1 Introduction
Though several key advances were made during the last decades on the origin of our Solar system and on its early history, some fundamental questions remain unanswered. These questions seem to become more and more complex with the advancement of astrophysical observations of star forming regions and of the ongoing formation of extrasolar planets and with the refinement of isotopic analyses of meteorites. The study of the traces of decay of short-lived radioactive nuclides in meteorites is one major way to bring new constraints on at least three of these fundamental questions.
What is the astrophysical context for the birth of the Sun and of the Solar system? Astrophysical observations show that stars form in molecular clouds by gravitational collapse of gas and dust grains in clusters of various masses and sizes [32,61]. The evolution of the forming star and of its accretion disk is likely to depend strongly on the type of cluster in which it formed: the disk can, for instance, be partially evaporated by UV light of nearby massive stars or be truncated by the passage of the shock wave of a nearby supernova explosion. The eventual presence in meteorites of short-lived radioactive nuclides produced during such a supernova explosion may thus bring quantitative arguments for the application of these scenarios to our Solar system, other arguments based on observations in our Milky Way and other galaxies being only probabilistic.
What are the processes responsible for the formation of the first solids in the accretion disk? High temperature condensation in the nebular gas was first suggested to be responsible for the observed variations in chemical composition of primitive components in meteorites [42]. Equilibrium condensation calculations allow one to reproduce many of the first order chemical characteristics of the components of chondritic meteorites, but fail to reproduce all the details of the variations in their chemistry, suggesting that other processes such as disequilibrium vapor-liquid-solid reactions had taken place [19]. Recently, the first experimental simulations of near-equilibrium laboratory condensation, in conditions (i.e. chemical compositions, temperature and pressure of the gas) similar to those inferred for the protosolar nebula, showed the strong effects of kinetics in governing the nature and the composition of the phases condensed from the gas [90]. The isotopic anomalies, due to the in situ decay of short-lived radioactive nuclides in the various high temperature components of primitive chondrites, may help to identify phases that had a common history and to constrain their environments of formation.
What are the rate and the chronology of the processes which lead to the first solids and to the first small planets? Though the absolute age of crystallization of the Ca-Al-rich inclusions (CAIs), presumably among the earliest condensates formed in the accretion disk, is known with extreme precision (it was originally determined at
2 Short-lived radioactive nuclides in meteorites
Now extinct, short-lived radioactive nuclides were present in the protosolar nebula, as shown by the presence of isotopic excesses of their daughter isotopes in various components of meteorites. Since the discovery of the first of them, 129I (T1/2 = 16 Ma) [76], more than a dozen of them have been found in primitive meteorites (see reviews [23,25,56,68–70,77]). Among them, those having a half-life of less than a few Ma such as 7Be (T1/2 = 53 days), 10Be (T1/2 = 1.5 Ma), 26Al (T1/2 = 0.74 Ma), 36Cl (T1/2 = 0.3 Ma), 41Ca (T1/2 = 0.1 Ma), 53Mn (T1/2 = 3.7 Ma) and 60Fe(T1/2 = 1.5 Ma), are of peculiar interest.
One major discovery in the field of short-lived radioactive nuclides was, thanks to the fall of the Allende meteorite in 1969, the finding of Mg isotopic variations in it [31,43] and the demonstration that the 26Mg excess in its Ca-Al-rich refractory inclusions were positively correlated with the 27Al/24Mg ratio in a manner demonstrating that the source of the 26Mg excess was the in situ decay of short-lived 26Al [44]. Because the abundance of 26Al found in most CAIs (26Al/27Al ≈ 4.5 × 10−5) is higher by at least one order of magnitude than that predicted from continuous galactic nucleosynthesis [59,71], the presence of 26Al in the protosolar disk requires a ‘last-minute origin’. As originally pointed out by [44], two origins are possible for 26Al: either it originates from nucleosynthesis in a massive star dying close in space and time to the nascent solar system, or it was produced by local irradiation of part of the protosolar disk by high energy solar cosmic rays. This question of the origin of 26Al and of other short-lived radioactive nuclides is strongly debated and various models may explain part of the short-lived radioactive nuclides inventory either from stellar sources (e.g. [9]) or from irradiation scenario (e.g. [26]). Progress was, however, made recently from the finding of several short-lived radioactive nuclides (7Be and 10Be, 60Fe and 36Cl) to which a clear origin can be ascribed.
