1 Introduction
In recent years, the concept of “privileged medicinal structures or scaffolds” has emerged as one of the guiding principles of the drug discovery process [1]. This term was originally introduced by Merck researchers in their work on benzodiazepins [2]. These privileged scaffolds commonly consist of a rigid hetero ring system that assigns a well-defined orientation of appended functionalities for target recognition.
The chromene moiety often appears as an important structural element in both biologically active and natural compounds [3–6]. It is widely performed in natural flavonoids (flavons, flavonols, catechins, tocopherols, anthocyanins, anthocyanidins) [7–10] and was also found in some natural alkaloids [11,12]. Among different types of chromene systems, 2-amino-4H-chromenes are of particular utility since they belong to privileged medicinal scaffolds, and are used for the treatment of viral hepatitis [13], Alzheimer's disease [14], cardiovascular disorders, epilepsy, inflammatory bowel syndrome [15], hypertension, and atherosclerosis [16].
Increasing interest in 2-amino-4H-chromene derivatives bearing a nitrile group is connected with their application to the treatment of human inflammatory diseases such as carcinoma [17], arthritis [18], leukemia [19], and to cancer therapy [20,21].
The discovery of novel synthetic methodologies to facilitate the preparation of compound libraries is a pivotal focal point of research activity in the field of modern medicinal and combinatorial chemistry. Multicomponent reaction (MCR) strategy has sufficient advantages over conventional linear-type synthesis due to its flexible, convergent, and atom-efficient nature [22].
In the last two decades, solventless organic reactions have attracted great interest. They have many advantages, such as high efficiency and selectivity, operational simplicity, low costs, mild reaction conditions, and reduced pollution [23]. The grinding method for the solid-state reactions has been reported for the Reformatsky reaction [24], aldol condensation [25], the Dieckmann condensation [26], the Knoevenagel condensation [27,28], and other reactions [29–31].
