1 Introduction
Recently, we discovered [1] that the salt of chemical composition hydronium tetra-chloroferrate of cucurbituril contains in its crystalline lattice a cyclic dihydronium ion of composition (). The cucurbituril molecule acts as a template trap for this unusual hydronium ion, as shown in Fig. 1. Fig. 2 shows the geometrical parameters of the dihydronium cations.
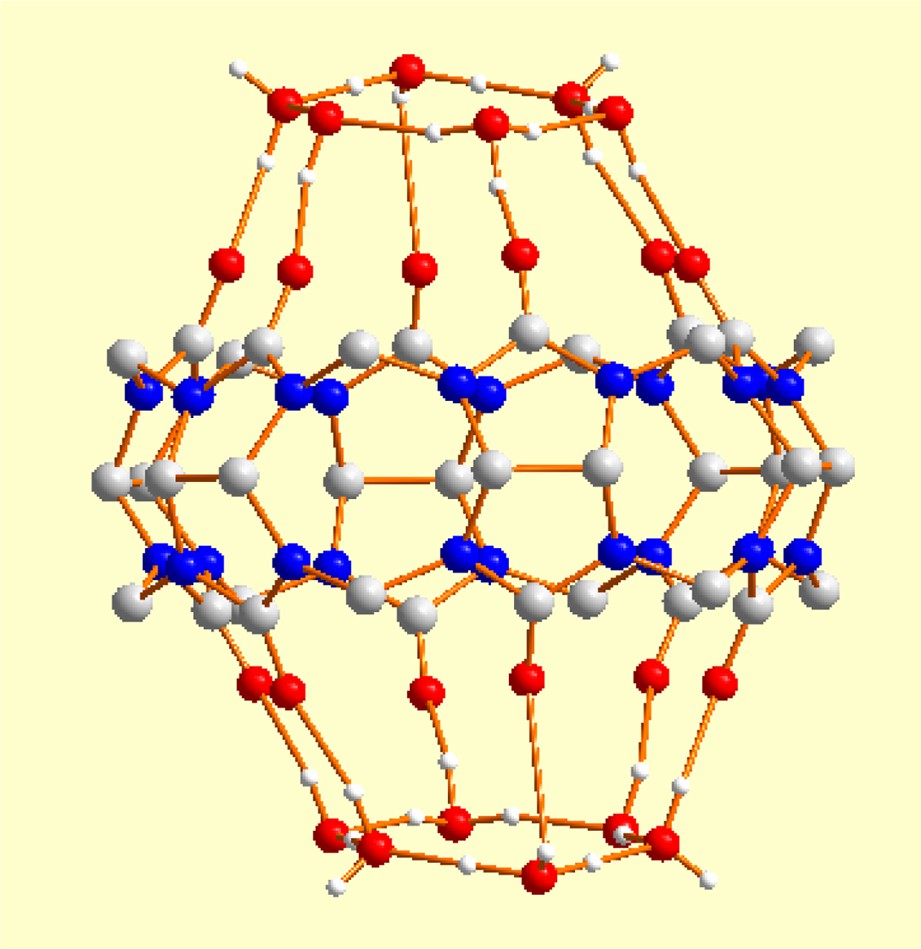
The central part of the figure is the cucurbituril molecule. Note the presence of six carbonyl oxygens in the upper and lower portions of the molecule. It is those rings of six carbonyl oxygens, on each side of the cucurbituril, that act as templates to trap the cations. The CSD REFCODE for this molecule is UCANOB [2].
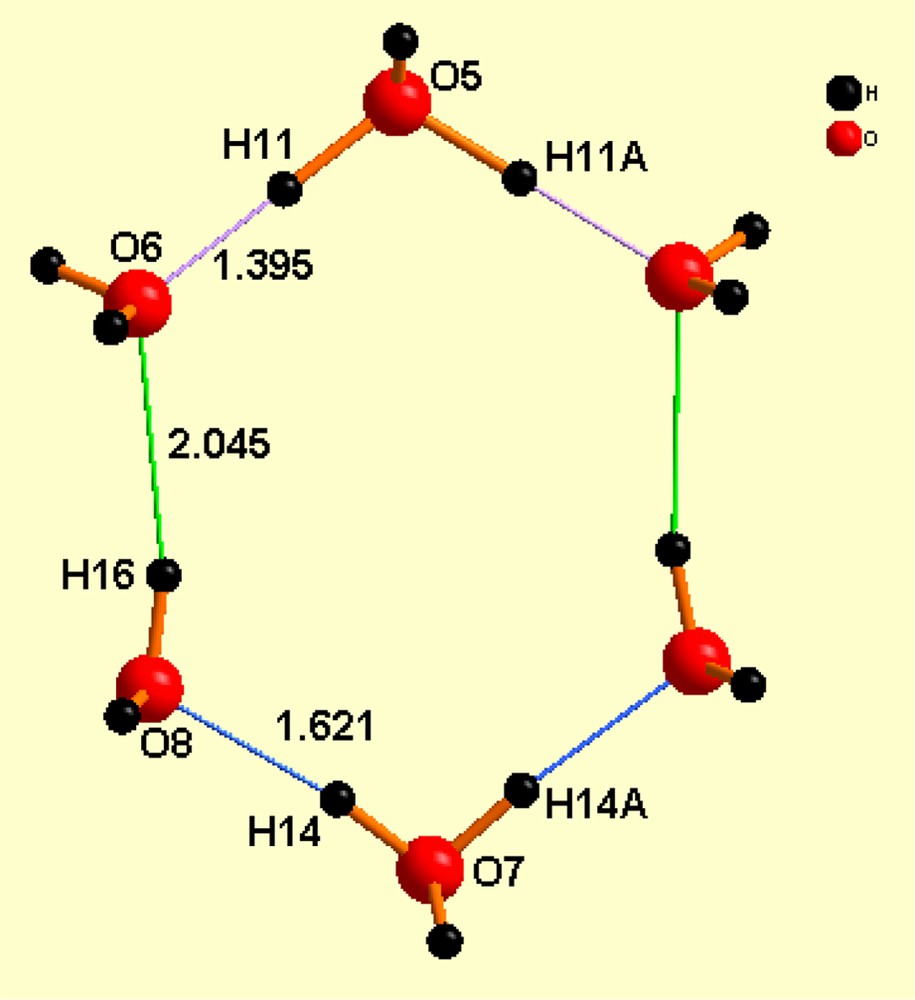
Distances within the cations, both of which are identical by symmetry. The remarkable aspects of these hydronium cations are (a) they bear a charge of 2+ and (b) they are planar within ± 0.007 Å, both characteristics being unheard of previously.
