1 Introduction
The growing anxiety about the sharp reduction in fossil fuel resources and the effect on climate change resulting from the increasing rates of emissions have led to great interest in biomass utilisation as a renewable source of energy and other valuable materials [1,2]. Particularly, the carbohydrates obtained from lignocellulosic biomass are a promising and abundant source of green energy, as they are obtained from non-edible biomass and are relatively inexpensive [3–5]. The numerous studies conducted in this field revealed that 5HMF is a very useful multiple source of chemical building block material of green fuels and valuable chemicals, which can be obtained directly from carbohydrates [6–8].
5-Hydroxymethylfurfural (5HMF) is a heterocyclic furanic compound consisting of two functional groups of hydroxide and aldehyde in the positions 2 and 5 in the furan ring. As shown in Fig. 1, 5HMF is produced from biomass resources through a variety of carbohydrates and it has useful downstream derivatives in the form of chemicals, pharmaceuticals, bioplastics and biofuels.
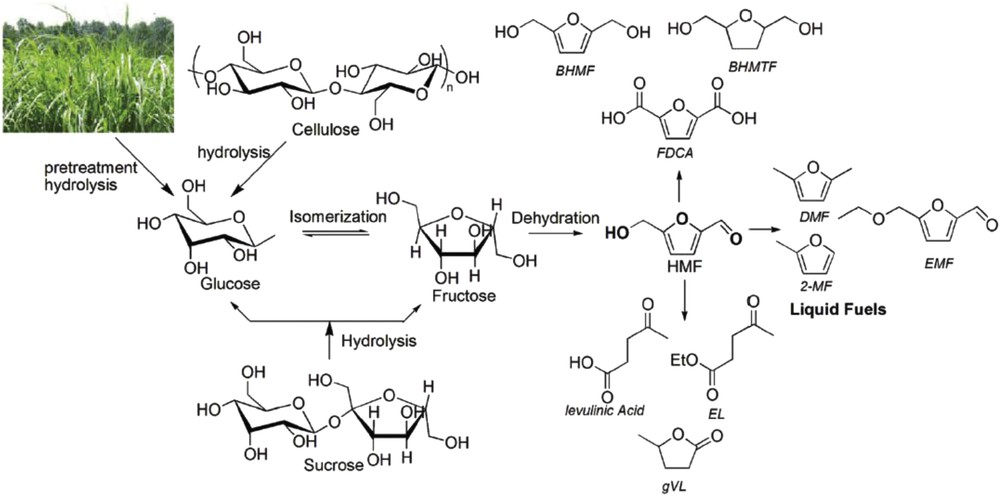
Production of 5HMF from biomass resources and its useful derivatives [9].
Carbohydrates and particularly fructose were dehydrated to 5HMF in different reaction media such as aqueous systems [10], biphasic systems [9] and ionic liquids [1]. The use of all previously mentioned systems in biomass utilisation was inhibited by several drawbacks such as low 5HMF yields, solvent-related environmental aspects, high cost, and the requirement of severe reaction conditions.
The production of 5HMF from carbohydrate started early in the 19th century by employing aqueous systems. Different mineral and organic acids were screened in carbohydrate dehydration to 5HMF. In recent screening for different mineral acids; the best 5HMF yield reported was 65.3% when phosphoric acid was used as a catalyst for fructose dehydration to 5HMF in subcritical water [11]. The use of aqueous systems in carbohydrate dehydration was limited by different challenges such as severe reaction conditions, and 5HMF rehydration to levulinic and formic acids caused by the presence of water as a solvent [12].
To overcome these drawbacks, great interest was shown in the use of biphasic systems in order to eliminate the rehydration of 5HMF by continuous extraction from the aqueous phase. Different organic solvents were combined with aqueous systems for this purpose. Roman Leshkov used a biphasic system to dehydrate fructose to 5HMF in an aqueous system catalysed by HCl, involving a mixture of MIBK and 2-butanol as an organic phase for continuous extraction; 80% yield of 5HMF was obtained [6]. The development of this system was limited by the disadvantages of some organic solvents, such as the ecological effect and the high boiling point of some solvents made the serration of 5HMF energy intensive, in addition to the high cost of organic solvents.