3 Evidence for multiple sources of short-lived radionuclides in the accretion disk
3.1 The presence of 10Be and of 7Be
The elements Li, Be and B have a peculiar nucleosynthetic origin: in addition to 7Li which is produced in significant amounts during the Big-Bang nucleosynthesis, all the isotopes of Li, Be and B are produced by spallation reactions between heavy nuclei (mostly 16O and 12C) and protons and alpha particles [75]. Because of the relatively low efficiency of this process, the cosmic abundances of Li–Be–B elements are lower by ≈5 to ≈7 orders of magnitude relative to that of their progenitors. These low concentrations in the presolar cloud and in the protosolar disk make these elements a priori very sensitive to any addition of Li–Be–B freshly nucleosynthetised by irradiation processes. The cross sections for the production of Be isotopes show that the two short-lived radioactive isotopes of Be, 10Be, (T1/2 = 1.5 Ma) and 7Be (T1/2 = 53 days), are co-produced with 9Be in significant amounts [60,74,81]. The presence in the protosolar nebula of fresh Li and B produced by irradiation processes is strongly suggested by the small scale Li and B isotopic variations found in chondrules [11,12].
The possible presence of 10Be in CAIs was thus investigated early [82] but, because of the analytical difficulties in measuring isotopic compositions of B present at very low concentrations, it is only with the last generations of ion microprobes that clear 10B excesses, positively correlated with the 9Be/11B ratios (Fig. 1a) could be found [57]. Since then, the in situ decay of 10Be has been detected in many CAIs [17,51,57,86]; in fact, in all CAIs in which the distribution of B was not perturbed by metamorphism, alteration or contamination, processes which can have strong effects for a trace element like B present at the sub-ppm level. By analogy with 26Al, a maximum 10Be/9Be ratio for CAIs of ≈1 × 10−3 can be defined, but significant variations in the 10Be/9Be ratios are present within CAIs (see review [10]). Hibonites and some rare CAIs appear to have formed with a 10Be/9Be ratio of ≈ half that of normal CAIs, which cannot a priori be interpreted in a chronological way since hibonites are more refractory than ‘normal’ CAIs and should condense first (see next section for details). Thus it is not in doubt that 10Be was present in the early solar system and that it was most likely produced by irradiation processes in the protosolar disk [26]. However, the presence or absence of 10Be in other meteoritic components, such as chondrules, has not yet been established because the anticipated 10B excesses are too small (the 9Be/11B ratio is low in chondrules) to be resolved by current ion probe techniques.
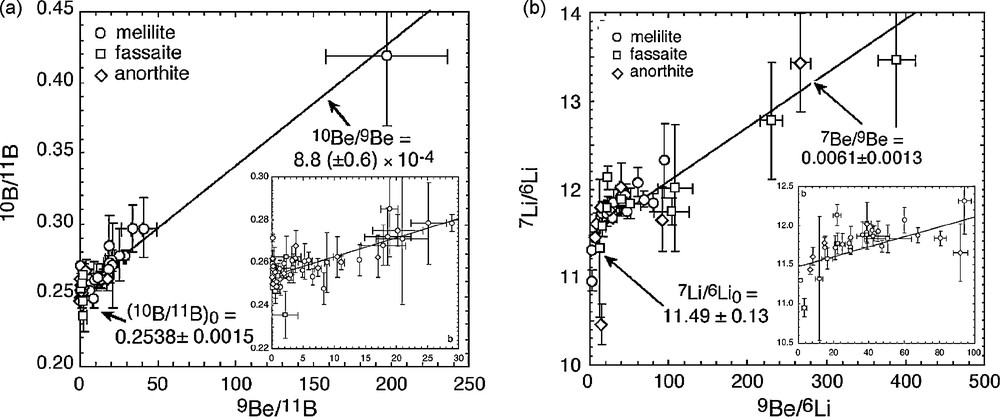
Variations of 10B/11B ratios vs. 9Be/11B ratios (Fig. 1a) and of 7Li/6Li ratios vs. 9Be/6Li ratios (Fig. 1b) in one type B CAI from the CV chondrite Allende [17]. The positive correlations observed imply that short-lived 10Be (T1/2 = 1.5 Ma) and 7Be (T1/2 = 53 days) were incorporated by the CAI at the time of its crystallization, i.e. when the fractionations responsible of the observed variations of the 9Be/11B and 9Be/6Li ratios took place.
