1 Introduction
Lipid-based nanoparticles, such as solid lipid nanoparticles (SLNs) and nanostructured lipid carrier (NLCs) have been broadly used as novel delivery systems for cosmetics [1,2] and for pharmaceutical lipophilic drugs [3]. They are submicron particles with a lipid matrix that stays solid at body and room temperatures. The lipid matrix of SLNs is entirely composed of solid lipids, while that of NLCs is composed of a blend of solid and liquid lipids [4]. Lipid-based nanoparticles have the advantage of being biodegradable and safe for human use due to their non-toxic ingredients [5]. They also have the potential to protect chemically labile active compounds against degradation [6,7]. In cosmetics, these properties make them a viable replacement of traditional formulations, and thus, they have been studied as encapsulation systems for various labile compounds, including sunscreen agents [8–11] and antioxidants [12,13].
Nowadays, due to the ozone layer depletion, the use of sunscreens is compulsory in order to protect the skin against the harmful effects of UV radiation [14]. Sunscreens on the market are based on UV filters that absorb, scatter or reflect the UVB (290–320 nm) and/or UVA (320–400 nm) rays [15]. Recently, it was found that the UVA rays are even more dangerous than the UVB rays. They are responsible for skin photoaging and they contribute to and even initiate the development of skin cancer [16].
Most of the sunscreens that protect against UVA rays are based on the organic UVA filter butyl-methoxydibenzoylmethane (BMDBM). The problem associated with this UV filter is its photo-instability [17], which needs to be addressed by any sunscreen that is based on it, in order to be effective. So, in the literature, there are various studies about formulations developed in order to photostabilize BMDBM [18–21].
In a previous study of the authors (Niculae et. al.), the efficacy of lipid nanoparticles in stabilizing BMDBM has been evaluated [22]. The study showed that by encapsulating BMDBM, its UVA photoprotective properties can be enhanced as compared to a reference emulsion. This enhancement has been attributed by various studies [8,9] to a synergistic effect of the UV absorbing properties of the UV filter coupled with the UV scattering properties of the solid lipid nanoparticles. When evaluating the photostability of the two carrier systems, only NLCs showed a stabilization effect, while for SLNs a significant decrease in the UVA photoprotective properties was observed.
Jutta Kockler et al. have studied the antioxidant effect of α-tocopherol, a compound of the vitamin E group, on the production of reactive oxygen species (ROS) by BMDBM (encapsulated into multilamellar phosphatidylcholine liposomes) in a phosphate buffer solution, which was exposed to UV radiation. It was observed that α-tocopherol exhibits a photostabilizing effect on BMDBM, the ROS generated in this case being fewer than for BMDBM without α-tocopherol [23].
In cosmetic products, α-tocopherol is used as an antioxidant and skin care substance being able to reduce the skin damage caused by UV radiation, and thus, preventing the photoaging [24]. It was also shown that vitamin E derivatives present UV protective properties, mainly in the UVB region [25], and in combination with UV filters lead to a synergistic effect [26]. Because tocopherol is sensitive to heat, light and oxygen, it was encapsulated into SLNs by Wissing et al., which obtained improved UV blocking and occlusive properties when compared to unloaded SLNs and to a reference emulsion with tocopherol [27].
Based on these studies, the research described below aims to outline the influence of α-tocopherol on the photostability of BMDBM, when they are encapsulated together into lipid-based nanoparticles. The developed nanoparticles prepared by the melt emulsification method coupled with high shear homogenization were characterized in terms of particle size and zeta potential. Cosmetic formulations based on BMDBM and α-tocopherol co-encapsulated into lipid nanoparticles were developed in order to evaluate their photoprotective properties. The effectiveness of the developed formulations was assessed by evaluating the sun protection factor (SPF) and the Erythemal UVA protection factor (EUVA–PF) initially and after subjecting them to UV irradiation.
