35.81 | 30.43 | 13.39 | 15.02 | |
1:1 | 26.43 | 40.51 | 32.72 | 13.70 | 15.46 | |
2:1 | 25.97 | 35.29 | 30.28 | 13.35 | 14.98 | |
3:1 | 24.54 | 30.75 | 27.47 | 13.01 | 14.86 |
Values of static dielectric constant (ε0), dielectric constant at angular frequency (ε∞), dielectric constant (ε′) and dielectric loss (ε″) for alcohols with ethyl methacrylate (EMA) in CCl4
Alcohols | Molar ratio Alcohol:Ester | ε′ | ε″ | ε0 | ε∞ |
1-Propanol | 1:3 | 2.5479 | 0.2325 | 2.8415 | 2.2404 |
1:2 | 2.5688 | 0.2621 | 2.9711 | 2.2422 | |
1:1 | 2.5713 | 0.2812 | 3.1017 | 2.2471 | |
2:1 | 2.5691 | 0.2637 | 2.9739 | 2.2417 | |
3:1 | 2.5474 | 0.2336 | 2.8401 | 2.2401 | |
1-Pentanol | 1:3 | 2.4855 | 0.1886 | 2.7763 | 2.2565 |
1:2 | 2.5082 | 0.2222 | 2.8956 | 2.2582 | |
1:1 | 2.5153 | 0.2402 | 3.0397 | 2.2687 | |
2:1 | 2.5088 | 0.2219 | 2.8947 | 2.2586 | |
3:1 | 2.4845 | 0.1897 | 2.7791 | 2.2561 | |
1-Heptanol | 1:3 | 2.4443 | 0.1375 | 2.7027 | 2.2601 |
1:2 | 2.4597 | 0.1644 | 2.8162 | 2.2616 | |
1:1 | 2.4782 | 0.1884 | 2.9314 | 2.2728 | |
2:1 | 2.4588 | 0.1635 | 2.8161 | 2.2612 | |
3:1 | 2.4433 | 0.1371 | 2.7002 | 2.2593 | |
1-Octanol | 1:3 | 2.4234 | 0.1176 | 2.6578 | 2.2609 |
1:2 | 2.4323 | 0.1362 | 2.7433 | 2.2622 | |
1:1 | 2.4477 | 0.1625 | 2.8666 | 2.2736 | |
2:1 | 2.4331 | 0.1352 | 2.7444 | 2.2617 | |
3:1 | 2.4216 | 0.1173 | 2.6565 | 2.2601 |
Values of dielectric relaxation time and molar free energies of activation (ΔFτ, ΔFη) for alcohols with ethyl methacrylate (EMA) in CCl4 at 298 K
Alcohols | Molar ratio Alcohol:Ester | Relaxation time | ΔFτ (kJ mol–1) | ΔFη (kJ mol–1) | ||
τ(1) | τ(2) | τ(0) | ||||
ps | ps | ps | ||||
1-Propanol | 1:3 | 17.88 | 21.32 | 19.53 | 12.11 | 13.81 |
1:2 | 18.54 | 25.92 | 21.92 | 12.59 | 14.25 | |
1:1 | 20.09 | 31.85 | 25.30 | 13.10 | 14.86 | |
2:1 | 18.59 | 25.92 | 21.95 | 12.59 | 14.28 | |
3:1 | 17.98 | 21.16 | 19.50 | 12.09 | 13.79 | |
1-Pentanol | 1:3 | 22.57 | 26.04 | 24.24 | 12.60 | 14.28 |
1:2 | 23.15 | 29.44 | 26.10 | 12.90 | 14.63 | |
1:1 | 25.56 | 36.87 | 30.70 | 13.46 | 15.12 | |
2:1 | 23.09 | 29.37 | 26.04 | 12.90 | 14.66 | |
3:1 | 22.80 | 26.22 | 24.45 | 12.62 | 14.33 | |
1-Heptanol | 1:3 | 24.11 | 31.73 | 27.66 | 13.09 | 14.72 |
1:2 | 25.19 | 36.62 | 30.37 | 13.45 | 15.06 | |
1:1 | 27.08 | 40.62 | 33.16 | 13.70 | 15.43 | |
2:1 | 25.17 | 36.90 | 30.48 | 13.46 | 15.09 | |
3:1 | 24.09 | 31.64 | 27.61 | 13.08 | 14.70 | |