Recently, we have accomplished solvent-free and ‘on-water’ multicomponent transformations of salicylaldehydes, malononitrile and cyanoacetates [32], nitroalkanes [33] or pyrazolin-5-ones [34] into the different types of substituted 2-amino-4H-chromenes.
2 Results and discussion
In the present study, we wish to report on our results on the novel efficient multicomponent assembling of salicylaldehydes 1a–g, malononitrile, and 4-hydroxy-6-methyl-2H-pyran-2-one into the hitherto unknown 2-amino-4-(4-hydroxy-6-methyl-2-oxo-2H-pyran-3-yl)-4H-chromene-3-carbonitriles 2a–g (Scheme 1).
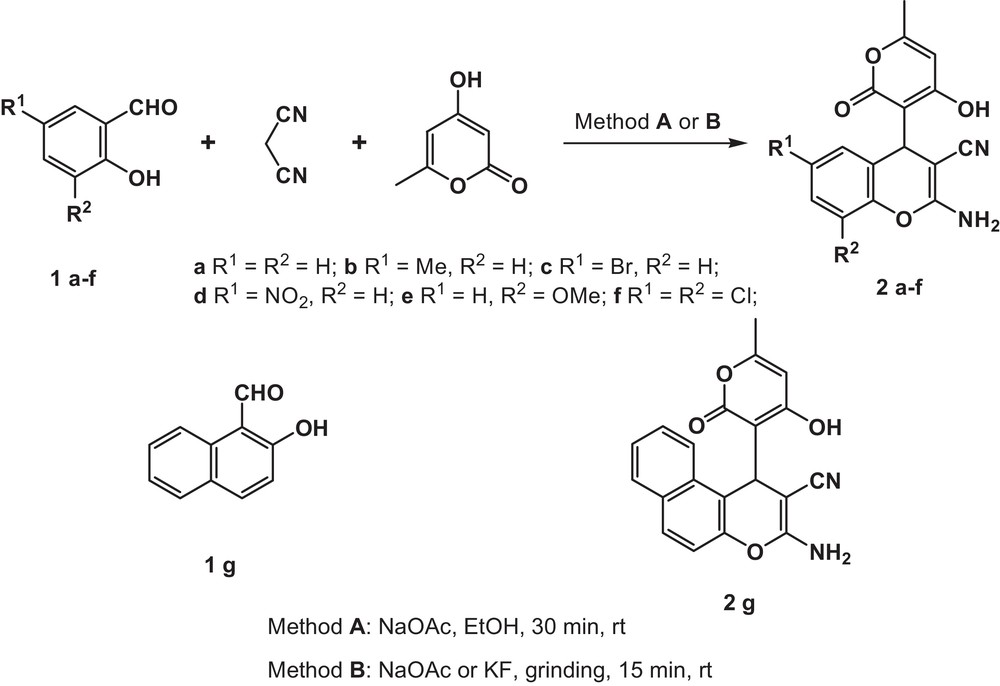
Multicomponent transformation of salicylaldehydes 1a–g, malononitrile and 4-hydroxy-6-methyl-2H-pyran-2-one into 2-amino-4-(4-hydroxy-6-methyl-2-oxo-2H-pyran-3-yl)-4H-chromene-3-carbonitriles 2a–g.
The previously unknown 2-amino-4-(4-hydroxy-6-methyl-2-oxo-2H-pyran-3-yl)-4H-chromene-3-carbonitriles 2a–g were obtained in 85–96% when we used mild (room-temperature) ‘in-solvent’ conditions [37] (Method A, Table 1).
Multicomponent transformation of salicylaldehydes 1a–g, malononitrile and 4-hydroxy-6-methyl-2H-pyran-2-one in ethanol into 2-amino-4H-chromenes 2a–ga.
Entry | Salicylaldehyde | Time, min | 2-amino-4H-chromene | Yield (%)b |
1 | 1a | 60 | 2a | 96 |
2 | 1a | 30 | 2a | 96 |
3 | 1a | 15 | 2a | 72 |
4 | 1b | 30 | 2b | 87 |
5 | 1c | 30 | 2c | 86 |
6 | 1d | 30 | 2d | 94 |
7 | 1e | 30 | 2e | 88 |
8 | 1f | 30 | 2f | 92 |
9 | 1g | 30 | 2g | 99 |
a Method A: 5 mmol of salicylaldehyde 1, 5 mmol of malononitrile, 5 mmol of 4-hydroxy-6-methyl-2H-pyran-2-one, 0.5 mmol of NaOAc, 10 mL of ethanol, ambient temperature.
b Isolated yield.
It was found that generally the reaction proceeds within 30 min (entry 2, Table 1). The reaction of salicylaldehyde 1a, malononitrile and 4-hydroxy-6-methyl-2H-pyran-2-one in the presence of 10% mol of NaOAc as a catalyst in ethanol during 15 min resulted in the formation of 2-amino-4-(4-hydroxy-6-methyl-2-oxo-2H-pyran-3-yl)-4H-chromene-3-carbonitrile 2a in only 72% yield (entry 3, Table 1).
The X-ray diffraction data unambiguously supports the configuration of 2-amino-4H-chromene 2d (Fig. 1).