2 Experimental
2.1 Materials
dl-α-Tocopherol was purchased from Calbiochem (Germany). Butyl-methoxy-dibenzoylmethane (BMDBM) was purchased from Merck. Polyoxyethylene-sorbitan monooleate (Tween 80 – T80) and polyoxyethylene-sorbitan monolaurate (Tween 20 – T20) were supplied by Merck (Germany). Synperonic PE/F68 (poloxamer, a block copolymer of polyethylene and polypropylene glycol) and l-α-phosphatidylcholine were obtained from Sigma–Aldrich Chemie GmbH (Munich, Germany). The solid lipids used were: n-hexadecyl palmitate (CP) purchased from Acros Organics (USA) and glyceryl stearate (GS) from Cognis GmbH. Myritol 812 (medium chain triglycerides – MCT) was purchased from Merck. The cream base (which contains stearates, glycerin, fatty alcohols, emulsifier, emollients and an antioxidant–butylhydroxyanisole) was supplied by Elmiplant S.A. Company, Romania. All the other chemicals used were of analytical grade.
2.2 Preparation of lipid nanoparticles
Lipid nanoparticles were prepared using the melt emulsification method coupled with high shear homogenization according to the method described in the previous study of Niculae et al. [22]. Briefly, the lipid phase consisted in CP, GS–for SLN preparation, together with MCT–for NLC preparation was heated under stirring at 85 °C. The active compounds, 0.5% BMDBM and/or 0.5% tocopherol (w/w) were added into the lipid phase and stirred until a clear molten solution was formed. The aqueous phase consisting of 3.5% (w/w) surfactant mixture composed of Tween 20/Tween 80, poloxamer and phosphatidylcholine was also heated under stirring at 85 °C. Before gradually adding the lipid phase into the aqueous phase to form a pre-emulsion, the latter was subjected to a high shear homogenization with a Lab rotor-stator Homogenizer at 15,000 rpm for 2 min (PRO250 type; 0–28,000 rpm; power of 300 W, Germany). After 2 h of stirring at 85 °C, the pre-emulsion was subjected to high shear homogenization by applying 25,000 rpm for 10 min. The obtained emulsion was allowed to cool under stirring at room temperature with formation of lipid nanoparticles dispersions loaded with active compounds.
In order to remove the excess of water and to increase the amount of active compounds into nanoparticles formulation, the dispersions were frozen overnight at –25 °C and they were lyophilized for 72 h at –55 °C using an Alpha 1-2 LD Freeze Drying System, Germany. The composition of lipid nanoparticles in dispersion is shown in Table 1.
The composition of SLN and NLC formulations in aqueous dispersion.
Formulation | Main surfactant (w/w %) | Lipids (w/w %) | Active compounds (w/w %) | ||||
T80 | T20 | CP | GS | Myritol | α-Tocopherol | BMDBM | |
SLN 1 | 2.45 | – | 5 | 5 | – | 0.5 | – |
SLN 2 | – | 2.45 | 5 | 5 | – | 0.5 | – |
NLC 1 | 2.45 | – | 3.5 | 3.5 | 3 | 0.5 | – |
NLC 2 | – | 2.45 | 3.5 | 3.5 | 3 | 0.5 | – |
SLN 3 | 2.45 | – | 5 | 5 | – | – | 0.5 |
SLN 4 | – | 2.45 | 5 | 5 | – | – | 0.5 |
NLC 3 | 2.45 | – | 3.5 | 3.5 | 3 | – | 0.5 |
NLC 4 | – | 2.45 | 3.5 | 3.5 | 3 | – | 0.5 |
SLN 5 | 2.45 | – | 5 | 5 | – | 0.5 | 0.5 |
SLN 6 | – | 2.45 | 5 | 5 | – | 0.5 | 0.5 |
NLC 5 | 2.45 | – | 3.5 | 3.5 | 3 | 0.5 | 0.5 |
NLC 6 | – | 2.45 | 3.5 | 3.5 | 3 | 0.5 | 0.5 |
2.3 Particle size and zeta potential characterization of lipid nanoparticles
The particle size was analyzed by dynamic light scattering (DLS) with a Zetasizer Nano ZS (Malvern Instruments Ltd., UK). The mean diameter (Zave) and the polydispersity index (PdI) of the lipid nanoparticles in dispersion were determined at an angle of 90°. Prior to analysis, the samples were diluted with double-distilled water in order to produce an adequate scattering intensity. The zeta potential (ZP) was determined by measuring the electrophoretic mobility of the nanoparticles in an electric field with the appropriate accessory of the Zetasizer Nano ZS. The ZP reflects the electric charge on the particle surface and it is a measure of the physical stability of colloidal systems. Prior to measurements, the lipid nanoparticles dispersions were diluted with double distilled water and the conductivity was adjusted to 50 μS/cm with a sodium chloride solution (0.9%, w/v). The measurements were performed at 25 °C and the results were given as average of three individual measurements.