Ionic liquids were also involved in carbohydrate dehydration to 5HMF using a variety of catalysts. Fructose dehydration in [Bmim]Cl, catalysed by ion exchange resins resulted in a 5HMF yield of 86.2% obtained at 75 °C and 20 min [13]. Sucrose dehydrated to 5HMF in [Emim]Br, and catalysed by using an amino acid, achieved a 5HMF yield of 76% [14]. Even though these examples explain that dehydration of carbohydrates by ionic liquids could be achieved at mild reaction temperatures in high yields of 5HMF, the high cost of ionic liquids is still a challenge in developing their use in carbohydrate dehydration chemistry.
Lately, a new kind of self-catalysed green solvents namely Deep Eutectic Solvents (DESs) were explored by Abbott [15].
A DES is a mixture formed by mixing quaternary ammonium salts with hydrogen bond donors such as amides, amines, alcohols or carboxylic acids [16].
The first DES form was made of a mixture of choline chloride as organic salt and urea as the hydrogen bond donor (HBD) [17].
The principle of DES mixture is an ionic interaction between the hydrogen bond donor and the organic salt. This reduces the electrostatic forces between the anions and the cations, which lead to a decrease in the freezing point of the mixture [18].
DESs are considered as a type of conventional ionic liquids. In addition, they share many properties like low volatility, non-flammable, high thermal stability and non-toxicity. They are cheaper than conventional ionic liquids, easy to prepare, biodegradable, non-reactive with water, have low melting point and high viscosity and no further purification is needed [19].
Therefore DESs were involved in a wide range of applications; in addition to their use as alternatives to ionic liquids, they were used in enzymatic catalysis, molecular purifications, electrochemistry and bio catalysis processes [20].
Previous studies reported the use of DES in fructose dehydration to 5HMF. Liu et al. have reported a 72% yield of 5HMF from fructose dehydration in a DES composed of CO2 and choline chloride at 120 °C [21]. Fructose dehydrated in different Deep Eutectic Solvents (DES) such as ChCl/oxalic acid, ChCl/malonic acid and ChCl/citric acid, involving ethyl acetate as an extraction solvent. The highest 5HMF selectivity obtained was 83.3% with a conversion of 91.9% when a DES composed of ChCl/citric acid was used at 80 °C and 1 h of reaction time. Also, it was found in this study that the use of an extraction solvent promoted the 5HMF yield by 9% compared to the run conducted in a monophasic system (without adding ethyl acetate) [22]. Later, the same author reported that inulin was converted to 5HMF using two DES systems composed of ChCl/oxalic acid and ChCl/citric acid in a biphasic system composed of ethyl acetate, and a 5HMF yield of 64 and 55% was obtained from the above mentioned systems, respectively [23]. Zhao used Nano size Ly3-xHxPW as an acid-base hetero-poly-acid (HPA), to catalyse fructose dehydration to 5HMF in a DES mixture composed of ChCl and fructose, and a 5HMF yield of 92.3% was obtained with a fructose conversion of 93.3% at 110 °C and 1 min [24]. Recently, the authors have reported fructose dehydration to 5HMF by using a facile acidic choline chloride with p-TSA as a green DES. A 5HMF yield of 90.7% was obtained [25].
This work investigates the fructose dehydration by using a novel self-catalysed green DES mixture composed of N,N-diethyl ethanol ammonium chloride as organic salt and p-toluene sulfonic acid monohydrate (p-TSA) as a hydrogen bond donor (HBD). The examined DES was found to be an efficient, cheap, environmentally friendly and renewable catalytic mixture. An 84.8% yield of 5HMF was obtained.
2 Experimental
2.1 Materials
p-Toluenesulfonic acid monohydrate (p-TSA) (CH3·C6H4·SO3H·H2O) 98.5%, calcium hydroxide Ca(OH)2 96% and fructose (C6H12O6) 99% were ordered from Sigma Aldrich. N,N-diethylethanolammonium chloride (C6H16ClNO), 98% and HPLC grade water were from VWR. All materials were used with no further purification.
2.2 DES mixture preparations
The DES mixture consisted of N,N-diethylethanolammonium chloride and p-toluenesulfonic acid monohydrate (p-TSA); both materials were dried prior to use at 70 °C under vacuum for 2 h. Afterwards both materials were mixed together at different molar ratios in a 50 mL stainless steel cylindrical vessel then stirred by using a magnetic stirrer at 300 rpm and heated at 80 °C by using an oil bath for 30 min. A colourless mixture was produced as shown in Fig. 2. A similar DES was used in free fatty acid treatment and synthesis of biodiesel [26]

The DES mixture.
