1 Introduction
One decade ago, in a paper entitled “TTF[Ni(dmit)2]2: now as thin films and nanowires”, we described the preparation of the first nano-objects (i.e. nanowires) of this well-known metal complex-based superconductor (TTF = tetrathiafulvalene, dmit2− = 1,3-dithiole-2-thione-4,5-dithiolato, Fig. 1) [1]. Nanowires were grown on a nanorough silicon surface by adsorption through dipping of the substrate into precursor solutions. The silicon surface presented nanoreservoirs in which the growth of the TTF[Ni(dmit)2]2 phase was initiated. The growth continues as nanowires given the 1–D character of this material due to the stacking of both TTF and [Ni(dmit)2] moieties along the (010) direction [2]. Despite of their 1–D character, molecule-based conductors could be prepared as roughly spherical micrometer-sized grains by electrochemical ways on a flat electrode [3]. However, processing them as particles exhibiting diameters in the nanometer range remained as a challenge. Recently, we succeeded in the preparation of TTF·TCNQ nanoparticles showing a diameter of about 40 nm (TCNQ = tetracyanoquinodimethane) [4]. They were grown by adding TCNQ to TTF in the presence of an imidazolium-based ionic liquid, this latter controlling the growth of the TTF·TCNQ phase as nanospheres. In this communication, we describe the preparation of well-dispersed TTF[Ni(dmit)2]2 nanoparticles grown in the presence of an ionic liquid (abbreviated as IL), namely [BMIM][N(CF3SO2)2] (BMIM = 1-butyl-3-methylimidazolium) or [(CH3)(n-C8H17)3N][Cl].
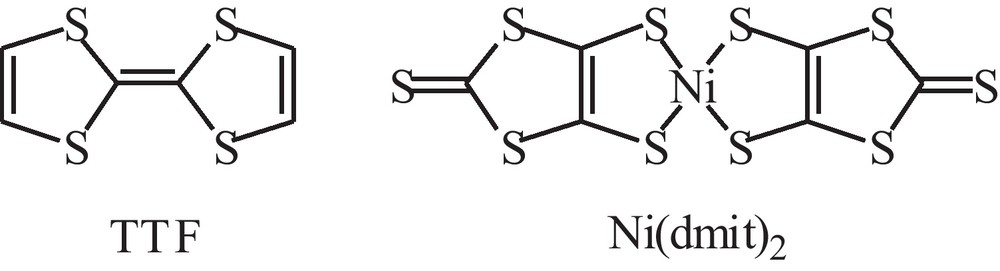
Molecular formulas for TTF and Ni(dmit)2.
2 Results
The dropwise addition of (TTF)3(BF4)2 in acetonitrile/IL into an acetone solution of [(n-C4H9)4N][Ni(dmit)2] at–80 °C leads to a black precipitate. Whatever the ionic liquid used ([BMIM][N(CF3SO2)2], 20 eq./TTF or [(CH3)(n-C8H17)3N][Cl], 4.5 eq./TTF), infrared spectra and X-ray diffraction patterns of the precipitate are identical. Infrared spectra confirm the presence of both TTF and Ni(dmit)2 moieties within the powder (Fig. 2). Peaks at 3081(w) and 819(m) cm−1 are respectively assigned to CH and CS stretching vibrations in TTF [5]. The band located at 509(m) cm−1 corresponds to the symmetric stretching vibration of the two carbon-sulfur single bonds in the S2C = S fragment of dmit [6]. The characteristic doublet present in all compounds containing the M(dmit)2 moiety, displays maxima at 1050(m) and 1070(s) cm−1, respectively. The higher frequency peak of this doublet (1070 cm−1) is assigned to the stretching vibration of carbon-sulfur double bond in the terminal S2C = S fragment of dmit, while the lower one (1050 cm−1) corresponds to the Fermi resonance peak between overtones of symmetric stretching vibration of two carbon-sulfur single bonds in the S2C = S fragment of dmit and the higher frequency [6]. Furthermore, several intense features in the 1240–1340 cm−1 range are the vibronic C = C bands resulting from electronic-molecular vibration (e–mv) couplings [7]. X-ray diffraction patterns are in good agreement with the pattern calculated from single crystal data of TTF[Ni(dmit)2]2 (Fig. 3) [8]. The calculated pattern allows indexing all the experimental diffraction signals. The resulting cell parameters: a = 4.621(6); b = 0.3728(4); c = 2.279(2) nm; β = 119.17(1)° are in excellent agreement with those obtained from single crystal X-ray diffraction study: a = 4.621(1); b = 0.3728(3); c = 2.2819(6) nm; β = 119.24(2)°, thus confirming unambiguously the chemical nature of the phase. For the two samples described in the experimental section, electron micrographs evidence well-dispersed roughly spherical nanoparticles (Fig. 4). Corresponding histograms of size are shown on Fig. 5. Mean diameters are calculated to be 17 and 27 nm for the samples prepared in the presence of [BMIM][N(CF3SO2)2] and [(CH3)(n-C8H17)3N][Cl], respectively.
