1 Introduction
Catalytic oxidation is a key technology for the conversion of petroleum feedstock to useful chemicals, such as alcohols, carbonyl compounds, and epoxides. Epoxides are important synthetic intermediates for the synthesis of oxygen-containing natural or unnatural compounds [1,2]. Effective and selective methods for the catalytic functionalization of hydrocarbons are still a major challenge in both synthetic and industrial chemistry [3]. Designing a clean catalyst with high selectivity, stability and reusability is an important development in this area. Many transition metal complexes have been used as catalysts for highly selective epoxidations. Amongst them, oxovanadium (IV) complexes are oxidation catalysts for the regioselective epoxidation of allylic alcohols [4]. During the past thirty years, many research works have been focused on the synthesis of different types of vanadium (IV) coordination compounds with Schiff base ligands derived from salicylaldehydes and 1,2-diaminoalkanes, or mixtures of vanadyl acetylacetonate and Schiff bases containing amine constituents [5–28] for epoxidation reactions, either in homogeneous [7–28] or heterogeneous environment [28–37].
Different solid materials, such as microporous, mesoporous or silica and silicate layers have been used as supports. The utilization of magnetic nanoparticles as solid supports is a new approach in recent years. Magnetic nanoparticles and nanoassemblies with uniform size distribution are currently of emerging interest because of their extensive applications in memory storage devices, catalysis, sensors, MRI, magnetically controlled drug delivery and hyperthermia treatment of tumour cells [38–46]. Immobilization of catalytically active species on the surface of magnetic particles is a suitable method because separation by applying an external magnetic field is easy [47–49].
Herein, the synthesis, characterization and epoxidation catalytic activity of a new stable and recyclable heterogeneous catalyst involving covalently attached vanadium to modified Fe3O4 through a tetra-dentate Schiff base ligand is described.
2 Experimental
2.1 Materials
Ferrous chloride tetra hydrate FeCl2·4H2O, ferric chloride hexahydrate FeCl3·6H2O, sodium chloride NaCl, sodium silicate, hydrochloric acid, aqueous NH3 (25% wt%), chloropropyl trimethoxysilane (Cl-PTMS), ethanol, dichloromethane, triethylamine, tert-butylhydroperoxide (TBHP, 80% in ditertiary butyl ether), acetonitrile, chloroform, trans-2-hexene-1-ol, limonene, 1-octene-3-ol, vanadyl sulfate, and geraniol were purchased from Merck Chemicals and were used without further purification. The Schiff base ligand, N,N-bis(3-salicylidenaminopropyl)amine (salpr) was prepared as reported before [50].
2.1.1 Preparation of (ClpSCMNPs)
Fe3O4 magnetic nanoparticles (MNPs) were prepared according to the previously reported method [51] followed by coating with sodium silicate [51]. ClpSCMNPs and salpr/SCMNPs were also prepared based on the reported procedure [52].
2.1.2 Preparation of (VOsalpr/SCMNPs)
A mixture of VOSO4 × 5H2O (0.4 g, 1.5 mmol) in ethanol (50 mL) and salpr/SCMNPs (1 g) was sonicated and then heated under reflux for 12 h. After separation of the solid by means of an external magnet, washing with ethanol in order to remove the unreacted vanadium residue and vacuum drying, VOsalpr/SCMNPs was obtained.
2.2 Catalytic epoxidation, general procedure
All epoxidation reactions of trans-2-hexene-1-ol, geraniol, limonene and 1-octene-3-ol were carried out in a bottom flask equipped with a stir bar and a condenser. Typically, a mixture of catalyst (50 mg) and substrate (20 mmol) in chloroform (10 mL) was added to the reaction flask upon slow stirring. After several minutes, TBHP (24 mmol, 80% in ditertiary butyl peroxide) was added and the mixture was heated under reflux for an appropriate time. After separation of the solid by means of an external magnetic field, the solution was subjected to GC and GC–mass analysis.
2.3 Instrumentation
X-ray diffraction patterns were obtained using a Siefert 3003 PTS diffractometer using the Cu Kα radiation (λ = 1.5406 Å). FT–IR spectra were recorded on a Bruker Tensor 27 IR spectrometer in KBr pellets over the 4000–400 cm−1 range under atmospheric conditions. The amount of vanadium in the complex was determined by atomic absorption spectroscopy (AAS) with a GBC flame spectrophotometer. Magnetic susceptibility measurements were carried out using a vibrating sample magnetometer (VSM) (BHV-55, Riken, Japan) in the magnetic field range from –8000 Oe to 8000 Oe at room temperature. Epoxidation products yields were analyzed by GC and GC–mass spectrometry using an Agilent 6890 Series with a FID detector, an HP-5% phenylmethylsiloxane capillary and an Agilent 5973 Network, mass selective detector, HP-5MS 6989 Network GC system, respectively.