Fig. 1. Variations des rapports 10B/11B en fonction des rapports 9Be/11B (Fig. 1a) et des rapports 7Li/6Li en fonction des rapports 9Be/6Li (Fig. 1b) dans une CAI de type B de la chondrite CV Allende [17]. Les corrélations positives observées entre ces rapports impliquent l’incorporation par cette CAI lors de sa cristallisation, c’est-à-dire au moment où les fractionnements observés pour les rapports 9Be/11B et 9Be/6Li ont été établis, des isotopes radioactifs à courte période 10Be (T1/2 = 1,5 Ma) et 7Be (T1/2 = 53 jours). Masquer
Fig. 1. Variations des rapports 10B/11B en fonction des rapports 9Be/11B (Fig. 1a) et des rapports 7Li/6Li en fonction des rapports 9Be/6Li (Fig. 1b) dans une CAI de type B de la chondrite CV Allende [17]. Les ... Lire la suite
Much effort was put for the detection of 7Be in CAIs since the discovery of 10Be [14–17]. The detection of 7Li excesses due to the decay of 7Be is, however, difficult because of: (i) the extremely low Li concentrations, in the ppb range, of non-altered CAIs; (ii) the presence of 6Li excesses theoretically associated to the presence of 7Be (Li co-produced together with 7Be and 10Be has a 7Li/6Li ≈ 2 and the chondritic 7Li/6Li ratio is of ≈12); (iii) the presence in significant amounts of spallogenic Li produced during recent exposure to galactic cosmic rays; and (iv) the fact that Li is a very mobile element from the geochemical point of view. The presence of Li produced by irradiation processes in the protosolar disk is obvious from the 7Li/6Li ratio of CAIs, which is generally lower than the chondritic one: the two lowest 7Li/6Li ratios found are of 9.20 ± 0.22 in Allende CAI 3529-41 [17] and of 9.9 ± 1.1 in Axtell CAI 2771 [51]. The presence of 7Li excesses due to the in situ decay of 7Be seems quite clear in the case of Allende CAI 3529-41 (Fig. 1b) and the correlation observed between the 7Li/6Li and the 9Be/6Li ratios would imply a 7Be/9Be ratio of ≈6.1 ± 1.3 × 10−3 at the time of formation of this CAI [17]. Models of irradiation processes around the early active Sun can reproduce the observed 10Be/9Be and 7Be/9Be ratios, but because of the very short half-life of 7Be, the observed 7Be abundance would require short irradiation times of one to a few years [26,30,46].