2.3 Fructose dehydration reaction procedure
Fructose dehydration reactions were carried out in a 50 mL stainless steel vessel, using an oil bath as a heating source and by stirring using a magnetic stirrer at 300 rpm for 1 h at 80 °C. At the end of the reaction, a brown mixture was produced as illustrated in Fig. 3. In terms of eliminating the occurrence of side reactions, at the end of the reaction, the produced mixture was quenched in an ice bath to be cooled down rapidly. Afterwards the mixture was diluted in 10 mL of HPLC grade water, then filtered and bottled for HPLC analysis.
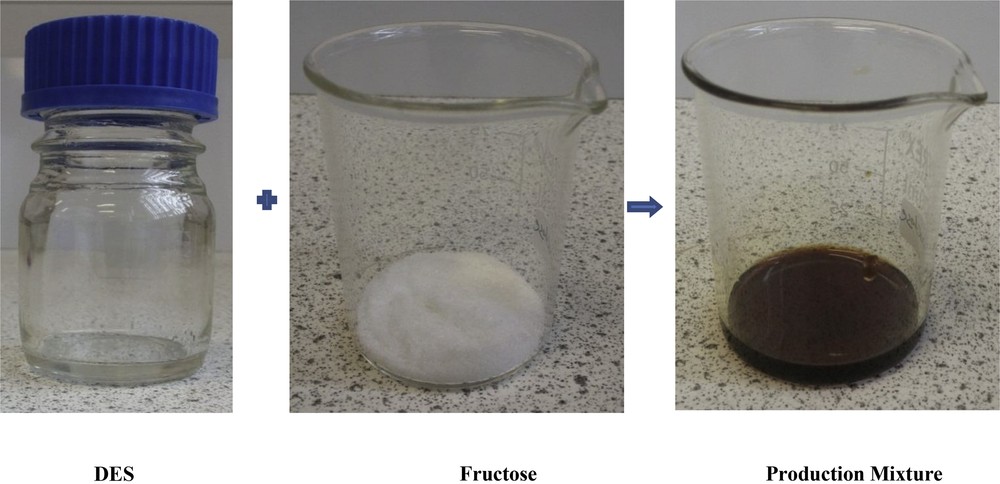
Fructose dehydration reaction in DES.
3 Results and discussion
3.1 The effect of feed ratio
The initial ratio of fructose has a great effect on the 5HMF yield and selectivity. As shown in Fig. 5, the 5HMF yield and selectivity gradually decreased by increasing the initial fructose ratio. This could be attributed to the rehydration of the produced 5HMF, caused by the presence of water generated from the fructose dehydration reaction. This could be controlled by implementing an efficient separation technique to remove the produced 5HMF from the reaction mixture such as using a bed of silica. The highest yield of 5HMF obtained was 70.5% at a feed ratio of 2.5, with a selectivity of 76.7%. When the feed ratio increased from 5 to 20, the 5HMF yield decreased from 66.4% to 45% with a 5HMF selectivity of 67.9% and 48.4%, respectively.
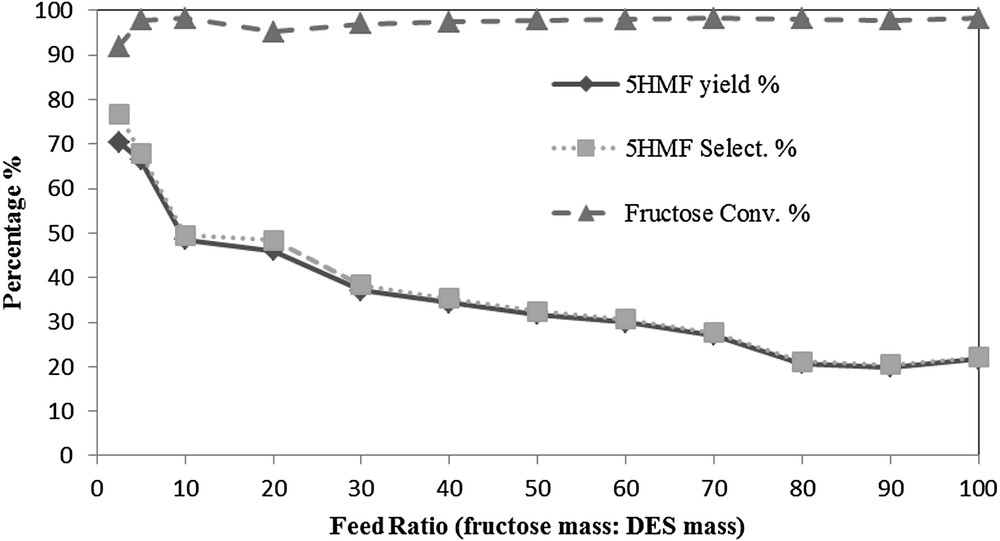
The effect of the feed ratio at a reaction time of 1 h, reaction temperature of 80 °C, and DES mixing ratio 1:1 (w/w).