2.4 Results and discussion
2.4.1 Characterization of VOsalpr/SCMNPs
The preparation method of VOsalpr/SCMNPs is presented in Scheme 1. Initially, the external surface of MNPs was coated with sodium silicate. Subsequent addition of Cl-PTMS followed by reaction with salpr and the VOSO4 afforded the desired VOsalpr/SCMNPs nanoparticles (Scheme 1).

Preparation of VOsalpr/SCMNPs.
The FTIR spectra of Fe3O4, Fe3O4@SiO2, ClpSCMNPs, salpr/(SCMNPs), VOsalpr/(SCMNPs) and that obtained by subtraction of the salpr/SCMNPs from VOsalpr/(SCMNPs) are shown in Fig. 1a–f, respectively. As shown in Fig. 1a and b, the bands appearing at 570 and 1100 cm−1 are assigned to Fe–O and Si–O vibrations due to the presence of Fe3O4 and SiO2 components. After functionalization with Cl-PTMS, two obvious bands appearing at 2860 and 2927 cm−1 are associated with C–H stretching vibrations (Fig. 1c). This is consistent with the predicted structure of Fe3O4@SiO2 @Cl-PTMS. The observation a weak band at 1639 cm−1 in Fig. 1d and a shift to the lower frequencies (1630 cm−1) after VO complexation is attributed to the CN vibration (Fig. 1e) [51–53]. The VO stretching vibration evidenced at around 970–1000 cm−1 in the complex is absent, perhaps masked by the strong and broad Si–O–Si and Si–OH vibrations positioned at 1000–1100 cm−1. The observation of a vibration band at 983 cm−1 after subtraction of the salpr/(SCMNPs) spectrum from that of VOsalpr/(SCMNPs) (Fig. 1f) confirms the formation of the VO complex on the modified iron oxide nanoparticles [19].
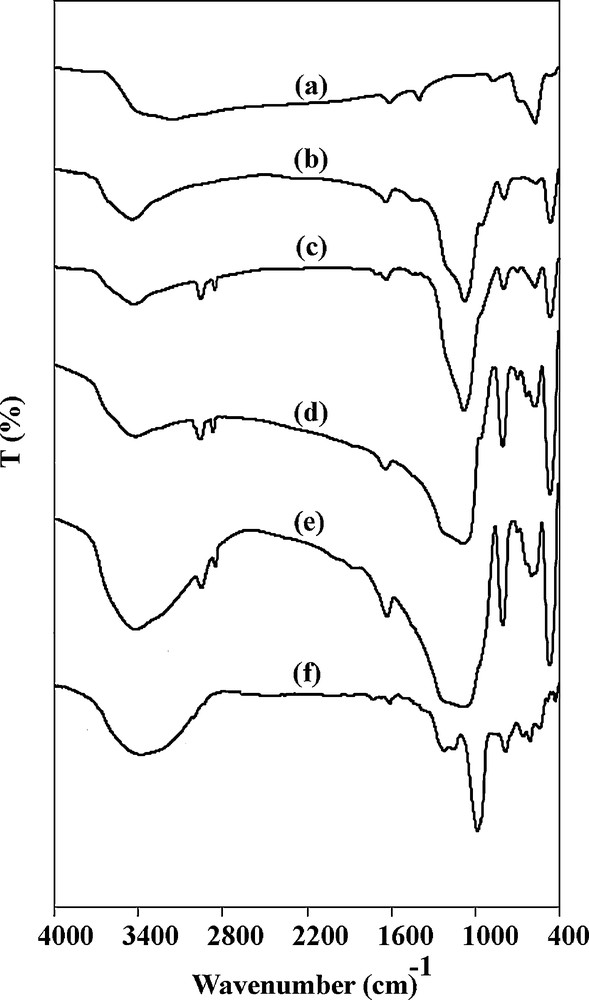
FT–IR spectra of (a) Fe3O4, (b) Fe3O4@SiO2, (c) ClpSCMNPs, (d) salpr/(SCMNPs), (e) VOsalpr/(SCMNPs), and (f) the difference spectrum of VOsalpr/(SCMNPs) and salpr/(SCMNPs).