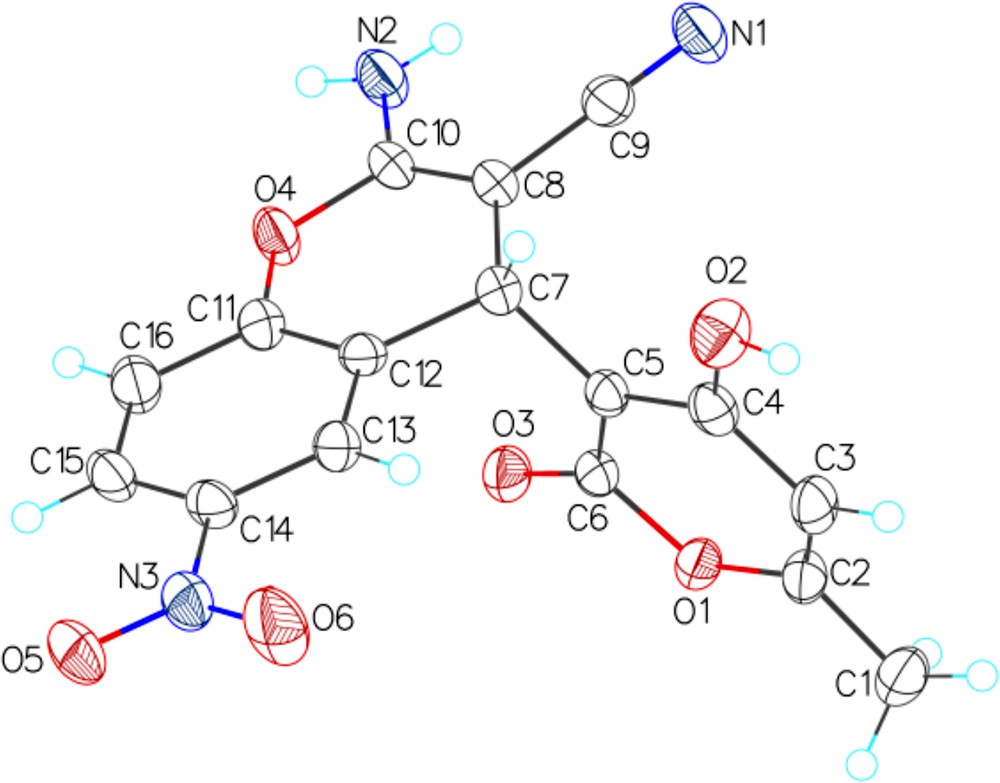
(Color online.) General view of 2d in the crystal. Atoms are represented by thermal displacement ellipsoids (p = 50%).
As it follows from the introduction, we were prompted to design a convenient, facile and ecologically reasonable methodology for the efficient synthesis of substituted 2-amino-4H-chromenes based on the multicomponent reaction of salicylaldehydes, malononitrile, and 4-hydroxy-6-methyl-2H-pyran-2-one (Scheme 1, Method B).
Thus, to evaluate the synthetic potential of the procedure proposed and to find the appropriate solvent-free conditions, the multicomponent transformation of salicylaldehyde 1a, malononitrile and 4-hydroxy-6-methyl-2H-pyran-2-one into 2-amino-4-(4-hydroxy-6-methyl-2-oxo-2H-pyran-3-yl)-4H-chromene-3-carbonitrile 2a under solvent-free conditions was also carefully studied (Table 2).
Solvent-free and ‘on-solvent’ multicomponent transformation of salicylaldehyde 1a, malononitrile and 4-hydroxy-6-methyl-2H-pyran-2-onea.
Entry | Base, mol % | Additive, mL | Time, min | Yield of 2a (%) |
1 | – | – | 15 | 26b |
2 | AcONa, 10 | – | 15 | 60b |
3 | AcONa, 10 | H2O, 2 | 15 | 73b |
4 | AcONa, 10 | EtOH, 2 | 15 | 85c |
5 | AcONa, 10 | EtOH, 2 | 20 | 85c |
6 | AcONa, 20 | EtOH, 2 | 15 | 86c |
7 | KF, 10 | – | 15 | 71b |
8 | KF, 10 | H2O, 2 | 15 | 79b |
9 | KF, 10 | EtOH, 2 | 15 | 90c |
10 | KF, 20 | EtOH, 2 | 15 | 89c |
11 | KF, 10 | MeOH, 2 | 15 | 80c |
12 | KF, 10 | PrOH, 2 | 15 | 86c |
a 2 mmol of salicylaldehyde 1a, 2 mmol of 4-hydroxy-6-methyl-2H-pyran-2-one, 2 mmol of malononitrile grinding for 15 min.
b NMR data.
c Isolated yield.