Further increase in the feed ratio led to a further decrease in the 5HMF yield and selectivity. The lowest 5HMF yield obtained was 21.7% with a selectivity of 22.1% at a feed ratio of 100. As the 5HMF yield was unstable at an initial fructose ratio of 2.5, due to the possibly insufficient mass of fructose, the ratio of 5 was selected for optimising the process.
At this point it is worth mentioning that the sample colour changed to dark brown upon increasing the initial fructose ratio, which could indicate the occurrence of side reactions possibly producing humins and polymers. Humins are dark brown solid compounds that appear as a by-product in sugar dehydration reactions. As shown in Fig. 4, this occurs as a result of 5HMF condensation by the water produced from the reaction, which subsequently led to a reduction in the 5HMF yield.

Fructose dehydration reaction pathway.
3.2 The effect of reaction temperature
As shown in Fig. 6, the effect of reaction temperature on fructose dehydration reactions using a DES mixture of N,N-diethylethanolammonium chloride and p-toluene sulfonic acid monohydrate (p-TSA) was investigated. The following reaction conditions were used: a DES mixing ratio of 1:1, reaction time of 1 h, feed ratio of 5% and reaction temperature range of 50 °C to 110 °C. Investigation of the effect of reaction temperature with a range of 50 °C to 90 °C revealed that there was no significant change in the 5HMF yield. It varied from 59.8% to 62.8%, while the 5HMF selectivity decreased from 86.2% at 50 °C to 68.9% at 80 °C. This could be attributed to the humin formation due to the occurrence of side reactions as a result of the thermal degradation of 5HMF and fructose. A further decrease in the 5HMF yield and selectivity is shown; when the reaction temperature was increased to 100 °C and 110 °C; the yield of 5HMF decreased to 53.9% at 100 °C with a selectivity of 57.3%.
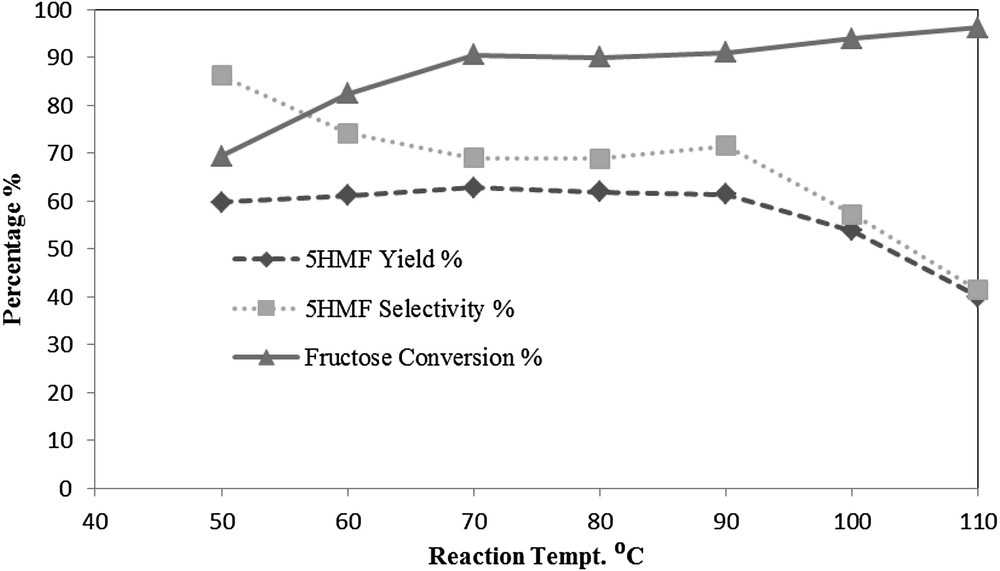
The effect of reaction temperature at the reaction time of 1 h, feed ratio of 5 (w/w) and DES mixing ratio 1:1 (w/w).
