Version française abrégée
1 Introduction
Les chaı̂nes de collision sont caractérisées par des déformations ductiles pénétratives pendant la phase d'épaississement. Cette déformation se répartit dans des zones de cisaillement et dans le plissement à l'intérieur des unités tectoniques. Les plis sont parfois strictement associés aux charriages et en général, dans les portions les plus externes des chaı̂nes de montagne, les mécanismes de plissement sont bien compris. Par contre, peu d'informations existent sur ces relations dans les portions plus internes, plus métamorphisées et plus déformées de ces chaı̂nes. La zone à nappes de la chaı̂ne varisque en Sardaigne [3], qui n'est pas affectée par les déformations alpines, est dans une situation idéale pour étudier ces relations. Ici, en effet, les plis asymétriques de dimension kilométrique de l'Unité de Meana Sardo (UMS) (Fig. 1) peuvent être suivis et cartographiés en continuité entre les contacts anormaux de la base et du sommet de l'unité.

A Geologic sketch map of the Meana Sardo Unit in the Mt. S. Vittoria area and localization of the study area. 1: Quaternary covers; 2: Basalts (Pliocene–Pleistocene); 3: Conglomerates, sandstones and shales (?Upper Oligocene–Aquitanian); 4: Quartzites, sandstones, limestones and dolostones (Triassic–Jurassic); 5: Dikes (Permian–Carboniferous); 6: Conglomerates, sandstones and siltstones (Permian); 7: Quartzites, metasandstones, metasiltstones and phyllites of the Low Grade Metamorphic Complex of Barbagia (?Cambrian–Ordovician sup.). Meana Sardo Unit: 8: Metalimestones and metapelites (Silurian–Devonian); 9: Black shales and metalimestones (Silurian); 10: Quartzites, metasandstones, metasiltstones and metapelites (Upper Ordovician). Mt. S. Vittoria Formation (Middle Ordovician): 11: Metagreywakes and metandesites; 12: Metavolcanoclastics and metaconglomerates; 13: Metarhyolites and sericitic schists. Solanas-S. Vito Formation (Middle-Upper Cambrian–Lower Ordovician): 14: Quartzites, metasandstones, metasiltstones and metapelites; 15: Barbagia Thrust; 16: Main faults; 17: Trace of the geological cross-section in 1B. B Geological cross section throughout MSU. Reversed folds are clearly shown.
A Carte géologique simplifiée de l'Unité de Meana Sardo dans la zone du Mt. S. Vittoria et localisation de la zone étudiée. 1 : Dépôts de couverture quaternaires ; 2 : Basaltes (Pliocène–Pleistocène) ; 3 : Conglomérats, grès et shales ( ? Oligocène supérieur–Aquitanien) ; 4 : Quartzites, grès, calcaires et dolomies (Triass–Jurassique) ; 5 : Dykes (Permien–Carbonifère) ; 6 : Conglomérats, grès et siltites (Permien) ; 7 : Quartzites, métagrès, métasiltites, et phyllites du complexe métamorphique de bas degré de la Barbagia ( ? Cambrien–Ordovicien supérieur). Unité de Meana Sardo : 8 : Métacalcaires et métapélites (Silurien-Dévonien) ; 9 : Shales noirs et métacalcaires (Silurien) ; 10 : Quartzites, métagrès, métasiltites et métapélites (Ordovicien supérieur). Formation du Mt S.Vittoria (Ordovicien moyen) : 11 : Méta-andésites et métagrauwackes ; 12 : Métavolcanoclastites et métaconglomérats ; 13 : Métarhyolites et schistes sériciteux. Formation de Solanas–S. Vito (Cambrien moyen-supérieur–Ordovicien inférieur) : 14 : Quartzites, métagrès, métasiltites et métapélites ; 15 : Chevauchement de la Barbagia ; 16 : Failles principales ; 17 : Trace de la coupe géologique en 1B. B Coupe géologique à travers le UMS. Les plis couchés sont clairement représentés.