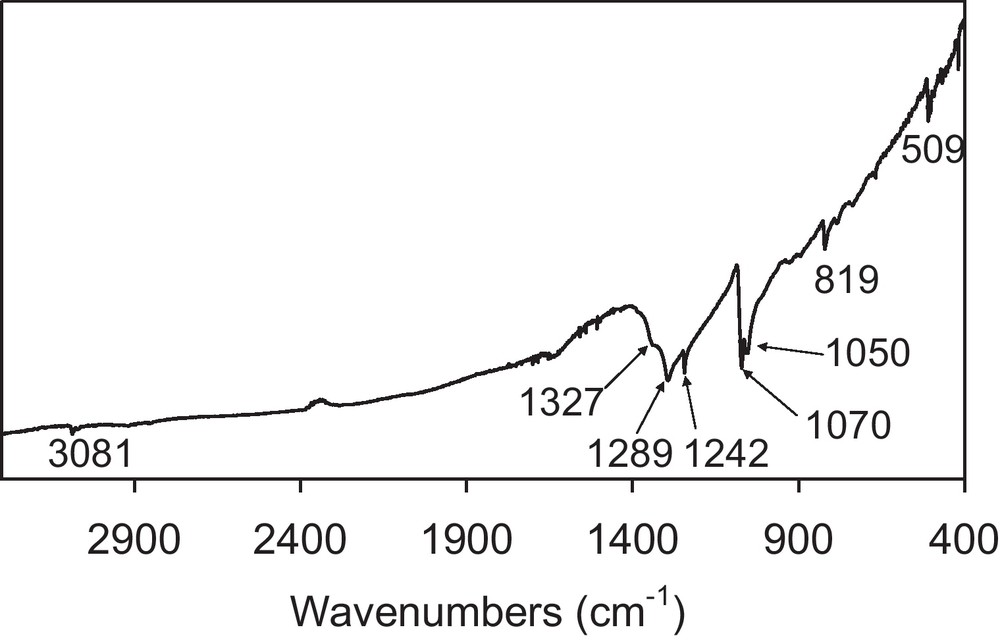
Infrared spectrum for TTF[Ni(dmit)2]2 nanoparticles prepared in the presence of [BMIM][N(CF3SO2)2].
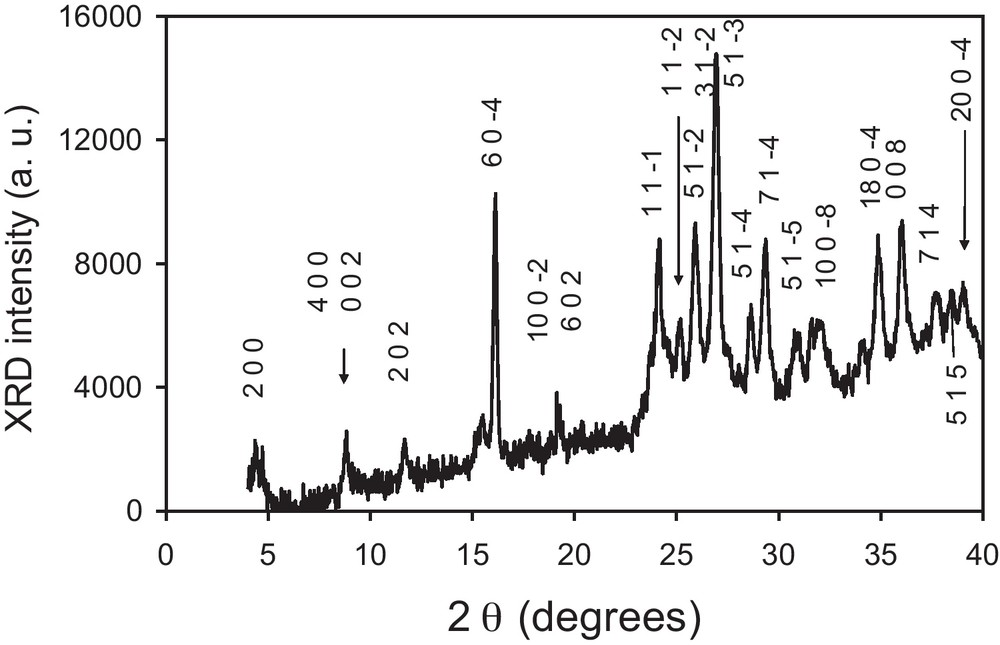
X-ray diffraction pattern for TTF[Ni(dmit)2]2 nanoparticles prepared in the presence of [BMIM][N(CF3SO2)2].