1-Octanol | 1:3 | 26.62 | 33.66 | 29.93 | 13.24 | 14.98 |
1:2 | 27.98 | 38.56 | 32.85 | 13.57 | 15.21 | |
1:1 | 31.83 | 43.53 | 37.22 | 13.87 | 15.56 | |
2:1 | 27.34 | 38.88 | 32.60 | 13.59 | 15.25 | |
3:1 | 26.91 | 33.82 | 30.17 | 13.25 | 15.00 |
Values of static dielectric constant (ε0), dielectric constant at angular frequency (ε∞), dielectric constant (ε′) and dielectric loss (ε″) for alcohols with butyl methacrylate (BMA) in CCl4
Alcohols | Molar ratio Alcohol:Ester | ε′ | ε″ | ε0 | ε∞ |
1-Propanol | 1:3 | 2.5129 | 0.2025 | 2.8115 | 2.2514 |
1:2 | 2.5298 | 0.2321 | 2.9211 | 2.2592 | |
1:1 | 2.5401 | 0.2492 | 3.0596 | 2.2611 | |
2:1 | 2.5281 | 0.2337 | 2.9204 | 2.2597 | |
3:1 | 2.5134 | 0.2036 | 2.8101 | 2.2525 | |
1-Pentanol | 1:3 | 2.4484 | 0.1509 | 2.7208 | 2.2574 |
1:2 | 2.4582 | 0.1722 | 2.8131 | 2.2581 | |
1:1 | 2.4784 | 0.1939 | 2.9392 | 2.2702 | |
2:1 | 2.4588 | 0.1735 | 2.8111 | 2.2571 | |
3:1 | 2.4491 | 0.1507 | 2.7201 | 2.2575 | |
1-Heptanol | 1:3 | 2.4155 | 0.1162 | 2.6505 | 2.2589 |
1:2 | 2.4266 | 0.1344 | 2.7309 | 2.2613 | |
1:1 | 2.4438 | 0.1506 | 2.8287 | 2.2754 | |
2:1 | 2.4257 | 0.1326 | 2.7318 | 2.2608 | |
3:1 | 2.4173 | 0.1185 | 2.6561 | 2.2594 | |
1-Octanol | 1:3 | 2.3989 | 0.0903 | 2.5928 | 2.2614 |
1:2 | 2.4107 | 0.1125 | 2.6834 | 2.2632 | |
1:1 | 2.4311 | 0.1309 | 2.7837 | 2.2796 | |
2:1 | 2.4113 | 0.1135 | 2.6849 | 2.2621 | |
3:1 | 2.3991 | 0.0916 | 2.5992 | 2.2609 |
Values of dielectric relaxation time and molar free energies of activation (ΔFτ, ΔFη) for alcohols with butyl methacrylate (BMA) in CCl4 at 298 K
Alcohols | Molar ratio Alcohol: Ester | Relaxation time | ΔFτ (kJ mol–1) | ΔFη (kJ mol–1) | ||
τ(1) | τ(2) | τ(0) | ||||
ps | ps | ps | ||||
1-Propanol | 1:3 | 19.70 | 24.90 | 22.15 | 12.49 | 14.18 |
1:2 | 21.45 | 28.47 | 24.71 | 12.82 | 14.56 | |
1:1 | 22.02 | 35.20 | 27.84 | 13.35 | 15.04 | |
2:1 | 21.86 | 28.35 | 24.89 | 12.81 | 14.52 | |
3:1 | 19.87 | 24.61 | 22.11 | 12.46 | 14.14 | |
1-Pentanol | 1:3 | 24.72 | 30.48 | 27.45 | 12.99 | 14.62 |
1:2 | 25.92 | 34.80 | 30.03 | 13.32 | 15.01 | |
1:1 | 27.22 | 40.13 | 33.05 | 13.67 | 15.34 | |
2:1 | 25.74 | 34.29 | 29.71 | 13.28 | 14.97 | |
3:1 | 24.54 | 30.37 | 27.30 | 12.98 | 14.59 | |
1-Heptanol | 1:3 | 28.56 | 34.15 | 31.23 | 13.27 | 14.95 |
1:2 | 29.32 | 38.23 | 33.48 | 13.55 | 15.21 | |
1:1 | 31.59 | 43.16 | 36.92 | 13.85 | 15.58 | |