3.2 The presence of 36Cl
The possible presence of short-lived 36Cl (T1/2 = 0.3 Ma) was first inferred from 36Ar excesses in fine-grained silicates from the carbonaceous chondrite Efremovka [66]. However, because 36Ar in meteorites can have several other sources than the in situ decay of 36Cl, e.g. trapped and cosmogenic components, an estimate of the 36Cl/35Cl ratio of 1.4 ± 0.2 × 10−6 could only be proposed, since no parent-daughter correlation could be established. Because 36Cl has a branched decay (98.1% to 36Ar and 1.9% to 36S), its presence should theoretically be better proved by looking for 36S excesses. Very clear 36S excesses positively correlated with the Cl/S ratios which were reported recently for secondary halogen-rich phases in CAIs (Pink Angel CAI from Allende) and chondrules [34,49]. These excesses cannot result from a late cosmic-ray production. The best estimate of the 36Cl/35Cl at the time of formation of these Cl-rich phases (e. g. sodalite grains) is ≈4 × 10−6. The origin of 36Cl in the Pink Angel CAI is strongly constrained by the fact that it contains no 26Al (upper limit of 1.7 × 10−6 for 26Al/27Al, [34]). This decoupling can be explained by either (i) a mechanism that produces 36Cl and no 26Al or (ii) an isotopic closure of the 36Cl/36S system when 26Al was extinct. In this latter hypothesis, assuming that 26Al and 36Cl are stellar products initially homogenized in the protosolar nebula, then the initial 36Cl/35Cl ratio should have been of ≈2 × 10−2 when 26Al/27Al = 5.85 × 10−5. Because stellar nucleosynthetic sources, either SN or AGB, co-produce 26Al and 36Cl but at a level of ≈10−6 (scaled assuming all 26Al in the protosolar nebula is a stellar product), the observed 36Cl in Pink Angel cannot be a stellar product [34]. Conversely, 36Cl is likely to be an irradiation product which was produced within the solar system: the production in sufficient amounts of 36Cl alone can be shown theoretically in various scenario, either close to the active early Sun or in the meteorite forming region, but its co-production with 10Be (and possibly 26Al) would require a production close to the Sun [24,26,29,30,46]. The presence of 36Cl in these alteration phases formed late is consistent with the fact that young stellar objects of masses similar to that of the Sun are observed to have a high X-ray luminosity for up to several Ma [21].
3.3 The presence of 60Fe
Among the various short-lived radioactive nuclides, 60Fe (T1/2 = 1.49 Ma) is the only one which is indubitably a stellar product because the abundances in the protosolar nebula of the projectiles and targets required to produce this neutron-rich isotope are too low [45]. Early studies of the Ni isotopic composition of CAIs [3] and eucrites [80] proved the presence of some radiogenic 60Ni excesses which, however, turned out difficult to use to determine the amount of 60Fe present in the early solar system because of the presence of nucleosynthetic Ni isotopic anomalies. An upper limit of 1.6 ± 0.5 × 10−6 was proposed from CAIs [3]. Clear evidence for 60Fe was reported recently from ion microprobe analyses of troilite and magnetite in the ordinary chondrites Bishunpur, Krymka and Semarkona [63,64,87]. The highest 60Fe/56Fe ratio found is of 0.92 ± 0.24 × 10−6 for troilite from Semarkona [64]. A somewhat lower 60Fe/56Fe ratio of 2.2 × 10−7 to 3.7 × 10−7 has been found in ferromagnesian chondrules from ordinary chondrites [88]. The latest high precision MC-ICPMS data add more complexity to the question of the distribution of 60Fe in the early solar system. A re-investigation of the Ni isotopic composition of CAIs has shown that in many CAIs, the nucleosynthetic anomalies on 62Ni are correlated with that of 60Ni, but that, in CAIs with no nucleosynthetic 62Ni anomaly, there are still 60Ni excesses which are correlated with the Fe/Ni ratios and would indicate a 60Fe/56Fe of 4.7 ± 2.9 × 10−6 at the time of formation of CAIs [73]. However, [6] have found a deficit in 60Ni (of ≈0.024‰) in iron meteorites, pallasites, ureilites and one angrite relative to the Earth, Mars and chondrites which they interpret as reflecting a late injection of 60Fe in the protosolar disk, approximately 1 Ma after the formation of CAIs (which thus should not contain any radiogenic 60Ni excess). There is no clear agreement at present on the Ni isotopic composition of iron meteorites [6,72]. Thus, though the presence in the protosolar nebula of 60Fe cannot be disputed, its initial abundance and its distribution are the matter of ongoing work.
4 Homogeneous or heterogeneous distribution of short-lived radionuclides in the accretion disk?
One obvious prerequisite for the use of short-lived radioactive nuclides as precise chronometers is that they were once homogeneously distributed in the accretion disk, so that time differences can be calculated for different objects relative to the initial abundance at a time ‘0’. Different chronologies can, however, be worked out in the hypothesis of an heterogeneous distribution [27]. Intuitively it seems logical to conceive an homogeneous protosolar nebula if all materials were once vaporized in the gas phase. However, this was not obligatory in the case.