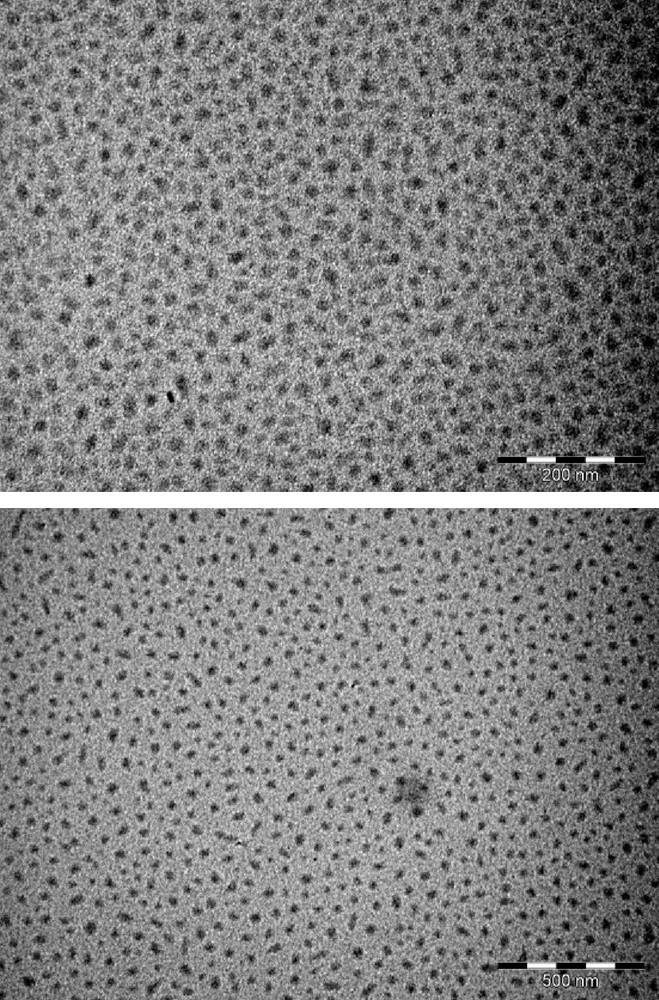
Electron micrographs for TTF[Ni(dmit)2]2 nanoparticles prepared in the presence of [BMIM][N(CF3SO2)2] (top, bar = 200 nm) or [(CH3)(n-C8H17)3N][Cl] (bottom, bar = 500 nm) at −80 °C.