2 Cadre géologique
Le soubassement cristallin de la Sardaigne fait partie de la chaı̂ne varisque sud-européenne et il est constitué par des métasédiments du Cambrien–Ordovicien et par des roches magmatiques du Carbonifère inférieur [3]. Le degré métamorphique, très bas dans le secteur sud–ouest de l'ı̂le, devient moyen puis élevé dans la partie septentrionale [3]. Dans la zone étudiée, affleurent essentiellement des roches peu transformées, avec le charriage du Complexe Métamorphique de bas degré de la Barbagia (CMBDB) sur l'Unité de Meana Sardo (UMS) (Fig. 1). La succession commence avec des métasédiments terrigènes du Cambrien moyen-supérieur – Ordovicien inférieur ; ils sont suivis par un Complexe magmatique ordovicien avec métavolcanites et métavolcano-clastites riches en métaconglomérats, couronné par des métasédiments terrigènes et carbonatés fossilifères de l'Ordovicien supérieur. La succession continue avec les classiques schistes noirs à Graptolites, les métacalcaires à Orthocères du Silurien et les métacalcaires du Dévonien. La phase d'épaississement et de mise en place des unités tectoniques a produit deux événements déformatifs (D1 et D2), suivis par deux phases tardives (D3 et D4) et par des failles normales de faible ou fort angle [3,5]. Alors que les phases D1 et D2 se sont développées de manière hétérogène dans l'UMS, la deuxième phase D2 devient la plus importante et la plus évidente dans le Complexe de la Barbagia. La phase D3, origine des antiformes et des synformes à échelle plurikilométrique, déforme les contacts principaux tectoniques entre les deux unités.
Des plissements asymétriques D4 et des failles normales de faible angle accommodent le collapse extensionel ultérieur de la pile de nappes [5].
3 Données structurales
La phase D1 est à l'origine de plis asymétriques, à échelle mésoscopique et mégascopique, à vergence ouest–SW et direction axiale d'environ NW–SE. Les plis appartiennent aux classes 1C, 2, 3 de Ramsay [15]. La foliation S1 de plan axial, caractérisée par un métamorphisme de bas degré, montre une direction moyenne de N90–N140E et elle est inclinée modérément vers le nord–est. La linéation d'extension L1 a une direction N30E et une inclinaison d'environ 30° vers le nord-est.
Un deuxième événement D2 [5,6,9], clairement hétérogène, produit des bandes de cisaillement localisées et il peut arriver à transposer complètement la foliation S1. Les caractéristiques des phases D1 et D2 indiquent une déformation non coaxiale [5,9], qui augmente d'intensité vers la surface de charriage de la Barbagia. Dans ce dernier complexe, la foliation principale est définie par le clivage de crénulation S2.
Les cataclasites et les mylonites associées aux nappes de la Barbagia ont des épaisseurs variables, de 50–200 m, et sont constituées par des phyllades, quartzites et méta-arénites mylonitisées. Le ‘shear band cleavage’ [14] constitue l'indicateur cinématique principal et fournit un sens de cisaillement sommet vers le sud–ouest. Le contact tectonique inférieur, au-dessus des unités du Gerrei, fournit des sens de déplacement similaires.
4 Déformation finie et plis
Une analyse de la déformation finie a été effectuée sur les métaconglomérats ordoviciens dans dix localités autour du noyau du pli anticlinal de Domu e' Orxia (Fig. 1B). Les échantillons ont été sectionnés selon les plans XZ et YZ de l'ellipsoı̈de de la déformation finie (X⩾Y⩾Z) et un nombre variable de clastes (50–150) ont été mesurés pour chacune des sections. Les données sur la déformation ont été obtenues en utilisant les programmes Surfor et Paror [12,13]. Les points projetés sur le diagramme de Flinn sont plutôt dispersés et les valeurs de K varient entre 0,01 et 1,56. Les ellipsoı̈des de la déformation calculés présentent les axes majeurs parallèles au plan axial du pli, autant dans la charnière, que sur les flancs et la quantité de déformation augmente en passant de l'extrados à l'intrados du pli (Fig. 2).

Profile of Domu e' Orxia asymmetric anticline with calculated finite strain ellipsoids (XZ sections). On the right, XZ axial ratio values (RXZ) of finite strain ellipsoid have been reported. XY planes of finite strain ellipsoids are parallel to fold axial plane and deformation increases from the outer to the inner arc of the fold.
Profil de l'anticlinal asymétrique de Domu e' Orxia avec les ellipsoı̈des de la déformation finie calculée (sections XZ). Sur la droite sont représentées les valeurs des rapports axiaux XZ (RXZ). Les plans XY des ellipsoı̈des de la déformation finie sont parallèles aux surfaces axiales des plis et la déformation croı̂t à partir de l'intrados vers l'extrados de la charnière du pli.