2:1 | 29.08 | 38.98 | 33.67 | 13.60 | 15.28 | |
3:1 | 28.59 | 34.03 | 31.19 | 13.26 | 14.92 | |
1-Octanol | 1:3 | 30.74 | 36.26 | 33.39 | 13.42 | 15.17 |
1:2 | 31.87 | 40.93 | 36.12 | 13.72 | 15.31 | |
1:1 | 34.75 | 45.49 | 39.76 | 13.98 | 15.68 | |
2:1 | 31.27 | 40.71 | 35.67 | 13.71 | 15.28 | |
3:1 | 30.75 | 36.89 | 33.68 | 13.46 | 15.22 |
Values of static dielectric constant (ε0), dielectric constant at angular frequency (ε∞), dielectric constant (ε′) and dielectric loss (ε″) for alcohols with methyl methacrylate (MMA) in benzene
Alcohols | Molar ratio Alcohol: Ester | ε′ | ε″ | ε0 | ε∞ |
1-Propanol | 1:3 | 2.6653 | 0.2846 | 2.9561 | 2.2838 |
1:2 | 2.6847 | 0.3064 | 3.0563 | 2.2875 | |
1:1 | 2.6911 | 0.3359 | 3.2357 | 2.3045 | |
2:1 | 2.6841 | 0.3097 | 3.0511 | 2.2891 | |
3:1 | 2.6614 | 0.2846 | 2.9548 | 2.2815 | |
1-Pentanol | 1:3 | 2.5803 | 0.2497 | 2.9039 | 2.2903 |
1:2 | 2.5904 | 0.2689 | 2.9728 | 2.2954 | |
1:1 | 2.6019 | 0.3087 | 3.1784 | 2.2988 | |
2:1 | 2.5901 | 0.2693 | 2.9734 | 2.2959 | |
3:1 | 2.5809 | 0.2502 | 2.9041 | 2.2908 | |
1-Heptanol | 1:3 | 2.5217 | 0.2138 | 2.8363 | 2.2915 |
1:2 | 2.5372 | 0.2349 | 2.9287 | 2.2974 | |
1:1 | 2.5405 | 0.2692 | 3.1329 | 2.2997 | |
2:1 | 2.5377 | 0.2354 | 2.9303 | 2.2979 | |
3:1 | 2.5221 | 0.2143 | 2.8375 | 2.2918 | |
1-Octanol | 1:3 | 2.4859 | 0.1827 | 2.8064 | 2.2938 |
1:2 | 2.4984 | 0.2039 | 2.8857 | 2.2963 | |
1:1 | 2.5096 | 0.2375 | 3.0664 | 2.2997 | |
2:1 | 2.4989 | 0.2042 | 2.8863 | 2.2975 | |
3:1 | 2.4861 | 0.1834 | 2.8071 | 2.2942 |
Values of dielectric relaxation time and molar free energies of activation (ΔFτ, ΔFη) for alcohols with methyl methacrylate (MMA) in benzene at 298 K
Alcohols | Molar ratio Alcohol:Ester | Relaxation time | ΔFτ (kJ mol–1) | ΔFη (kJ mol–1) | ||
τ(1) | τ(2) | τ(0) | ||||
ps | ps | ps | ||||
1-Propanol | 1:3 | 13.76 | 17.25 | 15.41 | 11.58 | 13.24 |
1:2 | 14.18 | 20.48 | 17.04 | 12.01 | 13.65 | |
1:1 | 16.01 | 27.38 | 20.94 | 12.72 | 14.26 | |
2:1 | 14.42 | 20.01 | 16.99 | 11.95 | 13.60 | |
3:1 | 13.83 | 17.41 | 15.51 | 11.60 | 13.27 | |
1-Pentanol | 1:3 | 16.36 | 21.88 | 18.92 | 12.17 | 13.91 |
1:2 | 17.28 | 24.01 | 20.37 | 12.40 | 14.23 | |
1:1 | 19.25 | 31.54 | 24.64 | 13.07 | 14.86 | |
2:1 | 17.36 | 24.03 | 20.43 | 12.40 | 14.25 | |
3:1 | 16.39 | 21.81 | 18.91 | 12.16 | 13.89 | |
1-Heptanol | 1:3 | 18.24 | 24.85 | 21.29 | 12.48 | 14.18 |