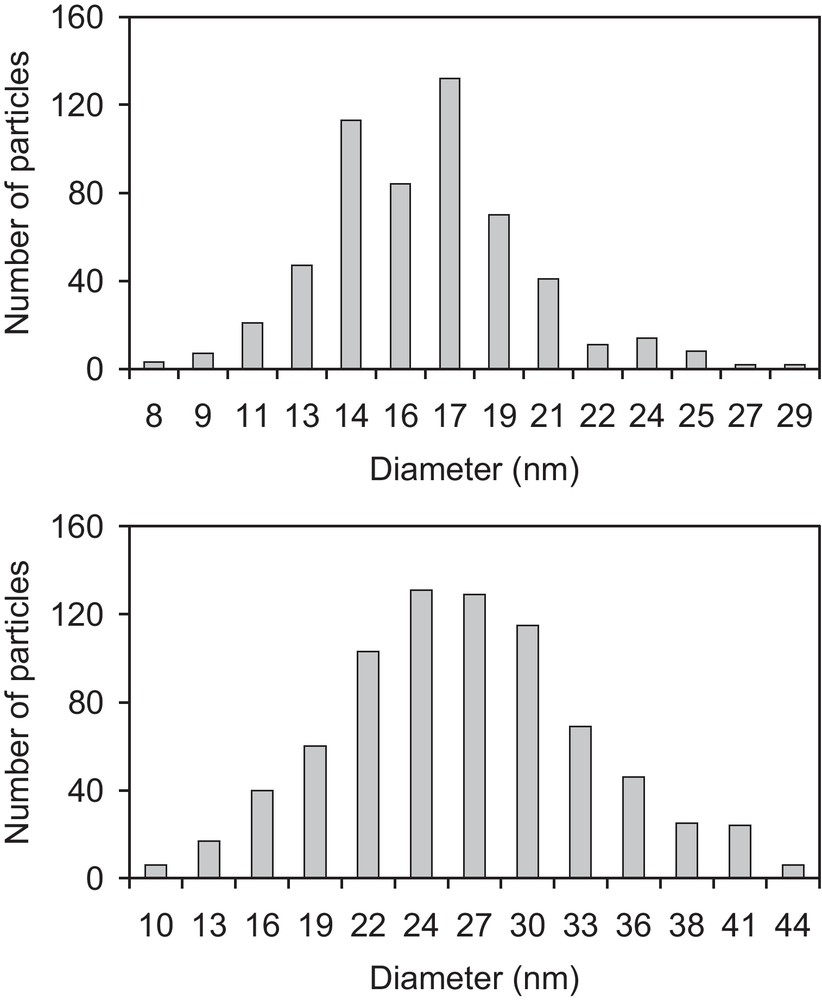
Histograms of sizes for TTF[Ni(dmit)2]2 nanoparticles prepared in the presence of [BMIM][N(CF3SO2)2] (top) or [(CH3)(n-C8H17)3N][Cl] (bottom) at −80 °C.
3 Discussion
The slow interdiffusion of saturated solutions of (TTF)3(BF4)2 and [(n-C4H9)4N][Ni(dmit)2] leads to needle-like TTF[Ni(dmit)2]2 crystals [2]. At ambient pressure, the room temperature conductivity of TTF[Ni(dmit)2]2 measured along the needle axis is of about 300 S.cm−1, which is a quite high value (the needle axis is parallel to the (010) stacking direction of TTF and Ni(dmit)2 moieties) [8]. This compound exhibits a metal-like conducting behaviour down to 4 K [8]. Its conductivity increases which pressure and it undergoes a complete transition to a superconducting state at 1.62 K under a hydrostatic pressure of 7.5 kbar [9].
When performing the slow diffusion of a saturated solution of (TTF)3(BF4)2 into a [(n-C4H9)4N][Ni(dmit)2] solution in the presence of an ionic liquid, namely, [BMIM][N(CF3SO2)2] or [(CH3)(n-C8H17)3N][Cl], we observe the formation of a fine black precipitate. For a molar ratio IL/TTF of about 20 (value comparable to that for the growth of well-dispersed TTF·TCNQ nanoparticles [4]), infrared spectra are ionic liquid-dependent. The infrared spectrum of the powder grown in the presence of [BMIM][N(CF3SO2)2] confirms that the precipitate corresponds to the TTF[Ni(dmit)2]2 phase (Section 2). The infrared spectrum of the powder obtained in the presence of [(CH3)(n-C8H17)3N][Cl] (IL/TTF = 20) does not show the characteristic doublet assigned to the Ni(dmit)2 entity (at 1050 and 1070 cm−1, respectively). However, it evidences peaks at 3073(w) and 816(m) cm−1, respectively assigned to CH and CS stretching vibrations for TTF [5]. Moreover, the X-ray diffraction pattern of this latter sample is in relatively good agreement with the pattern calculated using single crystal data of TTFCl0.77 [10]. Therefore, in the presence of a large excess of [(CH3)(n-C8H17)3N][Cl] (20 eq./TTF), the reaction takes place preferentially between tetrathiafulvalene and chlorine anions leading to the mixed valence TTFCl0.77 salt. For avoiding the formation of this mixed valence salt, we have performed the slow diffusion of a (TTF)3(BF4)2 solution into a [(n-C4H9)4N][Ni(dmit)2] solution in the presence of about 4.5 eq. [(CH3)(n-C8H17)3N][Cl] vs. TTF. Using these experimental conditions, the TTF[Ni(dmit)2]2 phase is grown (see the X-ray diffraction study below) preferentially to the TTFCl0.77 phase.