The solvent-free reaction of salicylaldehyde 1a, malononitrile and 4-hydroxy-6-methyl-2H-pyran-2-one without catalyst in a reaction time of 15 min (entry 1, Table 2) resulted in the formation of 4-pyrano-substituted 2-amino-4H-chromene 2a in only 26% yield. In the presence of 10 mol % of NaOAc as a catalyst, 2-amino-4H-chromene 2a was obtained in 60% yield (entry 2, Table 2). Using 10 mol % of NaOAc and 2 mL of water as additives led to 2a in good (73%) yield (entry 3, Table 2). And at last an 85% yield of 2a was obtained with 10 mol % of NaOAc and 2 mL of ethanol in a reaction time of 15 min. KF was a more effective catalyst in this multicomponent reaction compared to NaOAc (entries 7–10, Table 2).
Under the optimum ‘on-solvent’ conditions thus found (10 mol % of KF as catalyst, 2 mL of ethanol, reaction time 15 min), the substituted 2-amino-4H-chromenes 2a–g were obtained in excellent 80–90% yields in a reaction time of only 15 min (Table 3). As practically pure 4-pyrano-substituted 2-amino-4H-chromenes 2a–g were formed in the end of the reaction, the reaction mixture was only washed with water (2 × 2 mL) and dried to isolate pure 4-pyrano substituted 2-amino-4H-chromenes 2. Thus, the ‘on-solvent’ procedure for the synthesis of substituted 2-amino-4-(1H-pyrazol-4-yl)-4H-chromenes 2a–g in one step found by us is close to the “ideal synthesis” [38,39].
“On-solvent” multicomponent transformation of salicylaldehydes 1a–g, malononitrile and 4-hydroxy-6-methyl-2H-pyran-2-one into 2-amino-4H-chromenes 2a–ga.
Entry | Salicylaldehyde | 2-amino-4H-chromene | Yield (%)b |
1 | 1a | 2a | 90 |
2 | 1b | 2b | 82 |
3 | 1c | 2c | 83 |
4 | 1d | 2d | 86 |
5 | 1e | 2e | 80 |
6 | 1f | 2f | 88 |
7 | 1g | 2g | 90 |
a Method B: 2 mmol of salicylaldehyde 1, 2 mmol of malononitrile, 2 mmol of 4-hydroxy-6-methyl-2H-pyran-2-one, 0.2 mmol of KF, 2 mL of ethanol, grinding for 15 min.
b Isolated yield.
With the above results taken into consideration and the mechanistic data on the solvent-free cascade formation of 2-amino-4H-chromene scaffold from salicylaldehydes and malononitrile [40] as well as from salicylaldehydes and cyanoacetates [41], the following mechanism for the potassium fluoride-catalyzed multicomponent transformation of salicylaldehydes 1, malononitrile, and 4-hydroxy-6-methyl-2H-pyran-2-one into 4-pyrano-substituted 2-amino-4H-chromenes 2 is proposed. The initiation step of the catalytic cycle begins with the deprotonation of malononitrile by the action of potassium fluoride, which leads to the formation of malononitrile anion A (Scheme 2).