5 Évolution du plissement et mécanisme associé
L'unité de Meana Sardo est constituée par une succession multi-couches de sédiments clastiques et volcanoclastiques, avec un faible contraste de compétence. Ceci suggère la présence d'un raccourcissement initial parallèle au litage avant la première apparition du plissement [16] (Fig. 3A). La distribution de l'ellipsoı̈de de la déformation finie à travers le profil d'un mégaplissement montre des valeurs de rapport axial sur la surface XZ, qui augmente à partir de l'extrados vers l'intrados et suggère donc un mécanisme de plissement, avec déformation de la charnière (‘neutral surface folding’ [16]), qui peut produire des plis de la classe 1B [15] (Fig. 3B). En même temps, la géométrie des plis, avec charnière épaissie et flancs amincis, suggère la présence d'un raccourcissement ultérieur. La superposition d'une déformation par cisaillement homogène (Fig. 3C), comme celle induite par le charriage de la Barbagia sur l'UMS, peut développer une telle géométrie. Dans ce cas, le cisaillement simple a été préféré à la déformation pure, parce qu' il est capable d'entraı̂ner une rotation des plis (les plans axiaux sont fortement inclinés). La quantité de cisaillement simple ne doit pas être très élevée pour ne pas éliminer la différence de déformation entre l'intrados et l'extrados des plis.
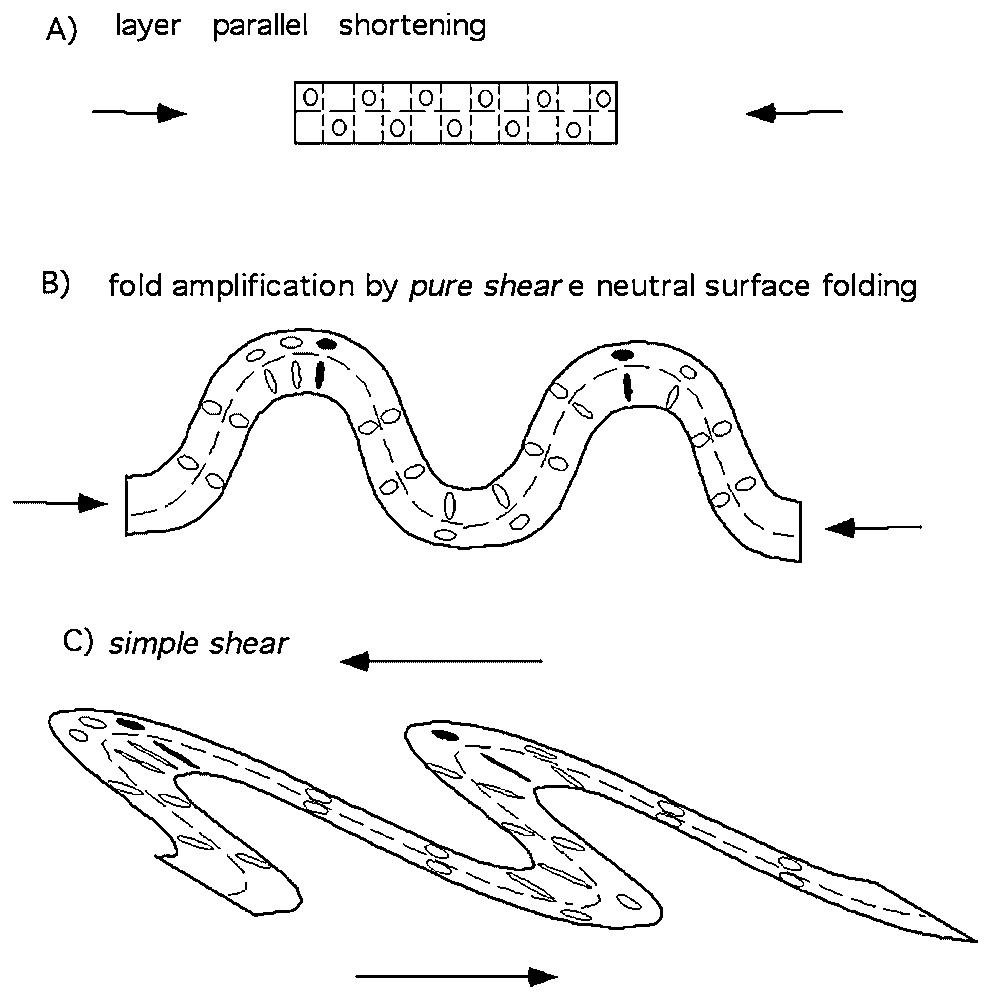
Evolutionary steps of folding event. A: initial parallel shortening with development of strain ellipsoids with the principal axis perpendicular to the strata; B: nucleation and amplification of upright parallel folds induced by neutral surface folding mechanism; C: folds are further shortened by a simple shear component (top-to-the southwest) and passively rotated toward the tectonic transport direction.