2.4 UV analysis
Cream formulations based on SLNs/NLCs were developed by incorporating the lyophilized lipid nanoparticles into a cream base. The photoprotective properties of lipid nanoparticles were preliminary assessed by evaluating their ultraviolet absorption. Thus, the creams (2 mg/cm2) were evenly applied onto a quartz plate covered with Transpore™ tape (3 M Health Care, USA) and the absorption spectrum was registered from 290 to 400 nm with a UV–Vis V670 Jasco Spectrophotometer equipped with an integrated sphere. A quartz plate covered with Transpore™ tape and without sample was used as the reference support [28]. The absorption spectrum of each sample was obtained from six measurements in different points.
2.5 In vitro protection factors’ measurements
The in vitro determination of EUVA–PF was performed according to Diffey and Robson methodology [29]. The method is based on the measurement of the radiation intensity transmitted through the substrate of the sample applied according to the UV analysis method, and by recording the photocurrent in 5-nm steps from 290 to 400 nm. The EUVA–PF was calculated following the SPF equation and considering the UVA wavelength ranging between 320–400 nm. The SPF and EUVA–PF were calculated by using the following equations [30]:
(1) |
(2) |
2.6 Photostability studies
The photostability of the developed creams was evaluated by exposing them to UV radiation at two wavelengths, 365 nm (UVA) and 312 nm (UVB), using the BioSun system (Vilver Lourmat, France). The samples were irradiated over two stages with a low energy in order to simulate the solar energy during the midday: irradiation I – with an energy of 19.5 J/cm2 and irradiation II with an energy of 39 J/cm2. The SPF of each sample was evaluated after irradiation.
3 Results and discussion
3.1 Particle size measurements
The mean particle size and the polydispersity index of the lipid nanoparticles loaded with α-tocopherol, BMDBM and α-tocopherol together with BMDBM are indicated in Fig. 1.

Mean particle size and polydispersity index of lipid nanoparticles, SLNs and NLCs, synthetized with different surfactants. (For interpretation of the references to colour in this figure legend, the reader is referred to the web version of this article).
The developed lipid nanoparticles showed a relative uniform distribution of mean particle size with PdI lower than 0.26. The nanoparticles prepared with Tween 20 as the main surfactant have particle sizes lower than those prepared with Tween 80, similar to the results obtained in our previous study [22]. NLCs synthetized with Tween 20 loaded with tocopherol exhibit larger particle sizes than those loaded with BMDBM. In general, by encapsulating BMDBM together with tocopherol into the lipid matrix, the size of nanoparticles is slightly increasing when compared to that of SLNs/NLCs loaded with only one active compound.
The mean particle size of NLC 2 loaded with tocopherol is 180 nm, while after the co-encapsulation with BMDBM, the mean particle size of the resulted NLC 6 is 181.2 nm. Thus, the lipid matrix of NLCs synthetized with Tween 20 presents many imperfections in the crystalline lattice of lipid core, having the ability to encapsulate both BMDBM and tocopherol without disturbing to a significant extent the matrix of the nanoparticles.
3.2 Physical stability
Both types of lipid nanoparticles, encapsulated and co-encapsulated, present a very good physical stability with zeta potentials more electronegative than –35 mV (Fig. 2). Generally, the NLCs are more stable than SLNs formulations (Fig. 2). Among NLCs, co-encapsulated NLC 6 prepared with Tween 20 has the best physical stability (ZP = –47 mV). Regarding the influence of encapsulation of one or two active compounds on physical stability, it can be observed that by co-encapsulation of both types of actives, the resulted SLNs and NLCs manifest a better physical stability as compared to lipid nanoparticles loaded with only one active compound (e.g., from –40 mV for α-tocopherol and –36.6 mV for BMDBM to –46 mV for α-tocopherol and BMDBM loaded into SLN T20).