1:2 | 19.12 | 28.14 | 23.19 | 12.79 | 14.46 | |
1:1 | 21.80 | 37.16 | 28.46 | 13.48 | 15.19 | |
2:1 | 19.16 | 28.16 | 23.23 | 12.79 | 14.48 | |
3:1 | 18.28 | 24.85 | 21.31 | 12.48 | 14.23 | |
1-Octanol | 1:3 | 19.31 | 29.62 | 23.91 | 12.92 | 14.59 |
1:2 | 20.28 | 32.07 | 25.50 | 13.12 | 14.87 | |
1:1 | 22.58 | 39.59 | 29.90 | 13.64 | 15.42 | |
2:1 | 20.39 | 32.04 | 25.56 | 13.11 | 14.85 | |
3:1 | 19.40 | 29.56 | 23.95 | 12.91 | 14.56 |
Values of static dielectric constant (ε0), dielectric constant at angular frequency (ε∞), dielectric constant (ε′) and dielectric loss (ε″) for alcohols with ethyl methacrylate (EMA) in benzene
Alcohols | Molar ratio Alcohol: Ester | ε′ | ε″ | ε0 | ε∞ |
1-Propanol | 1:3 | 2.5885 | 0.2463 | 2.8984 | 2.2876 |
1:2 | 2.5928 | 0.2657 | 2.9519 | 2.2892 | |
1:1 | 2.6049 | 0.3058 | 3.1413 | 2.2916 | |
2:1 | 2.5932 | 0.2661 | 2.9523 | 2.2897 | |
3:1 | 2.5891 | 0.2473 | 2.8989 | 2.2894 | |
1-Pentanol | 1:3 | 2.5152 | 0.2169 | 2.8544 | 2.2919 |
1:2 | 2.5217 | 0.2354 | 2.9283 | 2.2928 | |
1:1 | 2.5303 | 0.2687 | 3.0562 | 2.2957 | |
2:1 | 2.5222 | 0.2359 | 2.9483 | 2.2931 | |
3:1 | 2.5158 | 0.2173 | 2.8558 | 2.2924 | |
1-Heptanol | 1:3 | 2.4568 | 0.1759 | 2.7737 | 2.2946 |
1:2 | 2.4686 | 0.1957 | 2.8498 | 2.2967 | |
1:1 | 2.4719 | 0.2158 | 2.9699 | 2.2984 | |
2:1 | 2.4653 | 0.1964 | 2.8405 | 2.2971 | |
3:1 | 2.4574 | 0.1765 | 2.7746 | 2.2952 | |
1-Octanol | 1:3 | 2.4259 | 0.1428 | 2.7042 | 2.2964 |
1:2 | 2.4434 | 0.1752 | 2.8067 | 2.2995 | |
1:1 | 2.4526 | 0.1983 | 2.9385 | 2.3019 | |
2:1 | 2.4441 | 0.1761 | 2.8097 | 2.2999 | |
3:1 | 2.4274 | 0.1434 | 2.7075 | 2.2976 |
It is also evident from the Tables 2, 4, 6, 8, 10, 12 that, the values of τ(2) are significantly higher than τ(1) and τ(0) for all the systems. Higher values of τ(2) indicate that the contribution of intermolecular or overall molecular relaxation is larger in comparison to intramolecular or individual molecular relaxation in the systems [10].
In the present systems studied, it has been observed that the relaxation time of ternary mixtures (alcohols with esters in solvent) is much greater than the binary mixture (alcohols with solvent or esters with solvent). This result indicates that, there is a hydrogen bond formation between the hydrogen atom in O–H group of alcohol and the oxygen atom in C=O group of ester and is shown in Fig. 1.