It seemed reasonable that there may have been additional, heretofore unrecognized, new species of the hydronium cations. Thus, a search of the Cambridge Structural Database [2] appeared warranted. It was carried as follows:
- ● using the search engine ConQuest (version 1.7) of CSD [2] the search was carried out with the graphic motif ‘H3O+’. Since no further compositional conditions were imposed on the search, the entire data base was searched, provided that (1) there was minor or no disorder in the hydronium ion itself; however, if there is disorder in a charge compensating ion, solvent molecule of crystallization or a molecule of a co-crystal, the example may have been used in this review providing that the hydronium cation had sensible stereochemistry. If the same hydronium cation was found in an ordered crystal, the one structure with disorder was ignored, at least for the graphics; though, it may have been mentioned in the text (2) minor errors in the hydrogen positions of the hydronium cation were, sometimes, accepted if the structure was determined by X-rays. For example, the CSD contains comments such as “short intermolecular hydrogens of the oxonium cation”. In some cases this was accepted, see below (3), there were no errors found in the search carried out by CSD [2] to evaluate stereochemistry, odd geometrical parameters or unusual thermal parameters (4) if the space group was corrected in a subsequent publication, and accepted by CSD, the latest entry was used (5) finally, no powder diffraction data were accepted since they would never produce accurate positions of hydrogen atoms, if at all;
- ● individually, every entry found was examined as a diagram and as a 3-D graphics figure using the Mercury routine of ConQuest. If the 3-D picture showed all the expected hydrogens, given the chemical formulation, the entry was saved. If it did not, and that was very frequently the case, it was rejected. Despite these precautions, many were rejected upon plotting a packing diagram; because, unfortunately, the CSD 3-D figures do not, always, show all of the waters of crystallization or hydronium ions if there are several. Thus, many more were rejected when plotted as described in the next paragraph.
The resulting data set, deemed acceptable, was saved and used for a more detailed analysis using the packing diagram routine of DIAMOND [3]. The results are classified into the following categories: (a) acyclic systems (these are further subdivided by isolated or condensed and by the number of oxygens in the cation). If there are geometrical isomers or conformers, these will be graphically illustrated (b) cyclic systems, classified by ring size and by charge, separately. Here, isolated and condensed systems will also be dealt with separately (c) highly condensed systems will be dealt separately, and last.
It has been assumed that if the structural data appeared in the CSD compendium, and passed all the additional test we imposed, that there are no seriously faulty structures in this review, unless they were identified as being so. However, that can never be guaranteed 100% because the newly discovered cyclic cations described here were all described as something else by the original reports. One of the problems with these analyses of structural data is that recognition of the correct structure is often obscured by being oriented in arbitrary directions that require a lot of persistent work to document properly. This point will be enlarged upon below. Hopefully, such a problem did not occur in preparing this review with the concomitant loss of an interesting new structural specimen. If I did, it is my fault and would appreciate hearing about it.
Note that, as in the case of waters of crystallization, hydronium ions are anchored to the parent species of the lattice by (a) hydrogen bonds to suitable Lewis bases, such as the oxygens of C=O, NO, NO2, carboxylates, –NH2 functional groups or (b) to Lewis acids such as carboxylate hydrogens, in which case the oxygen of the water or hydronium ion is the Lewis base donor. It is because of this feature that more complex species, such as (and larger hydronium ions) are either symmetrical or asymmetrical. In the former case, hydrogen bonding is equally strong on all directions. In the asymmetrical cases, the tug to one end is larger than the other and the hydrogen bond expands in the direction of the stronger tug. This aspect of the geometry of hydronium cations will be mentioned later, as it seems appropriate.
Finally, a jpeg file has been deposited with the Editor of this journal which contains all of the images appearing in this review plus some additional ones which were considered later to be redundant by virtue of the fact that they showed no additional information from that present in the selected examples. With copyright permission from the Editor, interested parties can request a copy of this file for related studies. The pictures appear in the colors of the hardcopy.
2 Part 1. Acyclic systems with isolated cations
2.1 H3O+
This smallest and most ubiquitous of all hydronium cations is recognized even in high school chemistry as the hydronium ion present in solution of strong acids in pure water. A picture of a crystalline lattice containing such species in shown in Fig. 3. The cations are isolated from one another, as discussed in the figure caption. The first word in all figure captions is the CSD REFCODE identification entry name (Fig. 4).