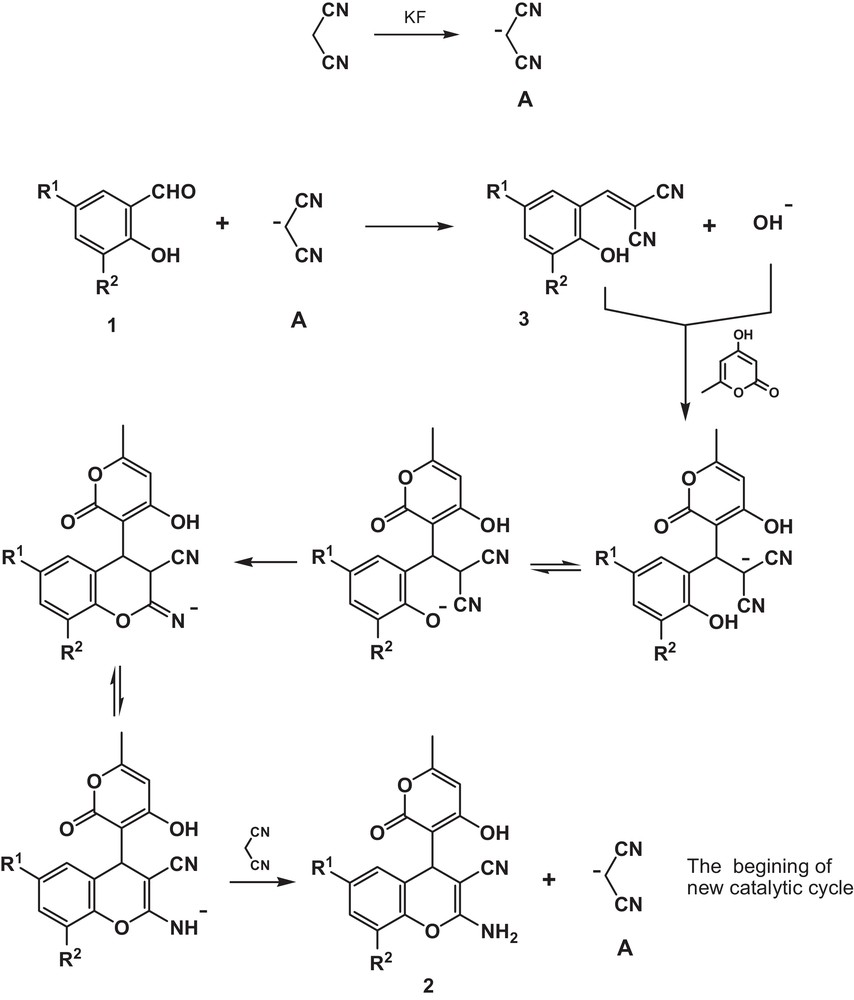
Mechanism of multicomponent transformation of salicylaldehydes 1a–g, malononitrile and 4-hydroxy-6-methyl-2H-pyran-2-one into 2-amino-4-(4-hydroxy-6-methyl-2-oxo-2H-pyran-3-yl)-4H-chromene-3-carbonitriles 2a–g.
Then the Knoevenagel condensation of the anion A with salicylaldehyde 1 takes place with the elimination of a hydroxide anion and the formation of Knoevenagel adduct 3. The subsequent hydroxide-promoted Michael addition of 4-hydroxy-6-methyl-2H-pyran-2-one to the electron-deficient Knoevenagel adduct 3 leads to the corresponding 4-pyrano-substituted 2-amino-4H-chromene 2 with the regeneration of malononitrile anion A as the last step (Scheme 2).
3 Conclusion
Thus, sodium acetate or potassium fluoride as a catalyst can produce, in ethanol as well as under ‘on-solvent’ (alcohol emulsion) conditions, a fast and selective multicomponent transformation of salicylaldehydes, malononitriles and 4-hydroxy-6-methyl-2H-pyran-2-one into 4-pyrano-substituted 2-amino-4H-chromenes in excellent yields. The new process opens an efficient and convenient one-pot way to create a novel type of substituted 2-amino-4H-chromenes—the promising compounds for the treatment of human inflammatory TNFα-mediated diseases and different biomedical applications. The catalytic procedure utilizes simple equipment; it is easily carried out and is valuable from the viewpoint of environmentally benign and diversity-oriented large-scale processes. The results obtained represent a new ‘on-solvent’ synthetic concept for multicomponent reactions, and allow for the combination of the synthetic virtues of MCR processes with considerable ecological benefits.