The lowest yield of 5HMF obtained was 40% at 110 °C, with a selectivity of 41.4%. Also, it was noticed that the sample colour was changed to dark brown by increasing the reaction temperature. As it is illustrated in fig, this may be attributed to the thermal degradation of 5HMF to humins upon increasing the reaction temperature. On the other hand, fructose conversion increased with the reaction temperature ranging from 69.4% at 50 °C to 96.3% at 110 °C. A reaction temperature of 80 °C was chosen as the optimum reaction temperature.
3.3 The effect of reaction time
The influence of reaction time on the 5HMF yield and selectivity, as well as the fructose conversion, was studied over the time range from 5 to 120 min. The study was carried out at 80 °C, at an initial fructose ratio of 5 and a 1:1 DES mixing ratio.
As expected, Fig. 7 reveals that the reaction time had a positive effect on the 5HMF yield and selectivity. The 5HMF yield varied from 58.8% to 66.5%, while the 5HMF selectivity was changing in a range from 62.1% to 78.4%. This may be attributed to the side reactions like rehydration of 5HMF as illustrated in Fig. 4, also the slight fluctuation in the 5HMF yield and selectivity as well as the fructose conversion increase over the time could be a sign of forward and back ward reactions. The fructose conversion was also effected by the reaction time as it was varying from 78.8% to 97.3.
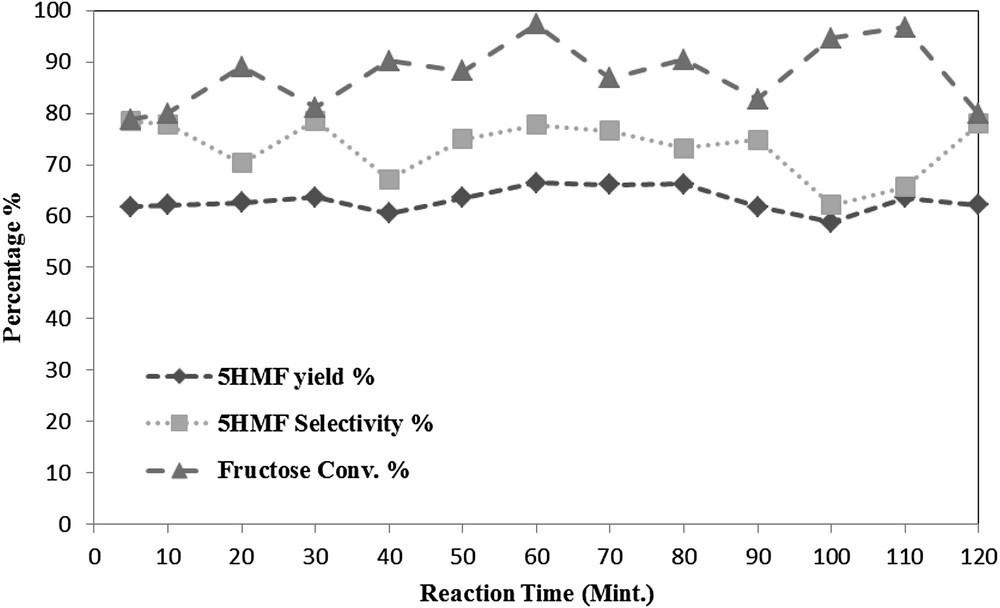
The effect of reaction time at a reaction temperature of 80 °C, DES mixing ratio 1:1 (w/w) and feed ratio of 5 (w/w).
Due to the notable effect of the reaction time on the fructose dehydration reaction, and the highest 5HMF yield obtained was 66.5% at a reaction time of 60 min, it was chosen as an optimum time in order to be comparable with a previous study carried out by using a different DES mixture composed of p-TSA and Choline chloride [25] with 1 h as the reaction time.
3.4 The effect of DES mixing ratio
The DES mixing ratio is a key variable for the production of 5HMF by this reaction. As the acid p-TSA is the active side in the DES, the acid ratio was changed every time, while the salt molar ratio was fixed at 1.
In this work different mixing molar ratios were investigated. They are 1:0.5, 1:1, 1:1.5 and 1:2 of N,N-diethylethanolammonium chloride to p-toluenesulfonic acid monohydrate (p-TSA). The DES mixing molar ratio had a significant effect on the 5HMF yield and selectivity.