GOCJIR [4]. Isolated H3O+ cations present in this lattice. The shortest distance between them is 7.717 Å, far exceeding distances for acceptable hydrogen-bonded interactions. In this example, the hydronium cations are located in cavities formed by the organic molecule [N,N′-bis-(2-pyridylmethyl)malonic diamide] and the tetrafluoroborate charge compensating anions. Similar packing arrangements are found throughout the examples under consideration and is the origin of hydronium ions islation from each other, as well as the hydrogen-bonded interactions mentioned above.

PIWBOM [5]. Isolated H3O+ cations with waters nearby but at a distance of 4.254 Å which far exceeds the limit of hydrogen-bonded interactions. Note the cavities in the packing diagram, where the cobalt cations are located. The cobalt cations act as a template for H3O+ cations and waters. The figure illustrates the perils of locating hydrogens in X-ray diffraction studies. The authors list an H23? that makes appear the hydronium ions as H4O2+ cations. However, the H–OH23? angles are unacceptable; thus, suggesting that eliminating those atoms is justified. In this species, the problem with the hydronium ion hydrogens is probably the result of having disordered fluorines of the PF6– anion. This example is presented as an illustration of the problems encountered in putting together this review.
An example of a truly condensed system is that of the structure labeled MUKNOV [6]. As shown Fig. 5, it consists of strings of …H2O–H3O–H3O–H2O… Note, however, the caption on that figure.

MUKNOV [6]. The hydrogen bonds herein described are substantial: the two identical H3O–H3O bonds are 1.818 Å in length while the H3O–H2O are 1.869 Å. Somehow, this does not fit too well and, intuitively, I would have guessed that it would be the other way around due to Coulomb repulsion arguments. Perhaps this study should be repeated. This comment is accentuated by the note in the CSD entry that of the 20 waters described by this report, 18 are H2O; but, two appear to be a H3O+ and an OH–. Be as it may, the data provided by CSD leads to the structure shown here, which we consider suspect and provides another example of the perils of some the data in the literature.
As in the case of MUKNOV, Fig. 6 shows a condensed version of the H3O+ cation. But, whereas the strings in MUKNOV are finite and contain only pairs of H3O–H3O flanked by waters. Here, infinite strings of hydrogen-bonded H3O+ are held by two hydrogen bonds of slightly different lengths. It is remarkable that cations should form pairs of hydrogen bonds that bring them that close together (see figure caption). Normally, one would expect that Coulombic repulsion would preclude such an aggregation. The conclusion one must accept, unless the structure is incorrect, is that the hydrogen bonds to adjacent moieties [Fe(CN)63– anions] are sufficiently powerful to overcome the Coulombic repulsion forces between the H3O+ cations. The CSD document (IDABAQ) gives the name of the compound as “Tris(Bispentamethyl-cyclopentadienyliron)+ HexacyanoFe3–Dichloromethane Hexahydrate”. Caveat: all the oxygens appearing in the packing diagram are protonated; however, the oxygens labeled O2 have too many hydrogens. One of them was questioned by the authors who put a question (?) mark in its label. If that hydrogen is eliminated from the list, the drawing you get is than in Fig. 6. If correct, this is a very unusual species, and that is why it is presented here, in case it is correct, which I doubt. Readers may take that report cum grano salis since charge compensation arguments go against the formulation with H3O+ cations.

IDABAQ [7]. Note the two hydrogen bonds have lengths of 2.069 and 2.096 Å. These are substantial bonds, specially when viewed against the obvious Coulomb repulsions that must attempt to separate the H3O+ cations. Presumably, the string of hydronium cations are held ttogether the three negative charges of the hexacyanoferrate anions to which they are adjacent. A low temperature data set collected with a CCD instrument would be highly desirable in this case also.
(ESUDAX02), Fig. 7, depicts one of the strangest species I encountered in this survey. If correct, there is an infinite string of triply hydrogen-bonded H3O+ cations running down the c-axis of a hexagonal crystal. The cations are face-to-face to one another and equally spaced. The three identical hydrogen bonds they make (2.977 Å) to one another is near the limit of hydrogen bonds, assumed in this review to be 3.20 Å. Bizarre though this structure may seem, I believe the report is correct since (a) the data were collected with a modern CCD instrument at low temperature (three data sets were collected at 113, 130 and 250 K), (b) the ratio of independent observations to variable parameters is very favorable, (c) the R-factor and the weighted R-factor are 0.029 and 0.089, (d) the authors did not mention the possibility of twinning. The overall structure is shown in Fig. 7. The compound is extremely simple and rigid: it consists of a guanidium cation, two H3O+ cations and three NO3– anions. In fact, there is a similar situation in another hydronium structure within this survey. That is given below in the section on cyclic cations under the REFCODE of OBATAM (Fig. 17). In the case of OBATAM, the hydronium cations are also trapped in a cylindrical cavity that runs the entire length of the crystal (Fig. 8).

ESUDAX02 [8]. The string of hydrogen-bonded H3O+ cations is shown along the horizontal direction of the image. That is the c-axis of the hexagonal crystal. The three hydrogen bonds are identical, and that is why they are colored the same. The inter-cationic hydrogen bond length is 2.977 Å, which is on the upper limits of accepted hydrogen-bonded distances (3.20 Å), which makes sense given the expected Coulombic repulsions. However, the cations are surrounded by six oxygens of the nitrate anions and also form short O–H···O–N hydrogen bonds (see Fig. 8, and its caption).