Étapes de l'évolution du plissement. A : raccourcissement initial parallèle à la stratification qui a développé des ellipsoı̈des avec axe principal orthogonal à la stratification ; B : nucléation et amplification de plis droits et parallèles par effet du plissement à surface neutre ; C : les plis sont ultérieurement raccourcis par une composante de cisaillement simple et passivement tournés vers la direction de transport tectonique.
6 Relations entre les plis asymétriques et les charriages
Les plis asymétriques qui se sont formés pendant la déformation D1–D2 dans l'UMS montrent une longueur d'onde progressivement décroissante, quand on se déplace vers le contact de base de la nappe de la Barbagia. Même les linéations d'extension dans les cataclasites et les mylonites liées au charriage et visibles sur la foliation de plan axial des plis F1–F2 ont la même distribution.
Sanderson [19], Ramsay et al. [17] et Casey et Huggenberger [8] expliquent la formation des plis asymétriques par un cisaillement simple à bas angle par rapport à la stratification initiale. Rowan et Kligfield [18] ont développé un modèle pour la formation de plis asymétriques confinés entre les surfaces tectoniques des Nappes helvétiques, où ils suggèrent un raccourcissement parallèle au litage suivi par un cisaillement simple. Pour tester l'applicabilité de ce modèle au pli de l'UMS, un groupe de plis droits avec une ouverture de flancs de 100–110° a été construit et progressivement déformé par de petits incréments de cisaillement simple (de γ=1 à γ=6 ; Fig. 4) avec le software MATHEMATICA 3.1 (®Wolfram research (11)). L'étape correspondant à γ=1,73 est capable de donner naissance à un train de plis avec un angle d'ouverture de 66° et un plan axial incliné de 60° avec une géométrie similaire à celle des plis de l'UMS. Le rapport axial de l' ellipsoı̈de de la déformation finie sur la section XZ (avec X⩾Y⩾Z), qui correspond à 5,8, est compatible avec les mesures sur le terrain, qui varient de 1,6 à 5.
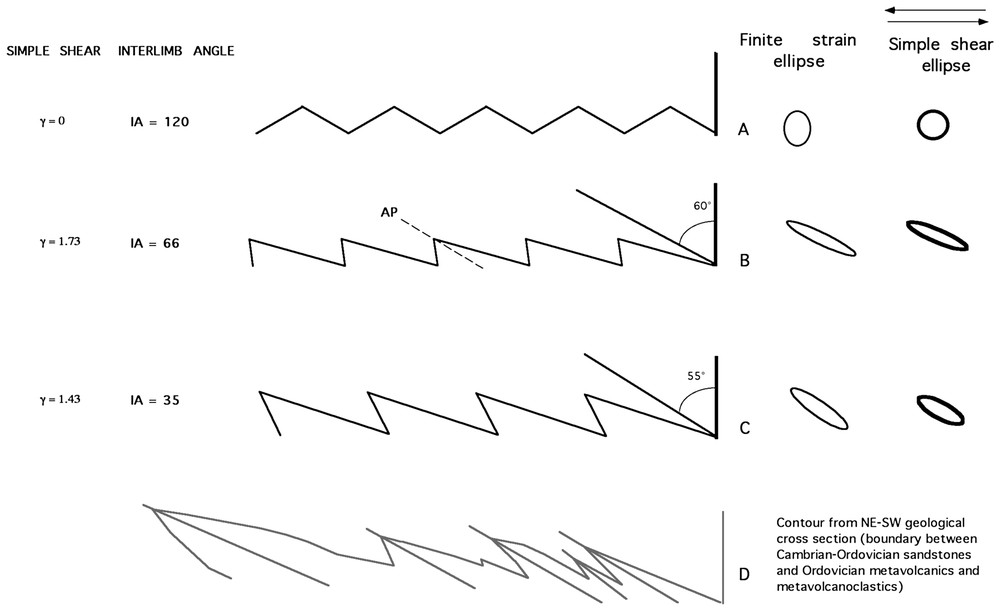
Schematic diagram, based on the bidimensional model of Rowan and Kligfield [18], applied to the development of asymmetric folds of MSU. A: initial status with upright folds with interlimb angle (IA) of 120° (γ=0, R=1); B: finite geometry obtained by the superposition of an incremental simple shear component (γ=1.73, R=4, sinistral shear sense in the figure) giving rise to an interlimb angle of 66°; C: finite geometry obtained by the superposition of contemporaneous simple and pure shear (γ=1.43, R=1.5, sinistral shear sense in the figure) giving rise to an interlimb angle of about 35°; D: geological profile of the folds observed in the field.