As shown in Fig. 8, both the 5HMF yield and selectivity are sharply decreased upon increasing the p-TSA acid molar ratio. At a mixing molar ratio of 1:0.5 the highest 5HMF yield of 84.8% was obtained with a 5HMF selectivity of 88.6%. As the mixing ratio increased to 1:1; the 5HMF yield decreased down to 66.4%, with a 5HMF selectivity of 67.9%. When the mixing ratio was further increased to 1:1.5, a lower 5HMF yield of 45% was obtained with a 5HMF selectivity of 49.2%. The lowest 5HMF yield obtained was 21.1% with a lower 5HMF selectivity of 23% at a mixing ratio of 1:2.
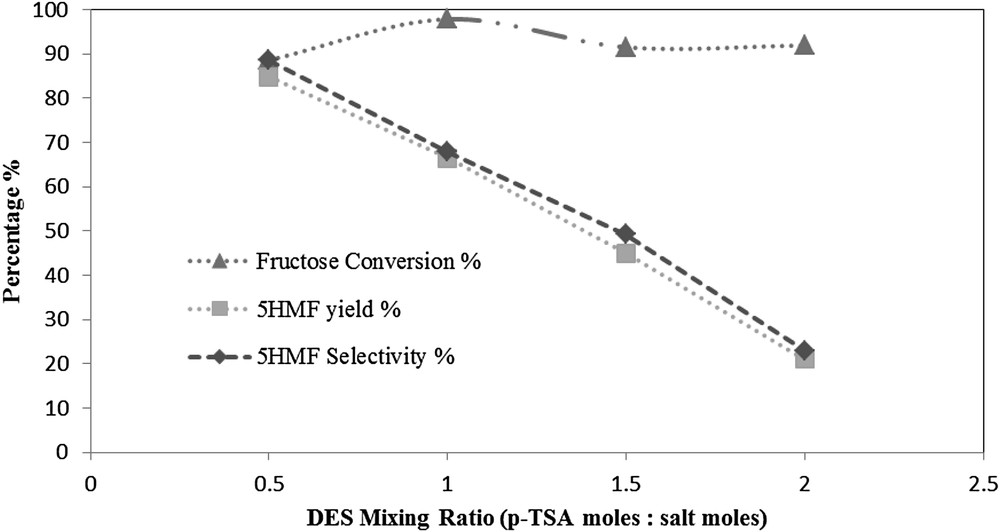
The effect of DES mixing ratio at a feed ratio of 5 (w/w), reaction temperature of 80 °C, and reaction time of 1 h.
The severe reduction in the 5HMF yield and selectivity can be attributed to the activation of side reactions caused by the acidic reaction medium as a result of increasing the acid content in the DES mixture. A previous study using different DES mixtures showed different trends of the effect on the fructose dehydration reaction [18]. This may be referred to the composition of the DES itself. The fructose conversion was varying between 88.6% and 97.8%.
4 Analysis
Both the reactants fructose and final product 5HMF were analysed by using a HPLC 1100 Agilent Series, using a refractive index detector and Rezex RCM-monosaccharide column with dimensions (300 × 7.8 mm). The column was ordered from Phenomenex.
The analytical method procedure used was 100% HPLC grade water as the mobile phase, at a flow rate of 0.5 mL per minute, column oven temperature of 75 °C, injection volume of 20 μL and total time of 40 min. The fructose and 5HMF peaks appeared at 16 and 35 min, respectively.
5 Mathematical equations
The used equations are as follows:
- 1.
- 2.
- 3. Fructose conversion
- 4.
- 5.
6 Conclusion
The use of a self-catalysed DES mixture composed of inexpensive N,N-diethylethanolammonium chloride and p-toluenesulfonic acid monohydrate (p-TSA) as a green solvent and catalyst has proven the validity and the high activity of the Deep Eutectic Solvents in the fructose dehydration reaction. In addition to the high yield of 5HMF obtained, the used DES was environmentally friendly, renewable, inexpensive, nontoxic, and non-flammable, has low vapour pressure and is easy to prepare. Most importantly, to perform the dehydration reaction in a DES does not require any addition of water, which would prevent the rehydration of 5HMF to lower levels. Moreover this system is catalysed in-situ with no need for any further addition of catalyst.
Also, it was found that the reaction can be performed under mild reaction conditions. The best yield of 5HMF obtained was 84.8% with a selectivity of 88.6% under optimised reaction conditions of a reaction temperature of 80 °C, reaction time of 1 h, DES mixing ratio of 1:0.5 salt to p-TSA and feed ratio of 5%. Further studies may be required for a robust separation system to purify the final product 5HMF and further analysis technique is needed to identify the by-products obtained from side reactions.
Vous devez vous connecter pour continuer.
S'authentifier