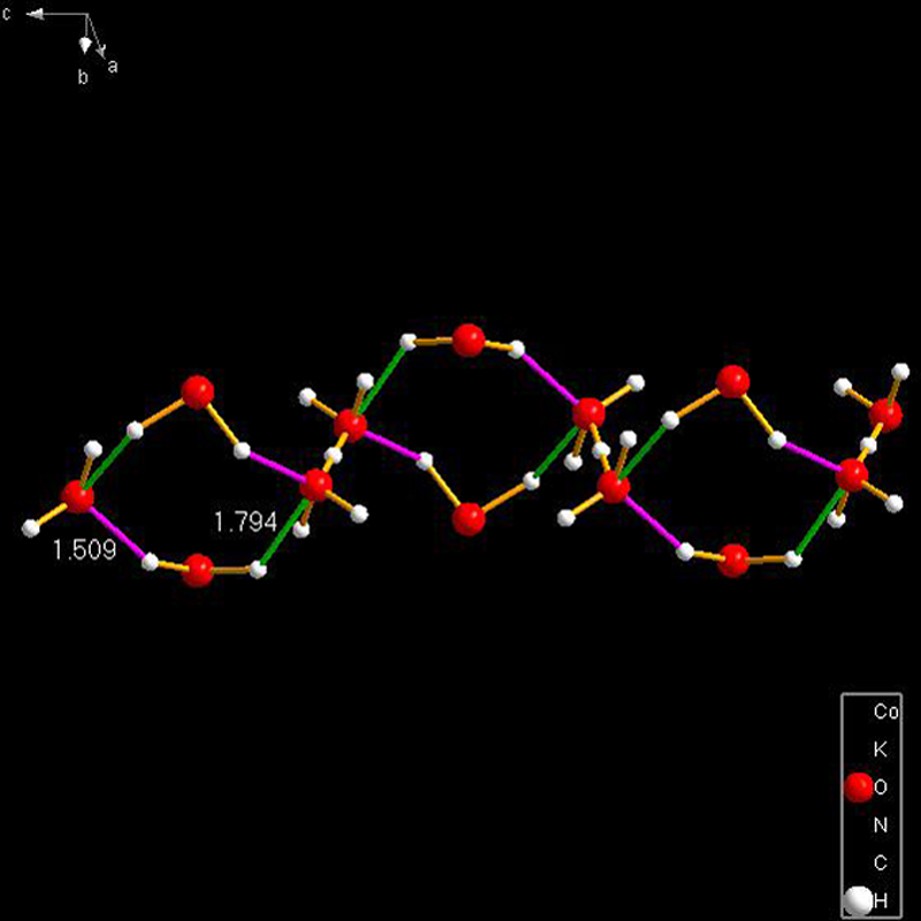
OBATAM [26]. This remarkable species consists of strings of beads of composition in which the beads are linked by a strong, symmetrical hydrogen bond of O–H–O = 0.796 Å. The string is extended for the entire length of the c-axis of a monoclinic lattice (C2/c, for details, see [26]). Please read the NOTE ADDED IN PROOF, at the end of the manuscript.

The complete packing diagram of ESUDAX, as ESUDAX01 [8]. Note that three nitrate anions surround each hydronium cation and form strong hydrogen bonds of length 1.613 Å, very substantial in nature. This is true of every hydronium ion present as can be documented by plotting the packing diagram with the c-axis as the vertical. Consequently, there is a significant level of charge compensation which, aided by the strength of hydrogen bonds, appears to be capable of handling the problem of inter-hydronium cationic repulsions.
Another, truly bizarre structure is that in Fig. 9 (WONLEQ) [9]. This species consists of a ring of eight hydrogen-bonded H3O+ cations – a fact that goes totally against all experience, if correct. A picture of this odd species is shown below.

WONLEQ [9]. As depicted, there are two pairs of identical hydrogen-bonded cations with hydrogen bond lengths of 1.922 and 2.104 Å. Another pair of cations is bonded asymmetrically with hydrogen bonds of 1.537 and 1.942 Å.
There are large numbers of isolated H3O+ cations in the literature. The ones described here are all those that are sufficient to cover all examples available. The rest are related to those described above but considered to be less desirable to use for illustrations.
2.2 systems
Fig. 10 shows an example of isomerism in hydronium ions of composition . Isomerism in this case refers to the fact that the hydronium ion and its attached water are linked by a bond whose length is a variable depending on the nature of the interaction between the other hydrogens and external objects to which they are attached. Moreover, as shown in this and subsequent figures, the planes of the terminal H–O–H fragments make different angles to one another. Again, this is due, no doubt, to the interactions of the terminal hydrogens tot the adjacent organic species with which the cations pack.
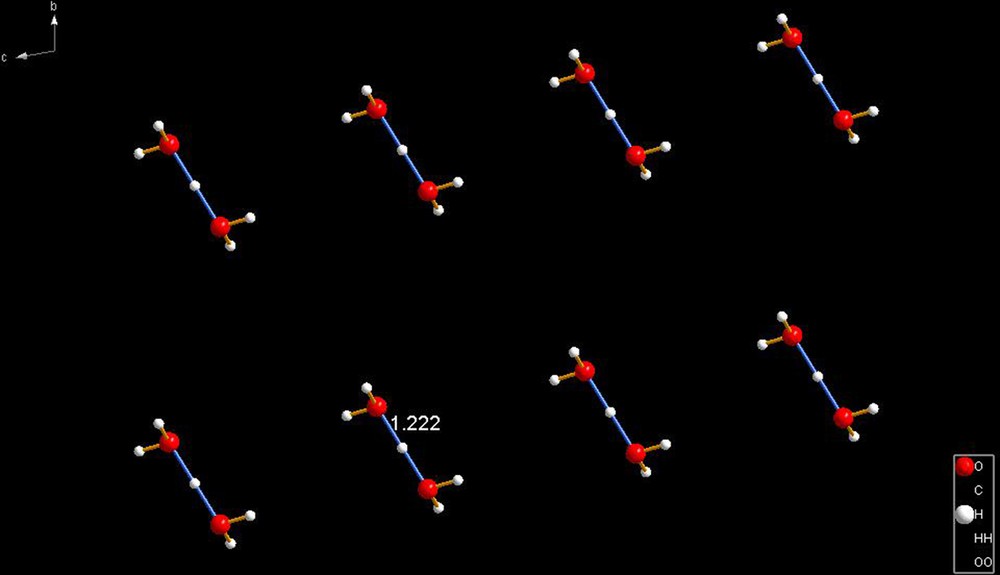
LUYWUX [10]. In this example, the hydrogen bond is exactly symmetrical, with both the O–H distances at 1.222 Å—a very short hydrogen bond, indeed. Another symmetrical hydrogen bond, and very short is QUQNUL [11a], with a perfect pair of 1.211 Å bonds, and another is KEZHOM [11b] with a perfect pair of 1.236 Å. In all these cases, the cations sit at special positions; that being the reason for the identical …O–H distances.