Diagramme schématique, développé d'après le modèle bidimensionnel de Rowan et Kligfield [18] et appliqué au développement des plis asymétriques de l'UMS. A : condition initiale avec des plis droits ayant un angle d'ouverture (IA) de 120° (γ=0, R=1) ; B : géométrie finie obtenue par la superposition d'une composante incrémentale de cisaillement simple (γ=1,73, R=4, dans la figure, avec un sens de déplacement vers la gauche) donnant un angle d'ouverture final (IA) de 66° ; C : géométrie finie obtenue par la superposition simultanée d'un cisaillement simple et un raccourcissement (γ=1,43, R=1,5, dans la figure avec un sens de cisaillement sénestre) en donnant un angle entre les flancs d'environ 35° ; D : coupe géologique des plis observés sur le terrain.
Des plis avec des géométries similaires à celles observées ont été obtenues en appliquant en même temps un raccourcissement (R=1,5) et un cisaillement simple (γ=1,43). En effet, même dans ce cas, les plis obtenus présentent une inclinaision des plans axiaux, des flancs reversés et des angles d'ouverture très similaires à la section naturelle. En outre, la valeur de R correspondante (Rx/z=4,35) ce rapproche très bien à celles calculées à partir des données du terrain.
7 Conclusion
La déformation principale de la Zone de Nappes de Sardaigne centrale est répartie en plis et charriage à vergence sud–ouest. L'UMS est caractérisée par des plis asymétriques de dimensions kilométriques, confinés entre un contact anormal supérieur (charriage de la Barbagia) et un contact anormal inférieur.
La géométrie, la cinématique et la déformation finie mesurée sur ces mégaplis ont permis de décomposer le développement des plis asymétriques en trois étapes :
- • un raccourcissement initial, parallèle aux stratifications, avec un faible contraste de compétence ;
- • le développement de plis à surface neutre afin d'atteindre un angle d'ouverture de 100–110° ;
- • un cisaillement simple, ou un cisaillement simple et racoursissement simultan, qui entraı̂ne une déformation passive et une rotation des plis vers le sud–ouest.
1 Introduction
Collisional belts show evidence of pervasive ductile deformation during the thickening stage. At the scale of the whole belt, deformation is usually partitioned into thrusts and/or shear zones and internal folding in the tectonic units. Nevertheless, folds are often associated to thrusts in the more external portions of the orogens and the fault-bend fold and fault-propagation fold models can easily explain asymmetric large scale folds in the more external areas of the orogens.
Comparatively few data exist on the relations between folds and thrusts or shear zones in the inner portion of the orogens where metamorphism and deformation increase, even if they represent large portions of the belts. Asymmetric large-scale folds have been documented both in compressional orogens [8,17–19] and in regional extensional deformation [2,10].
The Nappe Zone of the Variscan belt in Sardinia [3] represents a good place to investigate such relations, because the Paleozoic basement is poorly or not involved at all in the subsequent alpine deformations, and in addition it exhibits good outcrops and few post-Variscan covers.
We have chosen the area around the Mt. S. Vittoria (central Sardinia) (Fig. 1A) because here the Variscan structures are well exposed at all scales. In addition, kilometric-scale folds in Meana Sardo Unit (MSU) (Fig. 1) can be mapped with continuity from the upper thrust contact down to the lower tectonic boundary [6,7].
2 Geological setting
The crystalline basement of Sardinia is a segment of the Southern European Variscan belt, made up of Carboniferous magmatic and sedimentary rocks and metasedimentary Cambrian to Lower Carboniferous rocks with metamorphic grade increasing from very low-grade in the southwest side of the island up to medium- and high-grade in the northern sector [3]. Southward propagating polyphase deformation and metamorphism occurred during the collisional stage [3, and quoted references].
The low grade metamorphic rocks, cropping out in the investigated area, belong to the Nappe Zone, which extends from the suture zone in the north [1], to the external zone in the southwest [3]. In central Sardinia, the Low Grade Metamorphic Complex of Barbagia (LGMCB) overlies the MSU (Fig. 1), which in turn is thrust onto the Gerrei Units. The Barbagia Thrust separates the former two units and extends from central to southeastern Sardinia for more than 50 km. The minimum displacement, from the front of the nappe to the inner tectonic window in the higher Flumendosa Lake (Gennargentu Mountains), is at least 20 km.