Physical stability of lipid nanoparticles encapsulated with different active compounds evaluated in terms of zeta potential. (For interpretation of the references to colour in this figure legend, the reader is referred to the web version of this article.)
3.3 In vitro protection factors’ measurements
For the in vitro evaluation of the photoprotective properties of the lipid nanoparticles, cosmetic formulations were developed by incorporating lyophilized NLCs into a cream base. The developed formulations have a minimum content of UVA filter, 1.25% BMDBM, and 1.25% tocopherol. Figs. 3 and 4 illustrate the EUVA–PFs and SPFs of the formulations.
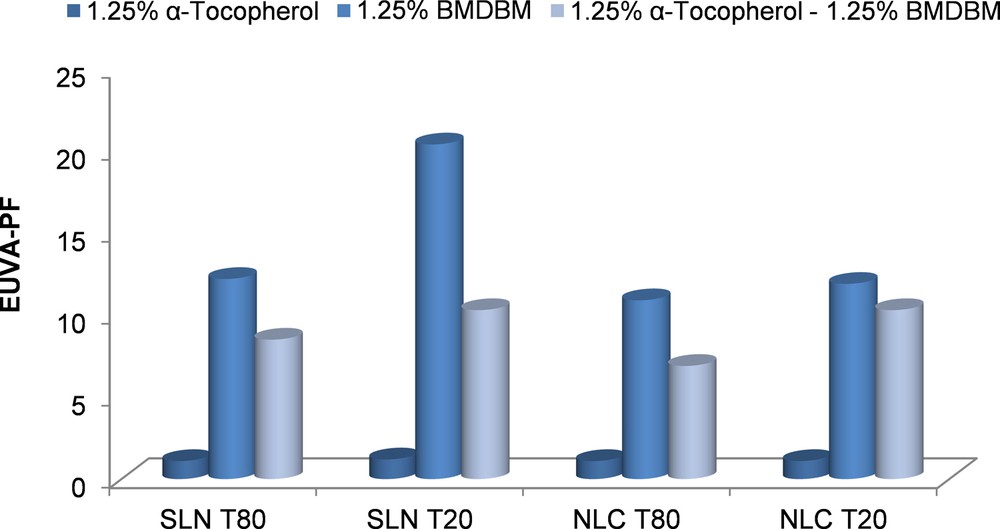
EUVA–PFs of cosmetic formulations based on lipid nanoparticles encapsulated with different active compounds. (For interpretation of the references to colour in this figure legend, the reader is referred to the web version of this article.)
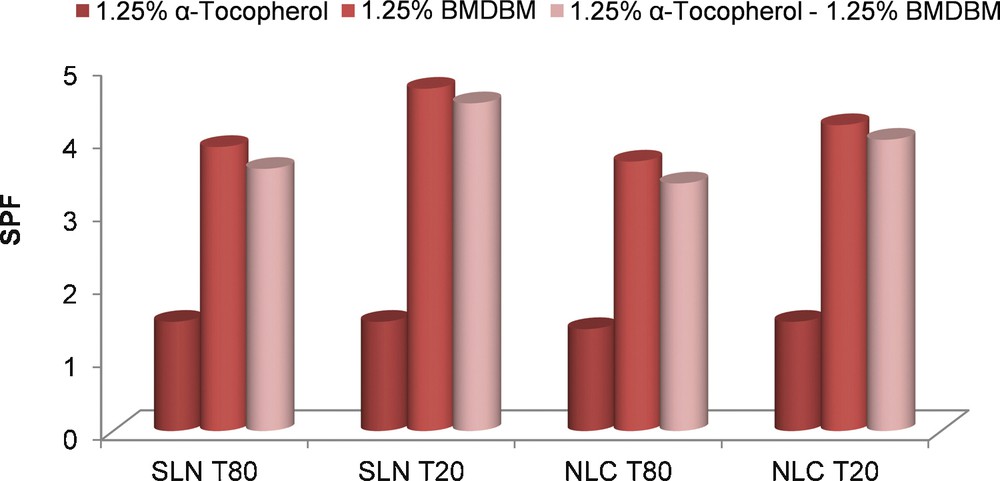
SPF of cosmetic formulations based on lipid nanoparticles encapsulated with different active compounds. (For interpretation of the references to colour in this figure legend, the reader is referred to the web version of this article.)