Other significant examples that illustrate the variability in length of the hydrogen bonds in species of composition are the following, with increasing length of the hydrogen bond:
- ● PICSUL [12], with 1.162 vs. 1.278 Å; CSD cites problems with intramolecular H-bonds in this case;
- ● TFMSAD01 [13], with 1.110 vs. 1.304 Å;
- ● SODFOG [14], with 1.141 vs. 1332 Å; disorder in the nitro group of the crown ether may be responsible for this result;
- ● NINDOD01 [15], with 1.087 vs. 1.390 Å; neutron diffraction study which may indicate precision in the positions of the hydrogens;
- ● NIZWAU [16], with 1.110 vs. 1.430 Å;
- ● VEXMIU [17], with 0.995 vs. 1.443 Å;
- ● NANNOF [18], with 1.075 vs. 1.547 Å;
- ● YEKLIJ [19], with 0.879 vs. 1.625 Å.
Using the above data, it seems there is a relationship between the pair of values but it is not quite true: the pair NEMSAD and NIZWAU, as well as VEXMIU destroy the pattern.
2.3 systems
In Fig. 11, we depict one of the examples having a symmetrical hydrogen-bonding scheme. Here, the two waters attached to the central H3O+ cation are related by a twofold axis, or nearly so, as is the case here. It is the central H3O+ cation that supplies the hydrogens for bond formation, while those of the outer waters are then free to bond to some anchoring species.

XUMKIF [20]. The two hydrogen bonds have nearly identical lengths of 1.553 and 1.556 Å, a difference of no more than one end; thus, mathematically insignificant.
SLBZAC10 [21] is a rather unusual form of the cation. The H3O+ cation occupies a terminal position, while two waters are attached to it asymmetrically, as depicted in Fig. 12. Therefore, symmetric-asymmetric isomerism is also observed for the cations, as was the case with the cations.
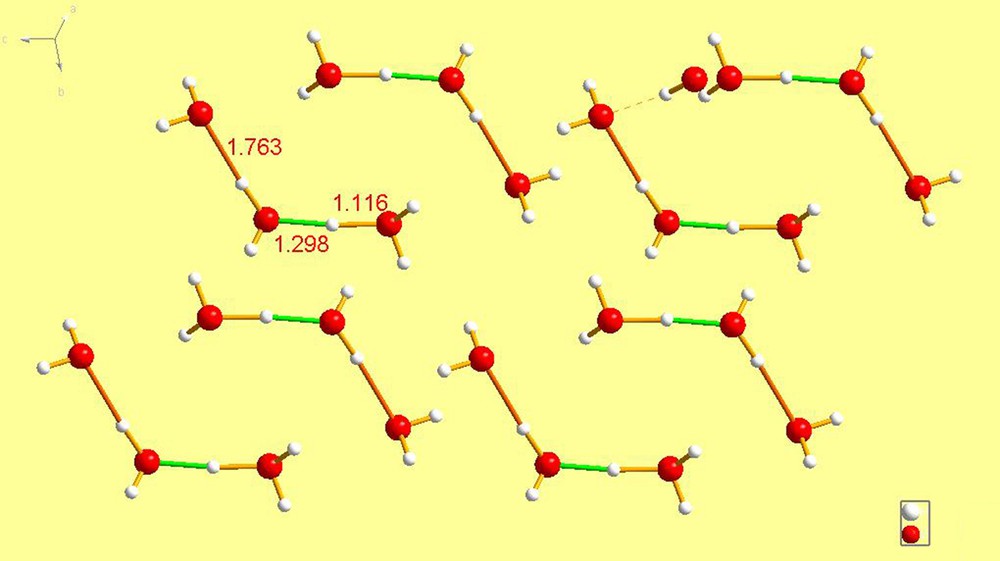
SLBZAC10 [21] has an H3O–H2O hydrogen bond in which the hydrogen is supplied, as expected, by the H3O+ moiety. It is an almost symmetrical system with bond lengths of 1.116 vs. 1.298. The two waters are linked to one another by a hydrogen bond of 1.763 Å; thus making this a truly asymmetric cation. Short intramolecular hydrogen contacts noted here also, no doubt due to the fact that this is an X-ray study.
2.4 systems
ASUHIF [22] is a classic of perfect symmetry. It can be described as a central cation flanked by two waters symmetrically arranged as shown in Fig. 13. The central part is so symmetrical that can readily be described as diaquated hydronium () cation.