The oldest sequences consist of metaconglomerates, metasandstones, metasiltstones and quartzites of Middle Cambrian – Lower Ordovician. Ordovician magmatic complex is made up by metavolcanics, varying in composition from basalt to rhyolite, and by metavolcanoclastics with a large amount of metaconglomerates. Quartzites, metarkoses, metaconglomerates, fossiliferous metasiltstones and metalimestones of Upper Ordovician occur at the top of the sequence. Silurian is represented by Graptolite-bearing black shales, lydites and Orthoceras-bearing metalimestones. Nodular metalimestones and calc-schists characterize the Devonian [3].
Overprinting criteria allowed us to recognize four deformative phases in the study area [3,5]. D1 and D2 deformation phases developed during the nappe transport and are followed by later deformation phases (D3 and D4), and by low- and high-angle normal faults. While D1 and D2 deformations are heterogeneously developed in MSU, D2 deformation becomes the more prominent deformation in LGMCB. D3 deformation is responsible for the formation of large-scale antiforms and synforms, affecting the nappe pile and the main thrusts. D4 asymmetric folds and extensional ductile/brittle shear zones accommodate the late tectonic extensional collapse of the nappe pile [5].
3 The earlier deformation phases
D1 deformation phase folds the sedimentary bedding and originates tight to isoclinal, mesoscopic and megascopic folds, facing to the west and southwest (Fig. 1). They are non-cylindrical and belong to the 1C, 2 and 3 classes of Ramsay [15]. F1 fold axes are shallowly plunging and are quite scattered with a main NW–SE trend.
S1 axial plane foliation strikes from N90E to N140E and moderately dips toward the northeast. It is a spaced disjunctive cleavage [22]. Synkinematic growth of quartz, albite, chlorite, calcite, white micas and oxides on S1 foliation indicate low-grade metamorphism.
The L1 stretching lineation is a discrete structural lineation and mineral grain lineation [22], trending mainly N30E and plunging about 30° toward northeast.
A second deformation event (D2) is often recognisable [5,6,9]. It generates localised shear band cleavage (from centimeters to a dozen meters), usually well developed in the less competent rocks. It can transpose the previous structures at the point, so that it becomes the prominent foliation and cannot be distinguished from the D1 deformation, at least at the outcrop scale. Small-scale intrafolial similar folds with strongly scattered axial trends can be sometimes recognized.
Structural features of D1–D2 deformation phases suggest the presence of a non-coaxial deformation [5,9]. The intensity of strain increases going toward the Barbagia Thrust, where strain localizes in thick cataclastic/mylonitic levels. The folds become more and more tight, the stretching lineation is more pervasive and finite strain values are higher [4]. It is worth noting that F1 folds wavelength decreases from one kilometer, at the base of the unit, to nearly 50–100 meters approaching the Barbagia Thrust [6].
In the LGMCB, D2 deformation transposes all the previous structures and reversed to recumbent F2 folds develop at the meso- and at the microscale (classes 1C-2, [15]). They are often non-cylindrical and transected [5]. F2 folds axes trend N60E–N70E. L2 stretching lineation trends mainly N20E–N30E. S2 axial plane foliation is an evoluted discrete crenulation cleavage.
4 Kinematic indicators
The Barbagia Thrust is characterized by thick cataclasites and mylonites (up to ∼200 m). The sheared rocks are mainly phyllites, quartzites and metasandstones. The most evident kinematic indicator is a shear band cleavage [14]. Intersection between S and C surfaces are usually perpendicular to the stretching lineation. S surfaces deform a pre-existing penetrative foliation developed under low-metamorphic grade. All the recognized kinematic indicators indicate a top-to-the southwest and, sometimes, west sense of shear.
Micro- and mesoscopic asymmetric folds show stretched limbs and thickened hinges with a general geometry compatible with a NE dipping strain ellipsoid, related to top-to-the southwest sense of shear. Fault rocks are characterized by the abundance of deformed quartz-veins, showing different amount of strain. Sigmoid quartz veins are often recrystallized and quartz frequently shows polygonal texture.
The tectonic contact of the MSU over the underlying Gerrei Unit also shows a top-to southwest sense of shear.
5 Finite strain and folds
Finite strain analysis has been performed in 10 localities from the hinge zone of a kilometer-scale anticline to better constrain folding mechanism. Ten field-oriented samples of Ordovician metaconglomerates and lithic metagreywackes were collected from the Domu e' Orxia anticline (Figs. 1B, 2). Samples have been accurately chosen in order to avoid the effects of later deformation phases. Clasts, flattened on the foliation plane and elongated parallel to the stretching lineation measured in the surrounding matrix, are suitable strain markers. The samples were sectioned along the principal planes of the finite strain ellipsoid (axes X⩾Y⩾Z); two sections were analysed (XZ and YZ), the third has been calculated from the other two. The number of the strain markers ranges from 50 to 150 for each section. Surfor and Paror [12,13] two-dimensional strain analysis methods were applied on each section.