The X-ray powder diffraction patterns of Fe3O4, ClpSCMNPs, salpr/(SCMNPs) and VOsalpr/(SCMNPs) are shown in Fig. 2a–d, respectively. The diffraction peaks of Fe3O4 nanoparticles with 2θ at 18.34°, 30.02°, 35.43°, 43.11°, 53.40°, 57.03° and 62.77° related to the (111), (220), (311), (400), (422), (511) and (440) planes (Fig. 2a) are consistent with JCPDS card No. 01-087-2334, which exhibits the characteristic peaks of iron oxides with a face-centered cubic structure. No other characteristic peak such as those of hematite impurities or hydroxides was detected [54–56]. The similar set of characteristic peaks observed for ClpSCMNPs, salpr/(SCMNPs) and VOsalpr/(SCMNPs) (Fig. 2b–d) indicates the stability of the ClpSCMNPs crystalline phase of silica coating and surface functionalization. Observation of a broad peak in all three samples indicates the nanocrystalline nature of some iron oxide nanoparticles after coating with Cl-PTMS. Moreover, the fact that no additional peaks due to other phases are detected indicates that no reaction has occurred between the core and the shell during the synthesis process.

The XRD patterns of (a) Fe3O4, (b) ClpSCMNPs, (c) salpr/(SCMNPs), (d) VOsalpr/(SCMNPs).
The transmission electron microscopy (TEM) results of the magnetic nanomaterial Fe3O4 and VOsalpr/(SCMNPs) are shown in Fig. 3a,b, respectively. As seen in Fig. 3a, Fe3O4 nanoparticles about 8 nm in diameter have been formed. A change in the particles’ diameter to 12–15 nm occurred after the immobilization of the VOsalpr complex within the modified MNPs (Fig. 3b).
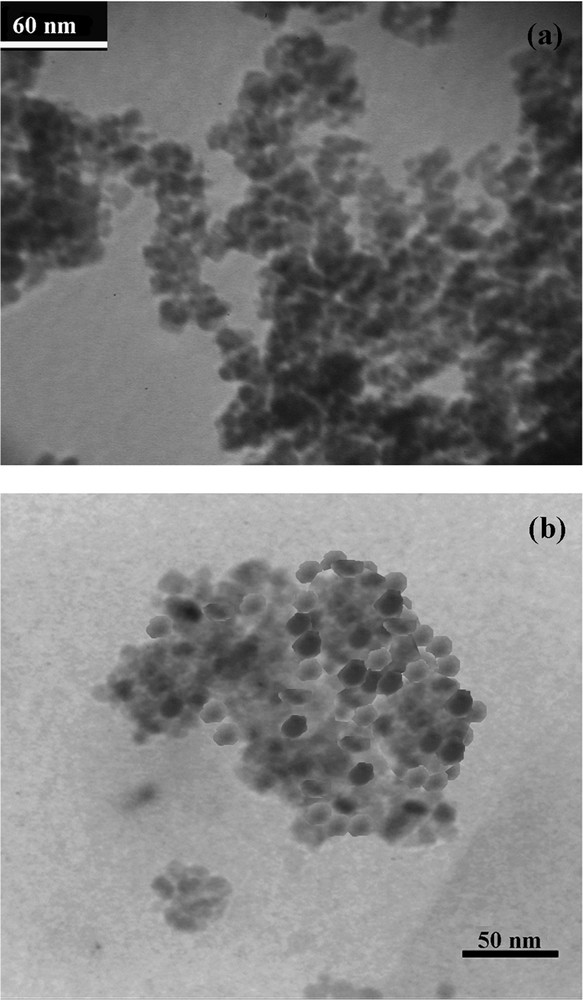
TEM images of (a) Fe3O4, (b) VOsalpr/(SCMNPs).
2.5 Epoxidation of allyl alcohols catalyzed by VOsalpr/SCMNPs
Epoxidation reactions were carried out in the presence of VOsalpr/SCMNPs (50 mg) as a catalyst over different durations using trans-2-hexene-1-ol as the representing substrate in order to obtain the best reaction time. As seen in Fig. 4, trans-2-hexene-1-ol is mostly oxidized within 4 h, beyond which no considerable oxidation occurs.