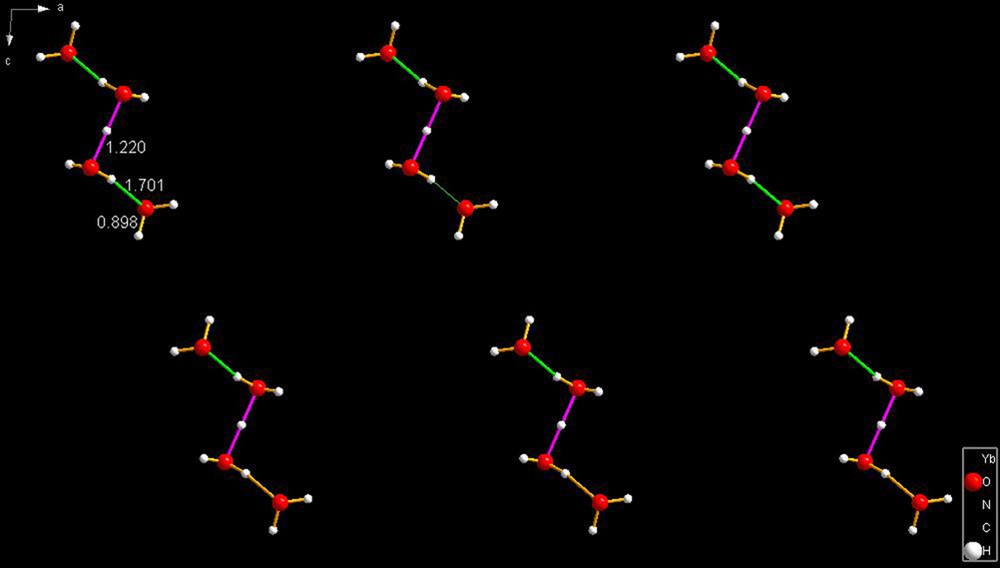
ASUHIF [22]. The central pair of hydrogen bonds have identical lengths of 1.220 Å while the outer hydrogen bonds are both identical at 1.701 Å.
JODJIV [23] is a completely different geometrical isomer of the cation since it contains a central H3O+ cation each of whose hydrogens has a water attached by a single hydrogen bond. The system has perfect threefold symmetry created by the fact that it lies on a threefold axis of a trigonal (P-3) space group.
Even though of different composition, the structures labeled WEWVEZ [24], WEWTOH [24] and WEWTUN [24] have identical structures for the cations present in their lattices. In turn, they are identical with those shown in Figs. 14 and 15. The difference is in the length of the hydrogen bonds: in WEWVEZ [24], WEWTOH [24] and WEWTUN [24] that distance is, respectively, 1.732, 1.711 and 2.013 Å. This illustrates very clearly the fact that the hydrogen bond length of hydronium cations is critically dependent on the nature of the attachments external to the hydronium ion components.
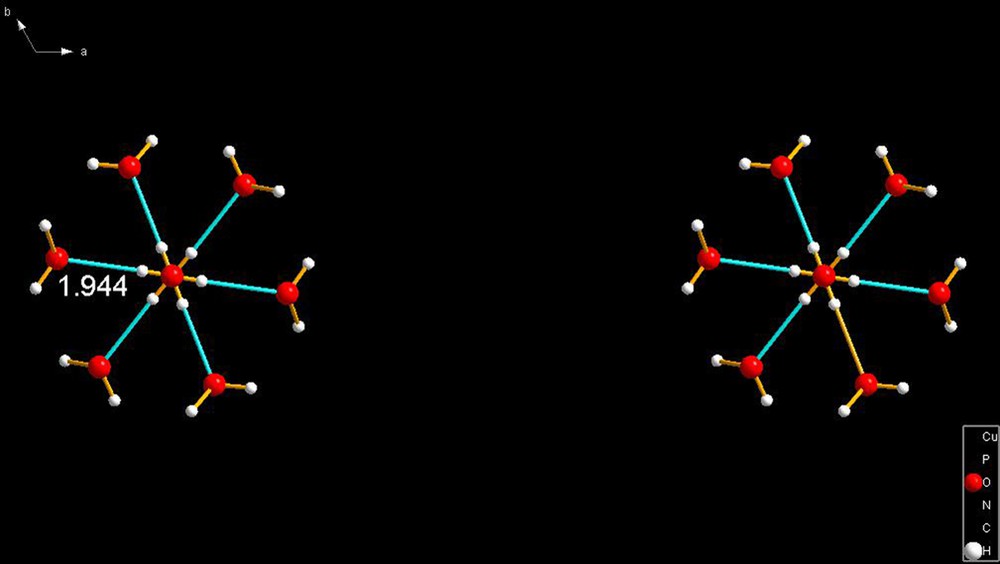
JODJIV [23]. In this view, the molecules were plotted as stick diagrams intended to show that (a) they have identical hydrogen bonds (b) that they are arranged in the lattice in a completely arbitrary direction which was convenient to properly display the cation (see Fig. 15 and caption).

JODJIV [23]. In this view, the molecules were plotted directly down the c-axis of the trigonal space group. The reason why there appears to be a hexagonal pattern is that a subsequent layer have trigonal molecules that are rotated 60° from those on either side of them; thus, creating the illusion that there are molecules with sixfold symmetry in the lattice.
3 Other species present in the solid state
IHEJOU [25] is a very interesting ion. Its composition is and can best be described as a tetrahydrated cation. The central species is symmetrical with an O–H distance of 1.173 Å. The waters are bound in pairs and asymmetrically as shown in Fig. 16.