Comparing the absorption spectra of the NLCs with Tween 20 as the main surfactant (Fig. 5), it can be observed that NLC 2 loaded with tocopherol presents an increase in the ultraviolet absorption in the UVB range in comparison to unloaded NLCs, even though, this increase does not lead to significant photoprotective properties for tocopherol-lipid nanoparticles that have a EUVA–PF and a SPF of 1.1 and 1.5, respectively.

Absorption spectra of cosmetic formulations based on unloaded and loaded NLC with Tween 20 as the main surfactant. (For interpretation of the references to colour in this figure legend, the reader is referred to the web version of this article.)
By co-encapsulation of tocopherol with BMDBM into lipid nanoparticles, a reduction in the ultraviolet absorption in the UVA range coupled with an increase in the UVB range was observed when compared to BMDBM encapsulated alone. Regarding the photo protection factors, a decrease of the EUVA–PF and SPF values was obtained when encapsulating tocopherol together with BMDBM. The biggest difference was obtained for SLN 6 with Tween 20 (about 10 units), and the smallest difference for NLC 6 with Tween 20 (1.6 units). In case of SLNs, this can be explained by the fact that the lipid matrix does not allow enough available space for the entrapment of two active compounds. In other words, either a part of the space occupied by BMDBM into the lipid matrix is taken by tocopherol when the two substances are encapsulated together, obtaining less BMDBM content entrapped, or the tocopherol is at the surface of nanoparticles, in the surfactant shell, thus blocking the effect of the UV filter found in the lipid core. For NLCs with Tween 20, the EUVA–PF values remain closer after co-encapsulation. A possible explanation can be the formation of a disordered crystalline structure by the oil consisting of medium chain triglycerides, and by the surfactant Tween 20, having as a result a number of free spaces capable for BMDBM and tocopherol entrapment.
As for the SPF values, the differences between BMDBM encapsulated into lipid nanoparticles with or without α-tocopherol are very small, by 0.2 units lower for the co-encapsulated systems.
3.4 Photostability of lipid nanoparticles
In order to evaluate the effectiveness of using tocopherol for the stabilization of BMDBM encapsulated into lipid nanoparticles, especially in the SLN systems, the developed cosmetic formulations based on SLNs and NLCs underwent a controlled irradiation into two stages.
The EUVA–PFs of the cosmetic formulations based lipid nanostructures with tocopherol and BMDBM (Fig. 6) are increasing after irradiation for both systems, NLCs and SLNs (from the initial value of 10.3 to 12 for SLN 4), while for the creams with BMDBM, only those based on NLCs maintain their photoprotective action. In Fig. 7, an example of absorption spectra of the cream based on tocopherol–BMDBM–SLN 5 subjected to irradiation is shown.

Irradiation effect on EUVA–PFs of cosmetic formulations encapsulated with different active compounds. (For interpretation of the references to colour in this figure legend, the reader is referred to the web version of this article.)

Absorption spectra of cosmetic formulation based on 1.25% α-tocopherol–SLN 2 exposed to UV radiation: (1) initially, (2) after stage I, (3) after stage II of irradiation. (For interpretation of the references to colour in this figure legend, the reader is referred to the web version of this article.)
In addition, the SPF values (Fig. 8) of creams containing α-tocopherol and BMDBM are increasing much after irradiation when compared to creams containing only BMDBM.