Finite strain results are reported in Table 1 and Fig. 2. XZ axial ratios range from nearly 1.6 to 5.0. Strain ellipsoids have K values ranging from 0.01 to 1.56 and the points on the Flinn graph are quite scattered showing that the deformation pattern is complex and it deviates from plane strain.
Results of the finite strain analysis applied on 10 samples collected along the profile of Domu e' Orxia anticline. In the table are reported the principal axial ratio (RXZ,RYZ,RXY), the orientation of the principal axis X (azimuth and plunge), the K Flinn parameter, the ρ parameter of the Flinn diagram showing the distance of the calculated ellipse from the origin, indicative of the amount of deformation
Résultat de la déformation finie appliquée sur 10 échantillons pris à travers la coupe de l'anticlinal de Domu e' Orxia. Sur le tableau ont été reportés les rapports des axes principaux (RXZ,RYZ,RXY), les orientations de l'axe principal (direction et immersion), le paramètre K de Flinn et le paramètre ρ du diagramme de Flinn qui représente la distance à l'origine de l'ellipse calculée, qui indique la quantité de déformation
No | sample | R XZ | R YZ | R XY | az/pl X axis | K | p |
1 | S45 | 2.62 | 1.49 | 1.76 | 050-30 | 0.15 | 0.9 |
2 | S66 | 3.24 | 1.63 | 2 | 082-30 | 1.56 | 1.182 |
3 | MSV | 3.59 | 2.37 | 1.51 | 044-43 | 0.37 | 1.46 |
4 | MSVB | 3.43 | 2.05 | 1.67 | 018-22 | 0.64 | 1.245 |
5 | S48 | 3.14 | 2.26 | 1.39 | 056-20 | 0.31 | 1.32 |
6 | S68 | 5.06 | 4.16 | 1.22 | 076-30 | 0.07 | 3.167 |
7 | SA15A | 1.95 | 1.47 | 1.33 | 043-18 | 0.73 | 0.574 |
8 | SA18A | 1.66 | 1.65 | 1.01 | 082-30 | 0.01 | 0.650 |
9 | SA20A | 1.59 | 1.26 | 1.26 | 049-26 | 1.0 | 0.367 |
10 | SA22A | 1.76 | 1.53 | 1.15 | 348-67 | 0.32 | 0.551 |
The long axes of the finite strain ellipsoids are parallel to the axial plane both in the limbs and in the hinge zone of the folds and strain increases from the external arc toward the inner arc of the fold (Fig. 2).
6 Folding mechanisms and evolution
The geometry of the asymmetric folds and the finite strain pattern allow us to better constrain their evolution. MSU is made by a multilayer of clastic and volcanoclastic sediments, with low competence contrast, as suggested by little refraction of the foliation crosscutting sedimentary boundaries. As a consequence the multilayer should have undergone initial layer parallel shortening before fold amplification [16] during D1 deformation. In this stage the strain ellipsoids have long axes perpendicular to the layers (Fig. 3A).
The strain pattern observed in the hinge zone allows us to exclude flexural flow mechanism, according to which the lowest strain values are expected to be formed in the hinge zone [16]. In addition to this, the higher strain values in the inner arc of the fold hinge suggest the occurrence of tangential longitudinal strain (neutral surface folding; [16]).
The superposition (stage B; Fig. 3B) of tangential longitudinal strain (characterized by layer-parallel strain ellipsoids in the outer arc and layer-perpendicular strain ellipsoids in the inner arc), on initially vertical strain ellipsoids from the stage A) is the origin of the following pattern: in the outer arc, strain ellipsoids of the stages A and B are perpendicular and finite deformation decreases, whereas in the inner arc the strain of the two stages is additive and the finite strain increases. Hence here we expect to find the most elongated finite strain ellipsoids.
Due to the observed fold geometries characterized by thickened hinges and thinned limbs, a further stage (C) should be superposed onto the parallel folds, with vertical axial planes, generated during the stages A and B. A superposed homogeneous deformation by simple shear, or a simultaneous simple and pure shear, is able to passively deform the folds in such a way (Fig. 3C). The amount of strain should be sufficiently high to allow the finite strain ellipsoids in the outer arcs to completely unstrain and to be newly deformed perpendicular to the layers, as we can see in the observed pattern. The deformation history on the inner arc is characterized by increasing deformation always in the same direction, so the finite strain in this area will exhibit the finite strain ellipses with the highest axial ratio.