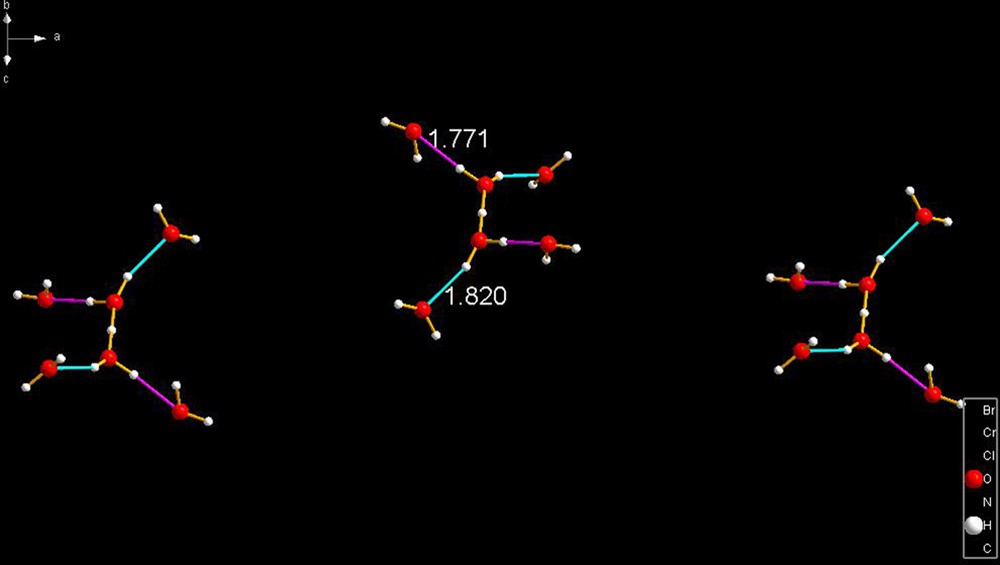
IHEJOU [25]. The waters are bound to the central species (a cation) in pairs having bond lengths of 1.771 and 1.820 Å, again corroborating the fact that these cations have geometrical features that depend on the nature of their binding to external entities that surround them in those crystalline lattices.
4 Additional cyclic hydronium cations
As stated in Section 1, the motivation for this study was the discovery of a cyclic hydronium of composition . A methodical search of the CSD resulted in identifying additional cyclic hydronium cations, including one from our own work and which had not properly been described – a shameful neglect on our part, which we now correct with the description of OBATAM [26], below.
BIKVIA10 [27] contains a cyclic hydronium cation of composition which is, in fact, identical with that present in UCANOB [1], as shown in Fig. 18, below.
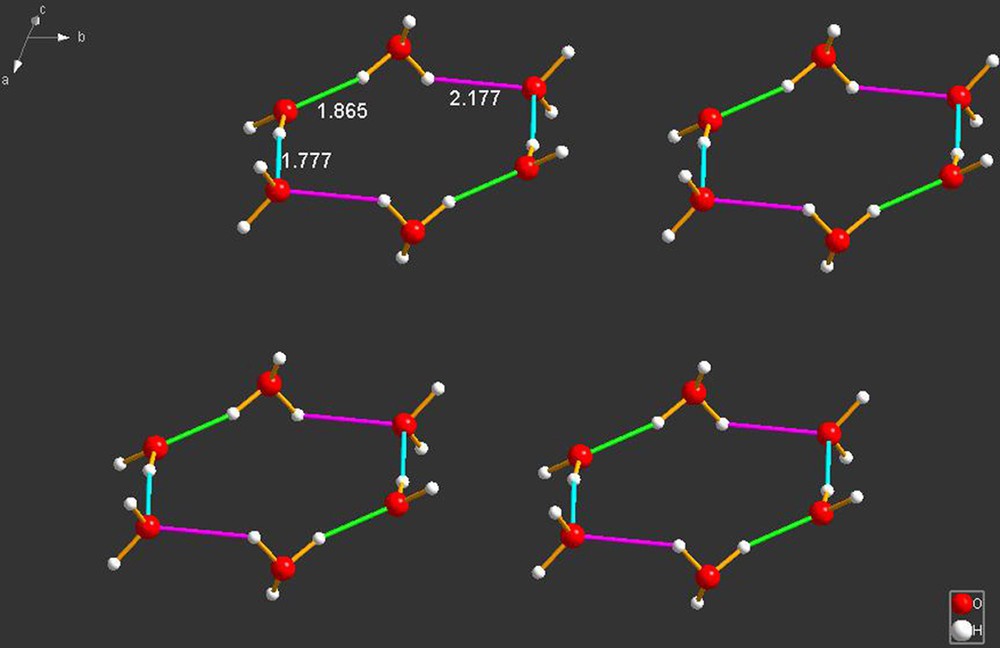
BIKVIA10 [27] contains two trans-H3O+ hydronium ions linked by water pairs, forming a six-membered ring exactly as in UCANOB [1]. As shown, the hydrogen bonds are 1.777, 1.865 and 2.177 Å in length; therefore the bonding is considerably different from that of UCANOB, where the relevant bonds are 1.395, 1.621 and 2.045 Å. It is interesting to note that the molecule present in BIKVIA contains a (O2S)–N–(SO2) fragment whose –SO2 oxygens form hydrogen bonds with the cyclic hydronium cation in a fashion reminiscent of that found in UCANOB. Interestingly, Goldberg [27] also investigated the co-crystal of the same sulfonimide with acetic acid. In that case, the hydronium ion present is and it is also held by hydrogen bonds with the –SO2 oxygens. Thus, sulfonamides appear to be useful as potential generators of inclusion compounds in which hydrogen bonds play a major role.