4.1 Theoretical considerations
In fact, it is quite impossible at present to demonstrate definitely that short-lived radioactive nuclides were homogeneously distributed in the protosolar nebula. Theoretical considerations indicate that this could be the case whatever the source of the short-lived nuclides. In the case of an injection of these nuclides, either in the presolar cloud or onto the surface of the protosolar nebula, nebular mixing and transport models show that an homogeneity of ≈10% relative can be reached on very short timescales of a few thousand years [7]. In the case of an irradiation scenario, in which short-lived nuclides are produced during the irradiation of the nebular dust by energetic particles from the active early Sun [29,30], it is conceivable to reach a more or less constant abundance of short-lived nuclides in the irradiated dust for typical residence times in the reconnection ring [45]. However, in this case the production of short-lived nuclides is restricted to the dust which enters the reconnection ring and an homogenization at the scale of the disk can only occur when the dust is launched to asteroïdal distances and thus ‘diluted’ into the accretion disk. The recent finding of CAI-like material in some cometary grains sampled by the STARDUST NASA mission [58,96] suggests that such a vigorous mixing existed in the early times of the disk.
4.2 Observational constraints in the case of 26Al
26Al is the only short-lived nuclide for which: (i) the data base is large enough (numerous in situ ion probe studies of CAIs mostly and of chondrules); and (ii) the precision of the latest bulk analyses is high enough (thanks to the developments of MC-ICPMS techniques), to try to test its homogeneity in the accretion disk.
The existence of a mode in the distribution of the initial 26Al/27Al ratios in CAIs at around 4.5 × 10−5, the so-called ‘canonic value’, [50] has long been taken as a strong argument in favour of an homogeneous distribution of 26Al, at least in the high temperature regions of the disk where CAIs and their precursors were formed. Many CAIs having 26Al/27Al ratios lower or higher than canonic can be demonstrated to have undergone secondary perturbations of the Al/Mg system. However, this is not case for all of them: CAIs from CBb chondrites formed with 26Al/27Al < 4.6 × 10−6 [28]. In addition, some hibonite crystals [20,35,79] and most grossite-bearing CAIs from the chondrite Acfer 182 [92] contain no resolvable radiogenic 26Mg excess (the inferred 26Al/27Al are < 5 × 10−6). This is best interpreted as reflecting condensation in a part of the protosolar nebula devoid of 26Al. It has been proposed that some of these samples could reflect a very early formation in the protosolar nebula prior to the arrival of 26Al injected from a nearby stellar source [70]. A recent high precision study of two hibonite-rich CAIs from Acfer 182 [83] has shown that in fact the radiogenic 26Mg excesses can be resolved, implying for these two CAIs 26Al/27Al have ratios of 4.5 ± 3.6 × 10−6 and of 1.11 ± 0.49 × 10−5, respectively. These two CAIs also contain radiogenic 10B excesses implying, in agreement with previous studies [53], a 10Be/9Be of 6.9 ± 1.6 × 10−4 at the time of their formation [83]. The difference in 10Be/9Be and 26Al/27Al ratios between the Acfer CAIs and ‘normal’ CAIs seems difficult to interpret in chronological terms assuming an homogeneous distribution of these short-lived nuclides in the early solar system (Fig. 2). Work is in progress to try to refine the 10Be and 26Al measurements in these CAIs. Because 10Be is not a stellar product, the 26Al present in the Acfer CAIs (at a lower level than in ‘normal’ CAIs) may be of irradiation origin. In the irradiation scenario, it has been noted that exposure of protoCAIs to gradual but not impulsive events could produce 10Be with little 26Al [30].
