1 Introduction
The Holocene, the current warm interglaciation, appears as a relatively stable climatic period when viewed in a long-term perspective. However, significant climatic variations have been evidenced over the continents. In Fennoscandia, it is generally accepted that climate became warmer shortly after the deglaciation and that a broad climatic optimum in mid-Holocene was followed by a declining climate [15]. Superimposed over this broad trend, glaciological, tree-ring and lake-sediment studies showed numerous climatic oscillations [20]. In the tropics, Holocene climatic variations result in abrupt hydrologic changes in regions influenced by the modern monsoon ([12] and references therein).
Recent years have seen the marine geological records of ocean and climate extended to higher and higher resolution. These studies demonstrated that century-scale changes are recorded in high-deposition-rate cores and that they correlate to high-resolution records from ice cores during the last glacial period [5]. However, the study of the present interglacial epoch in the ocean is made difficult because the upper centimetres of sediment are poorly compacted and are therefore badly preserved during coring. Consequently, high-resolution study of the Holocene requires both special coring techniques and selection of areas of fast and high sedimentary accumulation. In this paper, we shall review the major changes in sea-surface temperature (SST), thermohaline circulation and the hydrological cycle, which have been reconstructed using marine cores with accumulation rates high enough to preserve a detailed record of Holocene climatic variations and variability.
2 Sea-surface temperature variations
Long-term trends in North Atlantic SSTs have been explored recently [25,30]. However, the statistical error on SST estimates generated by faunal analysis is about 1–2 °C. As a consequence, only major SST trends can be detected by this method. By contrast, temperature reconstructions based on downcore variations in the concentration of alkenones indicate a small long-term cooling during the last 10 kyr north of 30°N opposed to a minor warming trend at low latitudes. This pattern of variability may be explained as a simple linear response of SST to the mean annual insolation, which decreases at high latitudes and increases at low latitude during the Holocene (Fig. 1). This interpretation is supported by a comparison with the SST trend observed during the previous interglacial (the Eemian, about 130–120 kyr ago), which shows a decreasing trend at high latitude and an increasing trend south of 40°N, in close correlation with the mean annual insolation variation pattern [8,9] as well as in model experiments [10]. Rimbu et al. [30] also showed that the western Mediterranean Sea exhibits a negative SST trend during the last 10 kyr, whereas the eastern Mediterranean Sea and the Red Sea show a positive trend, similar to that of the low-latitude North Atlantic Ocean. With reference to the analysis of modern observational and historical data, these authors interpret this behaviour as a continuous weakening of the North Atlantic Oscillation (NAO) pattern from the Early to the Late Holocene. Using atmospheric general circulation model simulations, which are solely forced by insolation and CO2, they suggest that the weakening of the NAO may be attributed to tropical warming during winter due to the increase in solar radiation during the Holocene in response to the Earth's precession cycle. These results illustrate the major role of the insolation forcing to explain the small variability of SST during interglacial periods.
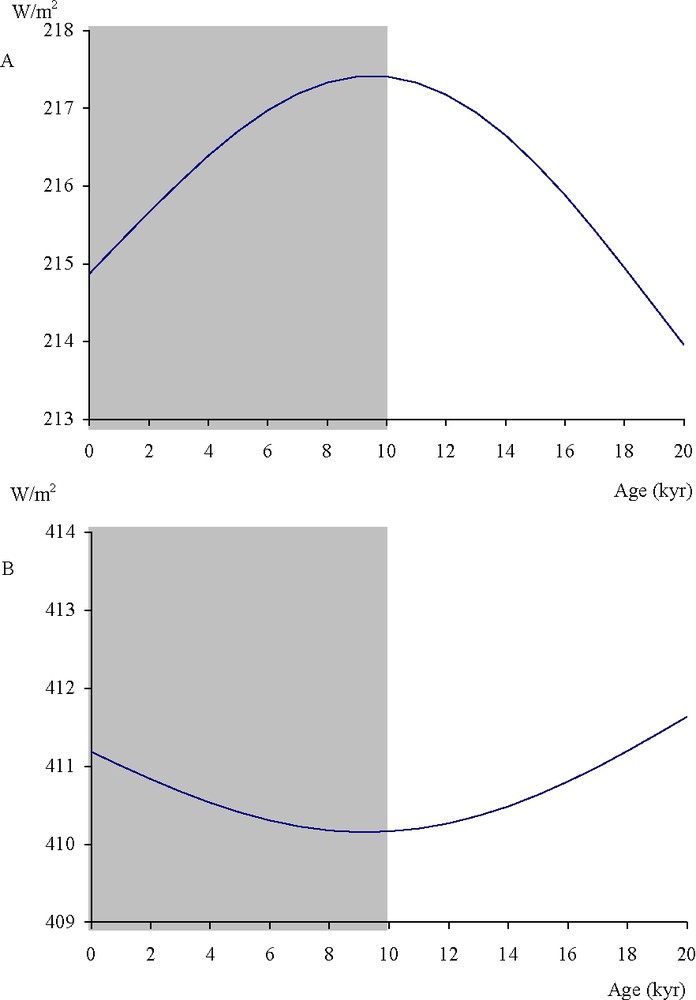
Mean annual insolation at 65°N (A) and 10°N (B) from 0 to 20 kyr BP [2]. The dashed zone indicates the Holocene.