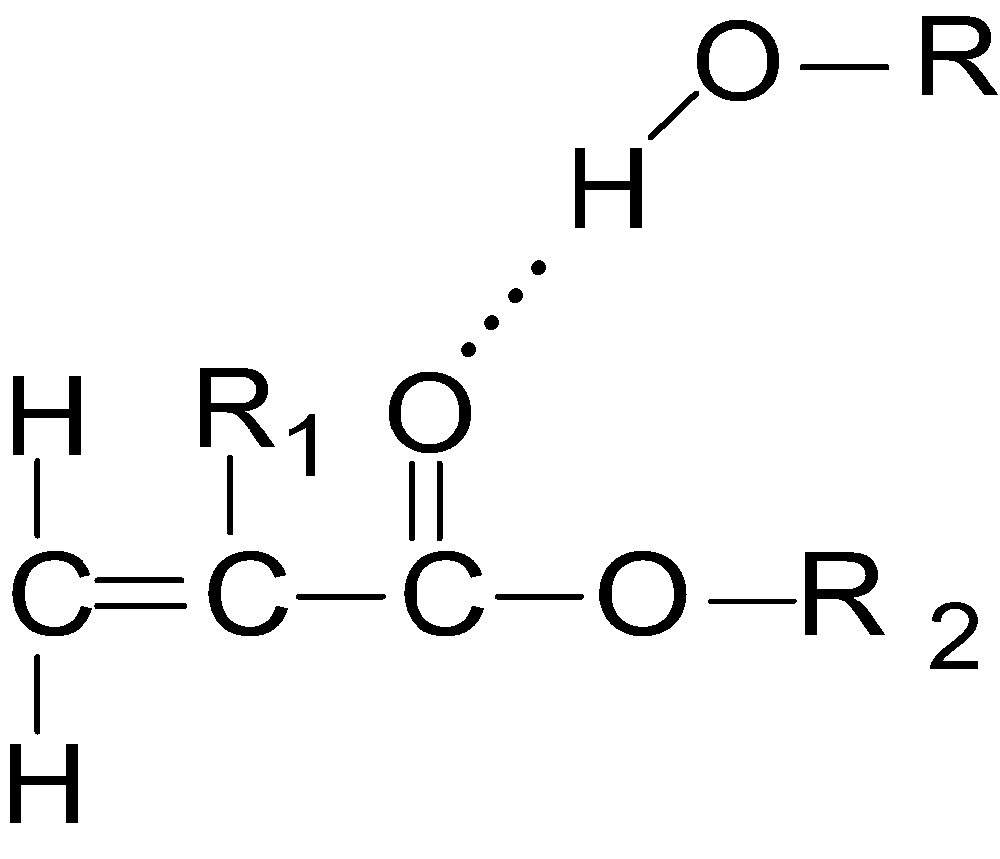
Hydrogen bonding interaction between alcohols and acrylic esters.
Where, R = C3H7 for 1-propanol;
R = C5H11 for 1-pentanol;
R = C7H15 for 1-heptanol;
R = C8H17 for 1-octanol;
R1 = CH3, R2 = CH3 for MMA;
R1 = CH3, R2 = C2H5 for EMA and;
R1 = CH3, R2 = C4H9 for BMA.
It is seen from the above same Tables that, the value of relaxation times (τ(1), τ(2) and τ(0)) increases with increasing chain length of both the alcohols and esters and offers hindrance to the rotation of the molecule. The increase in relaxation time may be due to two effects: (i) the increase of viscosity as the chain length increases and (ii) the increase of molecular size as the chain length increases [11].
From the Tables 2, 4, 6, 8, 10, 12, it is seen that the values of relaxation time in CCl4 are higher than benzene which shows direct proportionality with solvent viscosity. Therefore, the viscosity of the surrounding medium should have considerable effect on the dielectric relaxation time. Carbon tetrachloride is a symmetrical and a non-polar molecule. But each chlorine atom in this molecule is highly polarizable due to its three lone pairs of electrons and therefore it can function as an electron donor. There is, therefore, a possibility of interaction between the positive hydrogen of the hydroxyl group and a chlorine atom of the carbon tetrachloride molecule. The potential hydrogen bonding nature of the carbon tetrachloride molecule may therefore contribute to increasing the relaxation time [11,12].