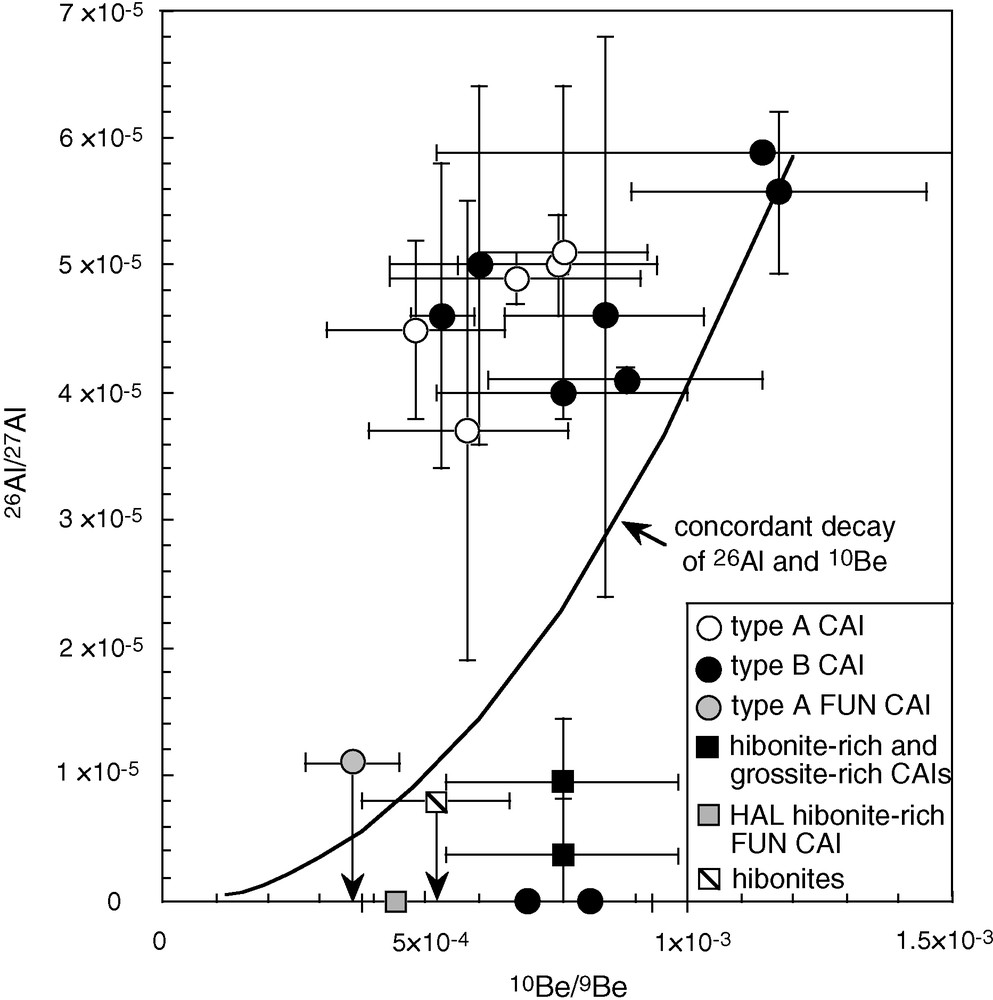
Variations of 26Al/27Al versus 10Be/9Be ratios in various refractory inclusions from chondrites, data from [17,20,51,53,57,79,83,86,92]. An arrow indicates that only an upper limit can be inferred for 26Al/27Al. The 10Be/9Be ratio for hibonites is inferred from an isochron grouping data on three grains combined together [53]. The hibonite-rich FUN CAI named HAL has a low but well-resolved 26Al/27Al ratio of 5.2 ± 1.7 × 10−8 [20] similarly to the grossite-rich CAIs [83,92]. The range in 26Al/27Al ratios within type B CAI is most likely due to secondary perturbations on the Al/Mg system [93]. Masquer
Variations of 26Al/27Al versus 10Be/9Be ratios in various refractory inclusions from chondrites, data from [17,20,51,53,57,79,83,86,92]. An arrow indicates that only an upper limit can be inferred for 26Al/27Al. The 10Be/9Be ratio for hibonites is inferred from an isochron grouping ... Lire la suite
Fig. 2. Variations des rapports 26Al/27Al en fonction des rapports 10Be/9Be dans différentes inclusions réfractaires de chondrites, données provenant de [17,20,51,53,57,79,83,86,92]. Une flèche indique que seule une limite supérieure a pu être déterminée pour le rapport 26Al/27Al. Le rapport 10Be/9Be des hibonites est déduit d’une isochrone déterminée à partir de trois grains différents [53]. La CAI FUN riche en hibonite appelée HAL a un rapport 26Al/27Al bas, mais bien résolu de 5.2 ± 1.7 × 10−8 [20], de même que les CAIs riches en grossite [83,92]. La gamme de variation des rapports 26Al/27Al à l’intérieur des CAIs de type B reflète très probablement des perturbations secondaires du système Al/Mg [93]. Masquer
Fig. 2. Variations des rapports 26Al/27Al en fonction des rapports 10Be/9Be dans différentes inclusions réfractaires de chondrites, données provenant de [17,20,51,53,57,79,83,86,92]. Une flèche indique que seule une limite supérieure a pu être déterminée pour le rapport 26Al/27Al. Le rapport ... Lire la suite
The significance of the so-called ‘canonic’ 26Al/27Al ratio of 4.5 × 10−5 has recently been challenged from high precision laser ablation MC-ICPMS measurements [93] which showed that some CAIs had initial 26Al/27Al ratios as high as 7.0 ± 1.3 × 10−5 or 6.3 ± 1.3 × 10−5, depending on the way one corrected for Mg isotopic mass fractionation. The ‘canonic value’ of 4.5 × 10−5 for the 26Al/27Al ratio can then be viewed as reflecting the end of the high temperature history of CAIs which could have undergone during up to ≈300 000 years (i.e. from 6.0 × 10−5 to 4.5 × 10−5) multiple reheating events as single solid particles transported towards and outwards the Sun in the protosolar nebula.