The strain difference between outer and inner arc is recorded also in the stage C, at the end of the folding history. Such a difference could disappear only in the event of a deformation in stage C, with strain values much higher than those that were assumed. The stage C corresponds to the prevailing simple shear deformation during the southwestward movement of the Barbagia Thrust, inducing passive deformation in the MSU.
7 Relation between asymmetric folds and thrust
The asymmetric folds in MSU have originated during the D1–D2 non-coaxial deformation. The observed decreasing of fold wavelength approaching the Barbagia Thrust suggests a close relation between them. In addition, the attitude of the stretching lineation in cataclasites/mylonites is the same as the stretching lineation observed throughout the tectonic unit.
According to Sanderson [19], Ramsay et al. [17] and Casey and Huggerberger [8] asymmetric large scale folds can be generally referred to simple shear acting at low angle respect to the bedding or metamorphic foliation. Rowan and Kligfield [18] developed a model for the development of asymmetric detachment folds between nappe boundaries in the Helvetic Nappes, with layer parallel shortening followed by rotation and tightening as strain increases. The first step is the initiation of upright folds in bedding at low angle to the overthrusts; they can be mechanically amplified until interlimbs angles approach 100° [17]. Further tightening and passive rotation occur by simple shearing [18]. This model could well explain the origin of the asymmetric folds in MSU. To check its applicability to the study area, a set of upright folds with variable interlimb angles has been deformed by progressive simple shear, and alternatively by contemporaneous simple and pure shear (using the matrix equations of [20,21]), both generated by matrix multiplication with the computer application MATHEMATICA [11]. Starting from amplified folds with interlimb angle of 100–120° small increments of simple shear have been added from γ=1 up to γ=6 (Fig. 4). The stage corresponding to a total shear strain γ∼1.7 produces interlimb angles of 66° and fold axial plane dipping 60° matching the geometry of the natural folds in MSU (Fig. 4). In this stage, strain ellipse Rx/z axial ratio by shear strain is 4 whereas finite strain ellipse Rx/z axial ratio is 5.8. These values are in keeping with those of the strain ellipses shown in the geological cross sections (Fig. 2) and observed in the field. Finite strain results are in general agreement with the theoretical values (measured XZ ratios vary from 1.6 to 5). The slightly lower values are due to competence contrast between clasts and their matrix.
To further check the model, a geological cross-section was de-strained by the application of an opposite simple shear of the same value γ=1.7. After this, the folds reach interlimb angles close to those indicated by Ramsay et al. [17], used by us as starting figure for incremental simple shear superposition.
However a slight difference arises when comparing the dipping of theoretical and natural reversed limb of folds.
Another possibility to realize the same geometry of folds comes from the simultaneous application of pure and simple shear to buckled folds [20,21]. Applying a simple shear with γ=1.43 contemporaneously with a pure shear with axial ratio of 1.5 gives rise to fold approaching the observed fold geometry (Fig. 4C). This solution originates folds with reverse limbs and axial planes matching the natural ones and show interlimb angles smaller than 66° previously determined. In addition finite strain axial ratio (Rx/z=4.35) is in agreement with the measured finite strain values detected across fold profile. Considering the variable values of interlimb angles measured on the natural cross section, ranging from 30 to 60°, the two proposed solutions are equally possible.
8 Conclusions
Deformation in the Nappe Zone of central Sardinia is partitioned in southwestern verging thrusts at the boundary between tectonic units and southwestern facing folds within the tectonic units. MSU, characterized by southwest facing kilometer-scale asymmetric folds, is confined between the upper Barbagia Thrust and the lower thrust onto the Gerrei Units. The geometry of the folds, the observed finite strain pattern and a 2-D model of development of asymmetric fold allow to explain their origin by three main stages:
- (i) initial layer parallel shortening in multilayer with little competence contrast;
- (ii) active fold amplification by neutral surface folding until the interlimb angle approaches 100–110°;
- (iii) simple shearing or contemporaneous pure and simple shearing, due to the overthrust of the LGMCB onto MSU caused passive further deformation of the folds in stage (ii).
Acknowledgements
This work has been supported by funds from the MIUR and University of Pisa. R. Carosi wishes to thank Enrico Tavarnelli for useful suggestions during the earlier stages of the work. M. Vanossi is thanked for a careful review of the manuscript.