Variations de l'insolation moyenne annuelle à 65°N (A) et à 10°N (B) entre 0 et 20 ka BP [2]. La zone hachurée indique l'Holocène.
3 Hydrological-cycle variations
Amplification of the northern hemisphere seasonal cycle of insolation during the mid-Holocene caused a northward shift of the main regions of monsoon precipitation over India and Africa. Enhanced continental rainfall in these areas resulted in enhanced outflow of both the Himalayan rivers in the Bay of Bengal and the Niger River. The outflow of the rivers draining the Himalayan Mountains increased at the end of the deglaciation, about 11.5 kyr ago, and it was larger than today during the Lower and Mid-Holocene. Both the oxygen isotopic composition and salinity of surface water in the Bay of Bengal were lower than today. This resulted in a broad negative
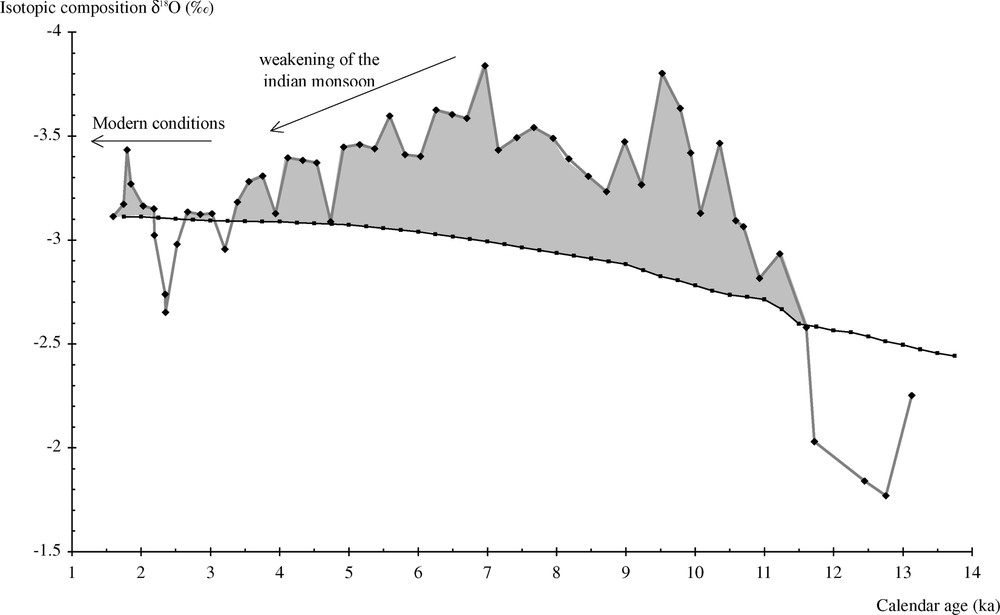
Oxygen isotopic composition (
Variations de la composition isotopique de l'oxygène (
Variations de la composition isotopique de l'oxygène (

Variations de la composition isotopique de l'oxygène (
Variations de la composition isotopique de l'oxygène (