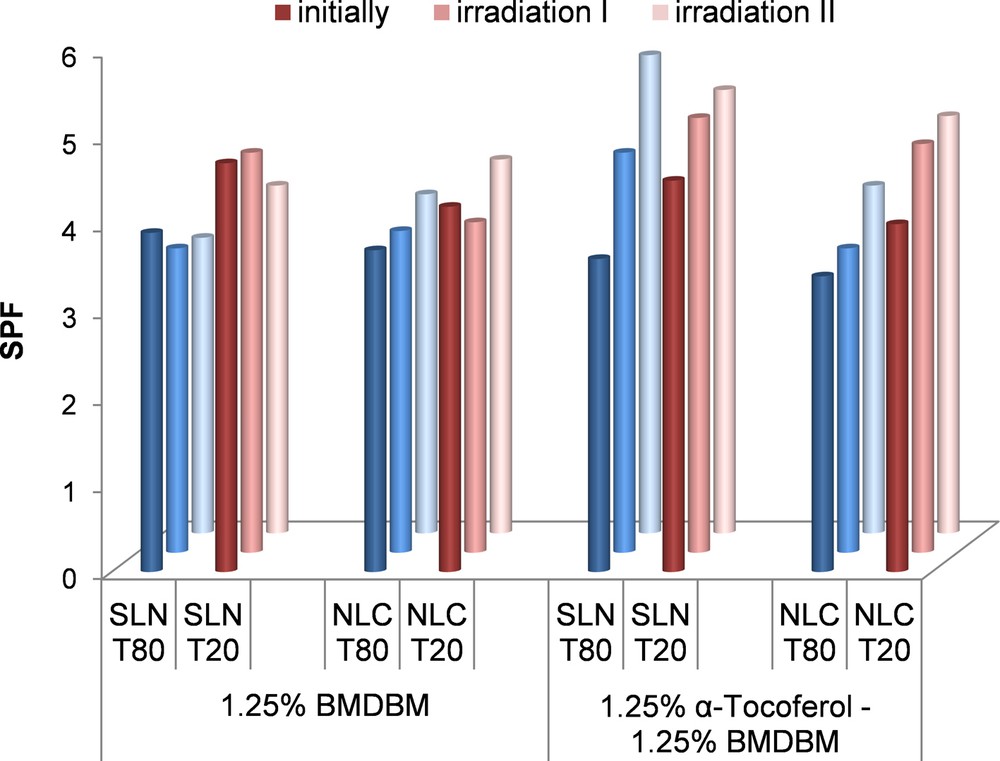
Irradiation effect on EUVA–PFs of cosmetic formulations encapsulated with different active compounds. (For interpretation of the references to colour in this figure legend, the reader is referred to the web version of this article.)
The improved photoprotective properties of the developed cosmetic formulations could be attributed to the antioxidant activity of tocopherol that provides stability of BMDBM by scavenging the reactive oxygen species resulting from UV exposure.
4 Conclusions
Lipid-based nanoparticles, SLNs and NLCs, co-encapsulated with two different active compounds, an UVA filter from the dibenzoylmethane class and an antioxidant, α-tocopherol, were successfully developed by using the melt emulsification method coupled with high shear homogenization. Physical stable nanoparticles with dimensions below 200 nm were obtained. The co-encapsulation of UVA filter and α-tocopherol into lipid nanoparticles determines only a slight increase of the size when compared to that of SLNs/NLCs loaded with only one active compound, fact which proves the effective capacity of the lipid matrix to incorporate both kinds of active compounds.
The presence of α-tocopherol into the lipid matrix has led to a slight decrease of the Erythemal UVA protection factors and SPFs of the co-encapsulated lipid nanoparticles when compared to those with UVA filter encapsulated alone. However, α-tocopherol proved to be effective in the photostabilization of the unstable UVA filter encapsulated into both systems, SLNs and NLCs, exposed to UV radiation. The comparative study achieved on the two types of lipid nanoparticles has revealed better photoprotective properties for SLNs systems than for NLCs systems.
This research contributes to the development of effective nano-lipid-based photoprotective formulations with a therapeutic potential due to the presence of antioxidants.
Acknowledgements
The work has been funded by the Partnership in priority domains program-PN-II, with support from ANCS, CNDI–UEFISCDI, project No PN-II-PT-PCCA-132/2012.