Finally, we come to RABCOI [30] (Figs. 19 and 20). This is a truly unusual species which can best be described as infinite, parallel, sheets of waters whose motifs are six membered rings, as shown in Fig. 21. As shown in that figure, these sheets are linked to one another by hydronium cations. Another demonstration by Mother Nature of her infinite resourcefulness at creating stereochemically entrancing molecular and ionic entities.

LOHJOH [28] consists of a ring of eight waters that has two waters external to the ring and held by hydrogen bonds of 1.736 Å. The internal bonds of the ring are in pairs, as emphasized by the color scheme, and those bonds have lengths of 1.659, 1.906 and 2.284 Å.
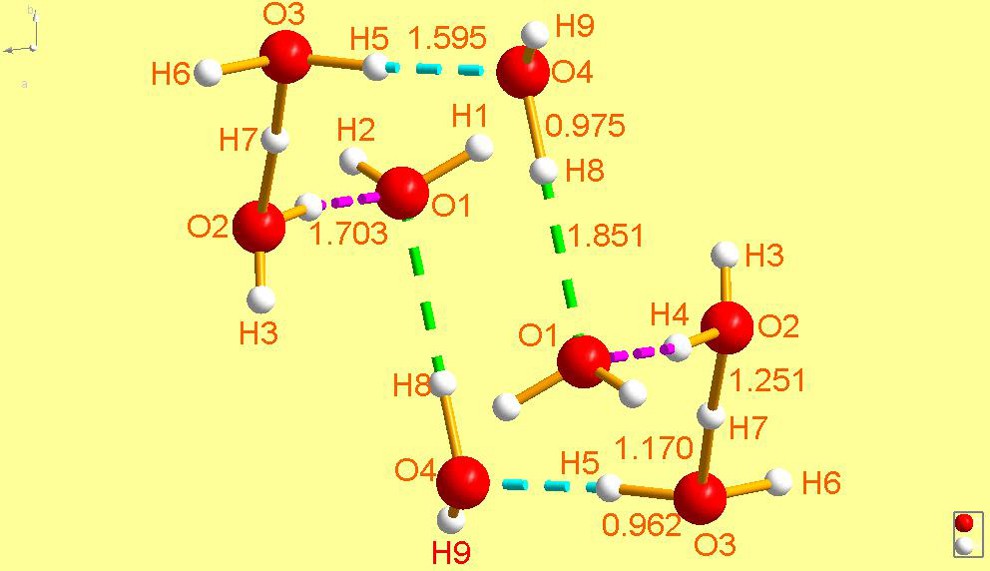

FEGTEC [29] is an extremely interesting cyclic structure consisting of a ring of eight waters, two of which are protonated and are trans to each other – the overall composition is . The ring is in a chair conformation and resembles closely the structure of cyclo-octane. It joins UCANOB [2] as the (so far) two cyclic species which are also bear a charge of +2. And, as was the case with UCANOB [2], this cation was also trapped by an organic species, in this case an aromatic sulfonate Moreover, Hanson reported in the same paper [29] that the same organosulfonate also traps a non-cyclic hydronium cation of composition (FEGTEC01) [29], which is not structurally related to FEGTEC. In fact, it is very close in stereochemistry to that found in JODJIV [23], depicted in Fig. 14, the difference being that JODJIV [23] has perfect trigonal symmetry (sits at a threefold axis), whereas FEGTEC01 [29] does not.

The structure of RABCOI [30] is difficult to see clearly unless one orients the motif correctly, as shown here. In this orientation one can see the linking hydronium cations binding together adjacent water sheets. The central portion of the cations is symmetrical and the two O–H bonds have lengths of 1.378 Å. However, the links to the water sheets is not symmetrical, having O–H distances of 1.746 and 1.879 Å. The amount of matter shown in this view has been reduced in order to avoid what would be serious overlap of atoms.
5 Conclusions
It has been my pleasure to research this topic and present it in textual and graphical form. It is my hope that it is useful to other scientists and that it becomes a catalyst for renewed efforts in the direction of stereochemical studies of these enormously important cations. It is also my hope that the review encourages researchers to check on the accuracy of some of the more bizarre aspects of the stereochemistry of the cations herein reported on. I have done my best point out that an effort was made (a) to weed out bad structural data, as described in Section 1, (b) to use those reports only if they passed those stringent conditions, (c) to accept those that passed such tests; however, I have also commented on my skepticism on some of the structures herein described. Hopefully, someone will redo those structures using CCD equipment and at low temperatures. If, indeed correct, there will have to be some major shifts in our thinking about what hydronium cations can and cannot do in terms of composition and stereochemistry. Finally, in the future, one has to consider doing an analysis of the substrates to which these hydronium ions are attached. It seems reasonable to assume that they influence strongly the final results. In other words, the pieces are present in aqueous solutions (e.g. protons and waters) how they end up the way they do, in crystals, is likely to be dictated by the composition and stereochemistry of the entities they are moored onto.
NOTE ADDED IN PROOF: Just prior to submitting the revised version of this manuscript, the compound OBATAM [26] was prepared again. The synthesis is straight-forward but the crystallization is not because the solution becomes fairly thick and, suddenly, the potassium-hydronium compound crystallizes out. If one allows the crystals to remain in the mother liquor a mixture of crystals of the potassium-hydronium crystals(thick) and of the di-potassium salt(thin) result. These can be separated mechanically with little difficulty and used for X-ray studies. A preliminary re-examination of the OBATAM [26] crystals (CCD, 100 K) is currently under way and the results will be announced upon completion of that study.