4 Oceanic-circulation variations
The role of the ocean as a regulator of the climate system is well known, but its potential role in destabilizing climate has only recently been discovered. The thermohaline circulation acts as a pump, which transfers heat (about
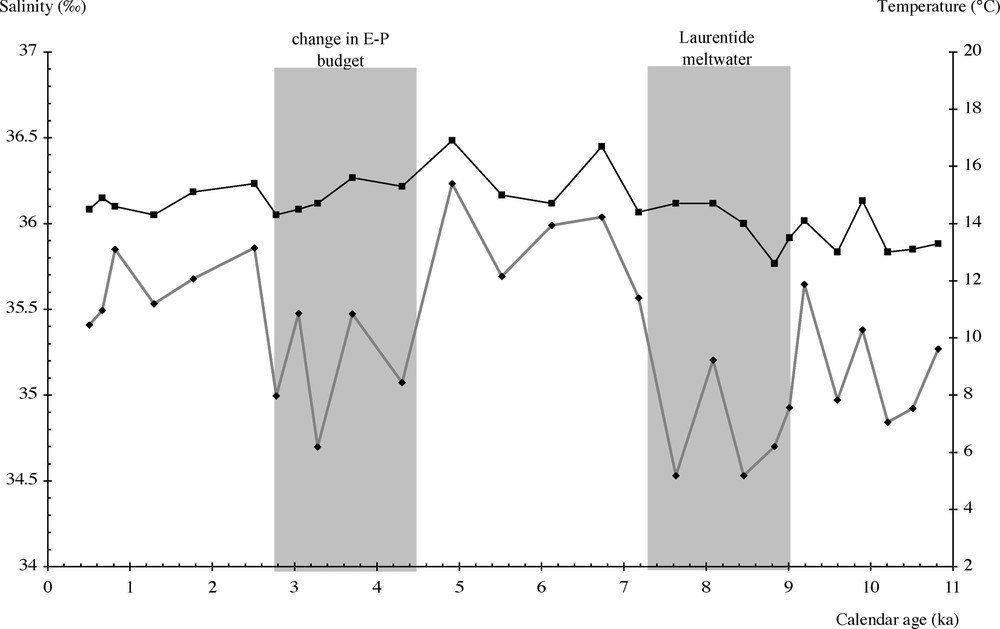
Sea-surface temperature (black squares) and salinity (black diamonds) reconstructions from core NA87-22 (55°28′N, 14°47′W, 2161 m) from the Rockall Plateau.
Reconstitution des variations de température (carrés noirs) et de salinité (losanges noirs) des eaux superficielles déduites de l'analyse de la carotte NA87-22 (55°28′N, 14°47′W, 2161 m) prélevée sur le plateau de Rockall.
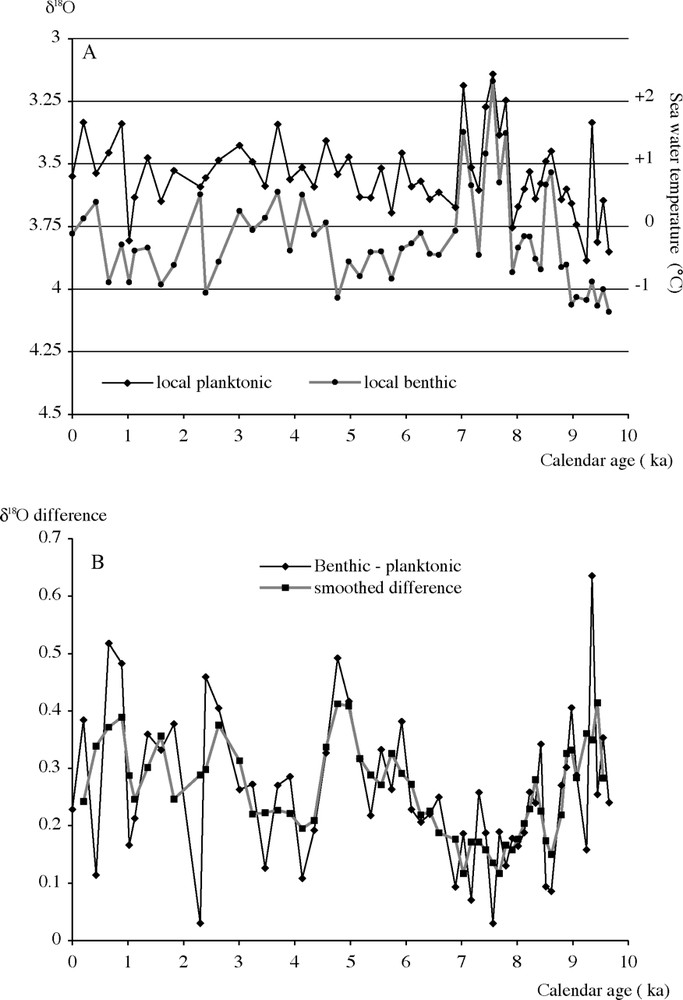
(A) Planktonic (black diamonds) and benthic (black dots)
(A) Planktonic (black diamonds) and benthic (black dots)
(A) Variations de la composition isotopique de l'oxygène (
(A) Variations de la composition isotopique de l'oxygène (
5 Conclusion and discussion
Climate changes have been less severe during the present interglacial period than during the last glaciation. Small sea-surface temperature variations