X-ray diffraction patterns of the powders grown in the presence of [BMIM][N(CF3SO2)2] (20 eq./TTF) or [(CH3)(n-C8H17)3N][Cl] (4.5 eq./TTF) are identical (Fig. 3). They are recorded for 2θ values in the 3 − 50° range. The X-ray diffraction pattern confirms the monoclinic lattice (C2/c space group) of TTF[Ni(dmit)2]2 with parameters (a = 4.621(6); b = 0.3728(4); c = 2.279(2) nm; β = 119.17(1)°) very close to those obtained from the crystal data study (a = 4.621(1); b = 0.3728(3); c = 2.2819(6) nm; β = 119.24(2)°) [8]. All diffraction peaks can be indexed (Fig. 3). For TTF·TCNQ powders prepared in the presence on an imidazolium-based ionic liquid, X-ray diffraction patterns evidence a preferential orientation of the crystallites, (002), (004), and (008) peaks being much more intense than the others [11]. For TTF[Ni(dmit)2]2 powders prepared in the presence of an ionic liquid, no such preferential orientation seems to be observed.
When the slow interdiffusion of solutions of (TTF)3(BF4)2 and [(n-C4H9)4N][Ni(dmit)2] in the presence of [BMIM][N(CF3SO2)2] (20 eq./TTF) or [(CH3)(n-C8H17)3N][Cl] (4.5 eq./TTF) is performed at room temperature, electron micrographs evidence agglomerates of particles in which sizes of individual particles are difficult to measure. When the same reaction is carried out at −80 °C, electron micrographs show well-dispersed TTF[Ni(dmit)2]2 nanoparticles (Fig. 4). Nanoparticles grown in the presence of the imidazolium-based ionic liquid exhibit diameters in the 8–30 nm range (mean diameter: 17 nm). Those grown in the presence of the ammonium-based ionic liquid present diameters in the 10–45 nm range (mean diameter: 27 nm). For TTF·TCNQ nanoparticles prepared in the presence of [BMIM][BF4] [12] or octylamine [13] or for TTFCl0.77 nanoparticles electrochemically grown with [(CH3)(n-C8H17)3N][Cl] as supporting electrolyte [14], infrared spectra all exhibit bands of [BMIM]+, octylamine, or [(CH3)(n-C8H17)3N]+, respectively. The nanoparticles then consist of a TTF·TCNQ or a TTFCl0.77 core surrounded by a shell of imidazolium salt, octylamine, or ammonium salt, acting as a stabilizing agent. In the TTF[Ni(dmit)2]2 case, infrared spectra do not show the vibration bands corresponding to the imidazolium or ammonium cation. This is confirmed by elemental analyses which are in good agreement with the TTF[Ni(dmit)2]2 compound (theo.: %C 19.53, %H 0.36; exp.: %C 20.02, %H 0.29). No significant nitrogen amount (present in both imidazolium and ammonium salt) is detected. The imidazolium or the ammonium salt plays a crucial role in controlling the growth of the TTF[Ni(dmit)2]2 phase as nanoparticles but does not adsorb at the particle surface. Ionic liquids are well known to control the growth of metal nanoparticles (obtained by decomposition of an organometallic precursor) and to serve as dispersive medium for them [15]. In reference [15], the authors describe the preparation of ruthenium nanoparticles in pure [BMIM][N(CF3SO2)2] at various temperature without stirring. At room temperature, Ru nanoparticles are bigger (and somewhat agglomerated) than those at low temperatures (well-dispersed and exhibiting an extremely narrow size distribution). Indeed, the ionic liquid organizes in nanodomains at low temperatures in which the confinement of Ru nuclei should be more efficient and afford smaller ruthenium particles. In the TTF[Ni(dmit)2]2 case, even if the reaction takes place in a mixture IL/conventional solvents (acetonitrile and acetone), agglomerates are observed at room temperature whereas well-dispersed small particles are favoured at low temperatures. Nanodomains would also exist at –80 °C but would be differently structured in the presence of a co-solvent (acetonitrile or acetone).