4 Experimental
4.1 General remarks
All melting points were measured with a Gallenkamp melting point apparatus and are uncorrected. 1H and 13C-NMR spectra were recorded with a Bruker AM-300 at ambient temperature in DMSO-d6 solutions. Chemical shift values are given in δ scale relative to Me4Si. IR spectra were registered with a Bruker ALPHA-T FT-IR spectrometer in KBr pellets. High-resolution mass spectrometry (HRMS) (electrospray ionization, ESI) measurements were made using a Bruker micrOTOF II instrument; the external or internal calibration was done with an Electrospray Calibrant Solution (Fluka). All starting materials were obtained from commercial sources and used without further purification.
4.2 Typical procedure in ethanol
A solution of salicylaldehyde 1 (5 mmol), malononitrile (5 mmol, 0.33 g), 4-hydroxy-6-methyl-2H-pyran-2-one (5 mmol, 0.63 g), and sodium acetate (0.5 mmol, 0.04 g) in ethanol (10 mL) was stirred in a 25-mL beaker at room temperature for 30 min. The reaction mixture was cooled to −10 °C for 1 h, solid phase was filtered out and dried to isolate pure 2-amino-4H-chromene 2.
4.3 Typical grinding procedure
A mixture of salicylaldehyde 1 (2 mmol), malononitrile (0.132 g, 2 mmol), 4-hydroxy-6-methyl-2H-pyran-2-one (0.252 g, 2 mmol), catalyst (10 mol %), and ethanol (2 mL) was taken in a mortar and mixed thoroughly with a pestle, then ground for 15 min. The resulting mixture was air dried in order to make the product crystallize. The crude solid was then filtered, rinsed with water (2 × 2 mL) and air dried to isolate pure 2-amino-4H-chromene 2.
4.3.1 2-amino-4-(4-hydroxy-6-methyl-2-oxo-2H-pyran-3-yl)-4H-chromene-3-carbonitrile (2a)
Yellowish solid; 1.42 g (96%); mp: 154–155 °C; νmax (KBr) = 3439, 3340, 3204, 2187, 1658, 1580, 1448, 1407, 1295, 1267 cm−1; HRMS (ESI): 319.0702 [M + Na]+, calcd for C16H12N2NaO4: 319.0689; δH (300 MHz, DMSO-d6) 2.12 (s, 3H, CH3), 4.97 (s, 1H, CH), 5.93 (s, 1H, CH), 6.61 (s, 2H, NH2), 6.86-7.06 (m, 3H, Ar), 7.12–7.20 (m, 1H, Ar), 11.65 (br s, 1H, OH); δc (75 MHz, DMSO-d6) 19.4, 29.4, 53.0, 100.6, 103.9, 115.2, 121.0, 122.7, 124.0, 127.5, 128.2, 149.5, 160.8, 161.1, 163.5, 167.0.
4.3.2 2-amino-4-(4-hydroxy-6-methyl-2-oxo-2H-pyran-3-yl)-6-methyl-4H-chromene-3-carbonitrile (2b)
Yellowish solid; 1.35 g (87%); mp: 179–180 °C; νmax (KBr) = 3464, 3355, 3131, 2189, 1656, 1583, 1498, 1404, 1289, 1269 cm−1; HRMS (ESI): 333.0853 [M + Na]+, calcd for C17H14N2NaO4: 333.0864; δH (300 MHz, DMSO-d6) 2.14 (s, 3H, CH3), 2.18 (s, 3H, CH3), 4.93 (s, 1H, CH), 5.99 (s, 1H, CH), 6.60 (s, 2H, NH2), 6.75 (d, J = 2.0 Hz, 1H, Ar), 6.80 (d, J = 8.2 Hz, 1H, Ar), 6.96 (dd, J1 = 8.2 Hz, J2 = 2.0 Hz, 1H, Ar), 11.55 (br s, 1H, OH); δc (75 MHz, DMSO-d6) 19.4, 20.2, 29.5, 52.6, 100.0, 104.3, 115.1, 121.0, 121.9, 127.8, 128.2, 133.0, 147.5, 161.3 (2C), 163.2, 165.5.