Conversion and selectivity of trans-2-hexene-1-ol for different reaction times in the presence of the VOsalpr/(SCMNPs) catalyst.
The effect of the amount of catalyst is shown in Fig. 5. As given in this figure, increasing the amount of catalyst from 5 mg to 50 mg increases the conversion from 90% to 100%. Therefore, all epoxidation reactions were carried out using 50 mg of catalyst.
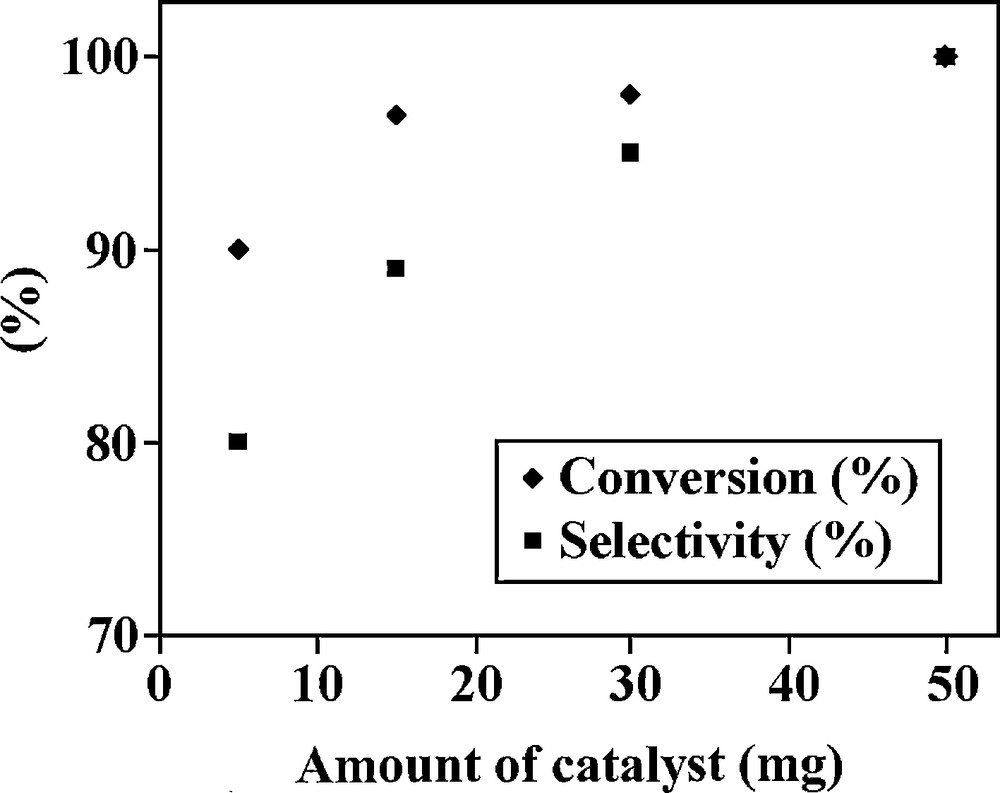
Conversion and selectivity of trans-2-hexene-1-ol in the presence of different amounts of the VOsalpr/(SCMNPs) catalyst.
Epoxidation results obtained for trans-2-hexene-1-ol, geraniol, limonene and 1-octene-3-ol with TBHP in chloroform in the presence of VOsalpr/SCMNPs are given in Table 1. Quantitative conversions of trans-2-hexene-1-ol or geraniol and 81% conversion of 1-octene-3-ol to the corresponding epoxides with 100% selectivities are notable (Table 1). On the other hand, limonene has undergone partial epoxidation through an unselective oxidation (Table 1).
Catalytic results of VOsalpr/SCMNPs in the epoxidation of the allylic alcohol with TBHP.
Substrate | Conversion (%) | Epoxide (%) | Others (%) | TONd |
Trans-2-hexene-1-ol | 100 | 100 | 0 | 746 |
Geraniol | 100 | 100 | 0 | 746 |
Limonene | 50 | 62a | 38b | 373 |
1-Octene-3-ol | 81 | 99 | 1 | 604 |
Trans-2-hexene-1-ol | 82c | 100 | 0 | 612 |
a cis-Limonene oxide (26%), trans-limonene oxide (36%).
b Limonenone (19%), limonene diol (19%).
c Catalytic test with recovered catalyst. The conversion was determined based on chlorobenzene as an internal standard.
d TON is the mmoles of product to mmoles of vanadium present in catalyst.