The broad absorption over the entire infrared spectrum of the TTF[Ni(dmit)2]2 nanoparticle powders (Fig. 2) is due to a charge transfer (CT) transition, well-known for organic conductors [7]. This bodes that, in the powder, which is actually made of nanoparticles, conduction paths do exist. Indeed, the room temperature conductivity of TTF[Ni(dmit)2]2 nanoparticle powders measured on a compressed pellet is about 10 S cm−1, a relatively high value. Bulk TTF[Ni(dmit)2]2 powders exhibit a room temperature conductivity of 50 S cm−1 [2]. These two values are of the same order of magnitude. The conductivity of a compressed pellet of a powder sample is the result of two main contributions: the conductivity of the grains themselves and that of boundaries between them. A third contribution should be the conductivity of the shell of stabilizer at the surface of grains. This latter significantly lowers the conductivity value for octylamine or ammonium-stabilized nanoparticles [14,15]. In the TTF[Ni(dmit)2]2 case, the absence of stabilizing agent at the particles surface would explain the quite high conductivity value. Finally, TTF[Ni(dmit)2]2 nanoparticle powders exhibit a semiconducting behavior in the 77–295 K range, which is not surprising for powdered materials. Their activation energies are about 30 meV.
In conclusion, we have prepared well-dispersed roughly spherical nanoparticles of the metal-organic superconductor TTF[Ni(dmit)2]2. It was not a priori obvious for a quasi-monodimensional system having no extended coordination bonds, as for Prussian Blue magnets (via CN ligands) which are known to be easily grown as nanoparticles exhibiting sizes as low as 2 nm [16]. Working in ionic liquid/conventional solvent mixtures and at low temperatures, particles of mean diameters lower than 30 nm are obtained. We are currently investigating the use of other solvents and liquid surfactants (in various relative amounts) in which TTF[Ni(dmit)2]2 nanoparticle powders could be soluble, as those of a nickel ethylene-tetrathiolate complex-based polymer, [(NiC2S4)[BMIM]x]n, ɛ [BMIM][BF4], which are very soluble in solvents such as acetone [17].
4 Experimental
The chemical syntheses have been carried out under an argon atmosphere using freshly distilled and degassed solvents. [(CH3)(n-C8H17)3N][Cl] is commercially available. Other starting compounds are prepared following previously described procedures: (TTF)3(BF4)2 in [18], [(n-C4H9)4N][Ni(dmit)2] in [19], and [BMIM][N(CF3SO2)2] in [20].
4.1 Preparation of TTF[Ni(dmit)2]2 nanoparticles
A solution of 100 mg of (TTF)3(BF4)2 (0.13 mmol) and 3.35 g (8 mmol) of [BMIM][N(CF3SO2)2] (or 0.70 g, i.e. 1.73 mmol, of [(CH3)(n-C8H17)3N][Cl]) in 20 mL of acetonitrile is added dropwise to a solution of 185 mg of [(n-C4H9)4N][Ni(dmit)2] in 20 mL of acetone at −80 °C without stirring. A black precipitate of TTF[Ni(dmit)2]2 is observed as soon as the suspension is added. After warming to room temperature, the black solid is filtered off, washed with 3 × 5 mL of acetonitrile and finally dried under vacuum (15 hours). The resulting black powder is air stable (yield: 95% when [BMIM][N(CF3SO2)2] is used and 40% when [(CH3)(n-C8H17)3N][Cl] is used).
4.2 Characterization of TTF[Ni(dmit)2]2 nanoparticles
Elemental analyses are performed by the Microanalysis Service of LCC–CNRS. For transmission electron microscopy observation, powder (0.5 mg) is dispersed in diethyl ether (2 mL) under slow stirring for 1 min. The TEM specimens are then prepared by evaporation of droplets of suspension deposited on carbon-supported copper grids. The experiments are performed on a JEOL Model JEM 1011 operating at 100 kV. Histograms of sizes are obtained using the ImageJ software (developer: W. Rasband). Infrared spectra (in KBr matrix) are taken at room temperature on a Perkin Elmer Spectrum GX spectrophotometer. Powder X-ray diffraction pattern are collected on a XPert Pro (θ − θ mode) Panalytical diffractometer with λ (Cu Kα1, Kα2) = 1.54059, 1.54439 Å. The extraction of peak positions for indexing is performed with the fitting program, available in the PC software package Highscore+ supplied by Panalytical. Pattern indexing is carried out by means of the DICVOL program implemented in the Highscore + package. The room temperature conductivity of the samples is measured on a compressed pellet by two-probe contacts. The temperature dependence of the conductivity for TTF[Ni(dmit)2]2 nanoparticles powders is evaluated by the four-probe technique as described in the experimental section of reference [4].