4.3.3 2-amino-6-bromo-4-(4-hydroxy-6-methyl-2-oxo-2H-pyran-3-yl)-4H-chromene-3-carbonitrile (2c)
Yellow solid; 1.61 g (86%); mp: 174–175 °C; νmax (KBr) = 3472, 3315, 3193, 2197, 1658, 1583, 1478, 1403, 1285, 1257 cm−1; HRMS (ESI): 396.9809 [M + Na]+, calcd for C16H11BrN2NaO4: 396.9794; δH (300 MHz, DMSO-d6) 2.14 (s, 3H, CH3), 4.98 (s, 1H, CH), 6.01 (s, 1H, CH), 6.75 (s, 2H, NH2), 6.90 (d, J = 8.6 Hz, 1H, Ar), 7.05 (d, J = 2.0 Hz, 1H, Ar), 7.34 (dd, J1 = 8.6 Hz, J2 = 2.0 Hz, 1H, Ar), 11.80 (br s, 1H, OH); δc (75 MHz, DMSO-d6) 19.4, 29.4, 52.4, 100.0, 103.8, 115.4, 117.8, 120.5, 125.0, 130.0, 130.5, 148.9, 160.9, 161.8, 163.1, 165.9.
4.3.4 2-amino-4-(4-hydroxy-6-methyl-2-oxo-2H-pyran-3-yl)-6-nitro-4H-chromene-3-carbonitrile (2d)
Yellow solid; 1.60 g (94%); mp: 181-182 °C; νmax (KBr) = 3458, 3330, 3193, 2195, 1689, 1579, 1527, 1407, 1340, 1257 cm−1; HRMS (ESI): 364.0538 [M + Na]+, calcd for C16H11N3NaO6: 364.0540; δH (300 MHz, DMSO-d6) 2.12 (s, 3H, CH3), 5.08 (s, 1H, CH), 6.04 (s, 1H, CH), 6.94 (s, 2H, NH2), 7.18 (d, J = 8.9 Hz, 1H, Ar), 7.76 (d, J = 2.1 Hz, 1H, Ar), 8.06 (dd, J1 = 8.9 Hz, J2 = 2.1 Hz, 1H, Ar), 12.00 (br s, 1H, OH); δc (75 MHz, DMSO-d6) 19.5, 29.5, 52.6, 100.0, 103.7, 116.7, 120.1, 123.5, 123.6, 123.9, 143.4, 154.1, 160.4, 162.1, 163.2, 166.2.
Single crystals of 2d were slowly grown from ethanol. A suitable crystal was selected, placed on a glass needle and measured on a ‘Bruker APEX-II’ diffractometer. The crystal was kept at 120 K during data collection. Using Olex2 [35], the structure was solved with the XS [36] structure solution program using Direct Methods and refined with the XL [36] refinement package using Least Squares minimizing.
Crystal Data for 2d. C20H23N3O8 (M = 433.41) (2d·2EtOH): monoclinic, space group P21/n (No. 14), a = 10.5986(7) Å, b = 9.1489(6) Å, c = 21.3199(14) Å, β = 95.8340(10)°, V = 2056.6(2) Å3, Z = 4, T = 120 K, μ(Mo Kα) = 0.110 mm−1, Dcalc = 1.400 g·mm−3, 22,948 reflections measured (3.84 ≤ 2θ ≤ 56.56), 5100 unique (Rint = 0.0750) which were used in all calculations. The final R1 was 0.0681 (> 2 σ(I)) and wR2 was 0.2134 (all data).
The crystallographic data reported for 2d have been deposited with the Cambridge Crystallographic Data Centre as supplementary publication No. CCDC 1011885. Copies of the data can be obtained free of charge via www.ccdc.cam.ac.uk/conts/retrieving.html. reference number CCDC 1011885.