The stability of the catalyst in the epoxidation of trans-2-hexene-1-ol was studied by recycling three times the recovered catalyst (Fig. 6). As can be seen, no selectivity change is observed, although conversion was decreased from 100 to the 80%. The redetermination of the vanadium content using atomic absorption spectroscopy showed that the used catalyst had a vanadium content of 2.71%, compared to that of the initial catalyst (2.74%), which means a 0.03% reduction in the vanadium content. The filtrate solution revealed no epoxidation activity for the allyl alcohol, confirming the heterogeneity character of the catalyst.
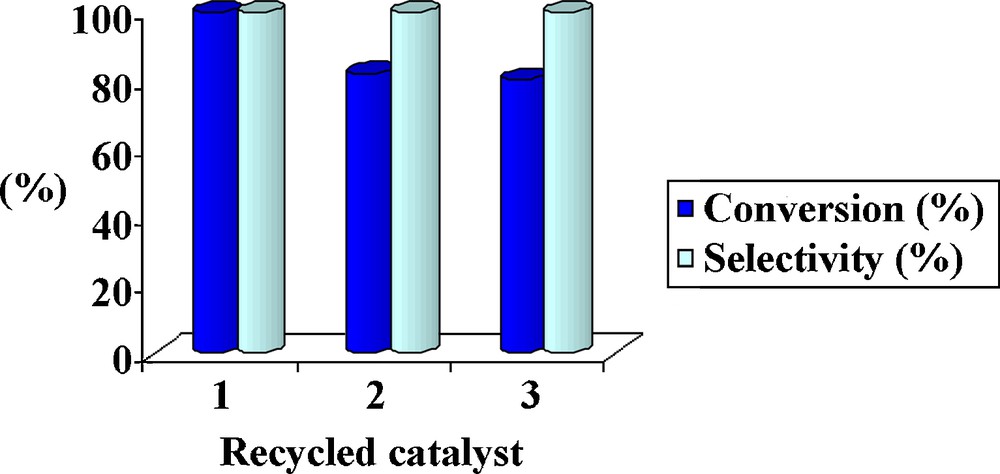
(Color online.) Recyclability of the catalyst in the epoxidation of trans-2-hexene-1-ol.
In order to study the magnetic properties of VOsalpr/SCMNPs before and after using it as a catalyst, the hysteresis loops have been investigated at room temperature by means of vibrating sample magnetometry (VSM) (Fig. 7a,b). Whereas the magnetization curve of VOsalpr/SCMNPs before using as catalyst exhibited no remanence effect (superparamagnetic property) with a saturation magnetization of about 11.37 emu/g, the magnetic nanoparticles of the used catalyst showed a superparamagnetic behaviour with a decrease of 7.18 emu/g in saturation magnetization. Recall that the superparamagnetic properties of the prepared VOsalpr/SCMNPs are critical for their application in preventing aggregation and enables metals to redisperse rapidly after the magnetic field has been removed. As mentioned before, the presence of magnetic properties after the catalyst has been recycled several times confirms the activity and the heterogeneity of the catalyst.

Magnetization curve of VOsalpr/SCMNPs (a) before and (b) after catalytic epoxidation reactions.
To get a better insight into the reaction mechanism, epoxidation reaction of trans-2-hexene-1-ol was carried out in the presence of diphenylamine as a radical scavenger [57]. The fact that no depletion in the epoxide yield was observed revealed that no radical intermediate is implicated in the oxidation process. Therefore, the formation of the epoxide seems to have occurred via the initial coordination of both allylic alcohol and hydroperoxide to the metal center followed by oxygen transfer from peroxide to alcohol via a concerted process [58].
3 Conclusion
VOsalpr/SCMNPs was prepared and characterized via the complexation of VOSO4 on modified Fe3O4. This catalyst successfully catalyzed the epoxidation of some allyl alcohols, such as limonene, 1-octene-3-ol, trans-2-hexene-1-ol and geraniol with 50 to 100% conversion and 62 to 100% selectivity. It was found the catalyst can be re-used for at least three times without significant reduction in its catalytic activity. Based on the promising advantages of magnetic nanoparticles in comparison to those of conventional catalyst supports, the utilization of VOsalpr/SCMNPs is recommended for consideration by researchers from the chemical industry.
Acknowledgements
The financial support from the Alzahra University is gratefully acknowledged.