It is evident from our data that the value of molar free energy of activation for viscous flow is greater than the free energy of activation for all the systems studied. This is in agreement with the fact that the process of viscous flow, which involves both the rotational and translational forms of motion, faced greater interference from neighbors than dielectric relaxation, which takes place by rotation only [13].
By a critical examination of Tables 2, 4, 6, 8, 10, 12, it is noticed that the energy value parameters ΔFτ and ΔFη is somewhat larger in carbon tetrachloride solution. One may say from this that carbon tetrachloride hinders the rotation of the solute dipoles in this solution much more effectively than benzene. Similar conclusions were also drawn by Madan [14].
Table 13 lists the formation constant (K) and the free energy change (ΔG0) values determined by Nash method [15] and Eyring's relation [16] for all the systems studied. The K and ΔG0 values for 1-octanol with all the proton acceptors (MMA, EMA and BMA) are found to be higher than that for 1-heptanol, 1-pentanol and 1-propanol with the same acceptors, which reveal that 1-octanol, have the greater proton donating ability than that of all other alcohols studied [17].
Formation constant (K) and free energy change (ΔG0) for alcohols with acrylic esters in different solvent systems at 298 K
Alcohol | Acrylic ester | K (l mol–1) | –(ΔG0) (kcal mol–1) | ||
CCl4 | Benzene | CCl4 | Benzene | ||
1-Propanol | MMA | 4.59 | 9.5 | 0.89 | 1.34 |
EMA | 5.0 | 10.0 | 0.95 | 1.37 | |
BMA | 6.0 | 10.4 | 1.06 | 1.39 | |
1-Pentanol | MMA | 6.07 | 11.6 | 1.06 | 1.46 |
EMA | 6.4 | 12.0 | 1.10 | 1.48 | |
BMA | 7.2 | 12.7 | 1.17 | 1.51 | |
1-Heptanol | MMA | 8.12 | 13.56 | 1.24 | 1.54 |
EMA | 8.93 | 14.34 | 1.29 | 1.57 | |
BMA | 9.51 | 15.05 | 1.33 | 1.60 | |
1-Octanol | MMA | 9.30 | 14.28 | 1.32 | 1.57 |
EMA | 10.05 | 15.06 | 1.36 | 1.60 | |
BMA | 10.84 | 15.91 | 1.41 | 1.63 |
For a given proton donor, the K and ΔG0 values for methyl methacrylate are found to be smaller than that of ethyl methacrylate and butyl methacrylate. This may be attributed to the difference in basicity of the alkyl groups, which varies in the order methyl < ethyl < butyl. This is because the negative inductive effect of the alkyl groups increases in the order methyl to butyl, and the electron contribution of the butyl group to the C=O group is significantly greater than that from the methyl group. Therefore, one would expect that the strongest intermolecular hydrogen bonds formed would be between the C=O group of butyl methacrylate and the OH proton of 1-octanol and the weakest between the C=O group of methyl methacrylate and the OH proton of 1-propanol [18].
It is evident from Table 3 that the formation constant and free energy change for benzene is higher than CCl4 for all the systems studied. It may be due to the local solvent interactions with the alcohols and esters in the order benzene > CCl4. The high values of K in benzene solutions suggests that, besides structure disruptions, the weak but specific interactions of –OH-π type between the hydroxyl group of alcohol and π-electrons of benzene and of n-π type between the carbonyl group of ester and π-electrons of benzene [19].
4 Conclusion
The hydrogen bonded complexes of alcohols (1-propanol, 1-pentanol, 1-heptanol and 1-octanol) and acrylic esters (methyl methacrylate, ethyl methacrylate and butyl methacrylate) have been studied in dilute solution of carbon tetrachloride and benzene using dielectric and FTIR spectroscopic methods. From this study, it may be concluded that the higher chain alcohols have relatively more tendency of complex formation than the lower chain alcohols and also the nature of solvents plays important role in the determination of strength of the hydrogen bond formation between the alcohol and acrylic ester.