4.3.5 2-amino-4-(4-hydroxy-6-methyl-2-oxo-2H-pyran-3-yl)-8-methoxy-4H-chromene-3-carbonitrile (2e)
Yellowish solid; 1.43 g (88%); mp: 195–196 °C; νmax (KBr) = 3478, 3325, 3200, 2195, 1657, 1580, 1484, 1446, 1402, 1270 cm−1; HRMS (ESI): 349.0792 [M + Na]+, calcd for C17H14N2NaO5: 349.0795; δH (300 MHz, DMSO-d6) 2.12 (s, 3H, CH3), 3.79 (s, 3H, OCH3), 4.96 (s, 1H, CH), 6.00 (s, 1H, CH), 6.51 (dd, J1 = 7.2 Hz, J2 = 1.9 Hz, 1H, Ar), 6.68 (s, 2H, NH2), 6.82–6.98 (m, 2H, Ar), 11.60 (br s, 1H, OH); δc (75 MHz, DMSO-d6) 19.3, 29.6, 52.5, 55.5, 99.9, 104.2, 110.4, 119.0, 120.9, 122.9, 123.7, 138.9, 146.6, 161.1, 161.3, 163.0, 165.5.
4.3.6 2-amino-6,8-dichloro-4-(4-hydroxy-6-methyl-2-oxo-2H-pyran-3-yl)-4H-chromene-3-carbonitrile (2f)
Yellowish solid; 1.68 g (92%); mp: 211–213 °C; νmax (KBr) = 3413, 3310, 3192, 2180, 1693, 1571, 1465, 1408, 1291, 1250 cm−1; HRMS (ESI): 386.9915 [M + Na]+, calcd for C16H10Cl2N2NaO4: 386.9910; δH (300 MHz, DMSO-d6) 2.15 (s, 3H, CH3), 5.00 (s, 1H, CH), 6.03 (s, 1H, CH), 6.91 (d, J = 2.0 Hz, 1H, Ar), 6.94 (s, 2H, NH2), 7.50 (d, J = 2.0 Hz, 1H, Ar), 11.80 (br s, 1H, OH); δc (75 MHz, DMSO-d6) 19.4, 29.8, 52.6, 99.8, 103.5, 120.0, 120.7, 126.0 (2C), 127.4, 127.7, 144.5, 160.3, 162.0, 163.0, 165.8.
4.3.7 3-amino-1-(4-hydroxy-6-methyl-2-oxo-2H-pyran-3-yl)-1H-benzo[f]chromene-2-carbonitrile (2g)
Yellow solid; 1.63 g (94%); mp: 177–178 °C; νmax (KBr) = 3411, 3331, 3210, 2160, 1703, 1654, 1584, 1425, 1410, 1238, 1083 cm−1; HRMS (ESI): 369.0851 [M + Na]+, calcd for C20H14N2NaO4: 369.0846; δH (300 MHz, DMSO-d6) 2.10 (s, 3H, CH3), 5.39 (s, 1H, CH), 5.98 (s, 1H, CH), 6.79 (s, 2H, NH2), 7.17 (d, J = 8.8 Hz, 1H, Ar), 7.36–7.53 (m, 2H, Ar), 7.82 (d, J = 8.8 Hz, 1H, Ar), 7.85–7.95 (m, 2H, Ar), 12.90 (br s, 1H, OH); δc (75 MHz, DMSO-d6) 19.3, 28.0, 53.3, 100.0, 104.0, 114.2, 116.6, 120.9, 122.7, 123.6, 124.6, 127.0, 128.6 (2C), 130.4, 130.8, 147.9, 161.3, 161.5, 163.3, 166.0.
Acknowledgements
The authors gratefully acknowledge the financial support of the Presidential Scholarship Program for the State Support of young Russian scientists–PhD (project No. MK-899.2014.3).