The latest high precision bulk Mg isotope analyses of CAIs from CV chondrites [4,89] define a perfect isochron, implying that all the CAIs analysed had the same 26Al/27Al ratio of 5.85 ± 0.05 × 10−5 and initial Mg isotopic composition (δ26Mg*) of -0.031 ± 0.0038‰ at the time of their formation. Because these data are bulk analyses, there are a priori not affected by secondary perturbation of the Al/Mg system as long as these perturbations occur in a closed system within the CAI. These remarkable data offer, for the first time, the possibility to test the homogeneity of the distribution of 26Al in the protosolar nebula. This can be done by comparing the Mg isotopic composition of the Earth (which was likely assembled from planetesimals formed over several A.U. from the Sun, see [62]) to that predicted from the Mg isotope evolution curve of the protosolar nebula anchored with CAIs (which condensed in the high temperature inner region of the nebula). This is shown in Fig. 3. As noted by Thrane et al. [89], the Earth does not fall on this evolution curve: assuming an homogeneous protosolar nebula whose Mg and Al isotopic compositions are identical to that defined by the CAIs isochron, a δ26Mg* of +0.0107 ± 0.0055 can be calculated for the solar nebula when 26Al is extinct. This value is significantly different from the δ26Mg* of −0.0002 ± 0.0022‰ measured for terrestrial rocks [89]. This apparent inconsistency implies that the Mg and Al isotope compositions of CAIs are different from that of the material that formed the Earth. In order to reconcile this observation with the hypothesis of an homogeneous protosolar nebula, it is necessary to postulate that CAIs, or their solid precursors, underwent a short period of closed system evolution, before they achieved isotopic closure for Mg. For a 27Al/24Mg ratio of ≈2.9 for CAIs [50], it can be calculated that after ≈9000 ± 2000 years in closed system, with initial compositions for the protosolar nebula (δ26Mg* = −0.0421 and 26Al/27Al = 5.9 ± 0.01 × 10−5), the CAIs would get their observed Mg and Al isotopic composition, while the Earth would get a δ26Mg* ≈ 0 (Fig. 3). This scenario, to work quantitatively, implies, however, that all CAIs: (i) had a very short closed system history; and (ii) that the duration of this closed system evolution was strictly inversely proportional to their (or that of their precursors) 27Al/24Mg ratio (for instance with a 27Al/24Mg ratio of 1 the duration should be of ≈27 000 years). Though this hypothesis cannot be ruled out, this fine tuning seems, however, a quite stringent requirement on the formation of CAIs and it cannot be easily reconciled with variations observed within CAIs [93], suggesting that CAIs experienced a longer closed system high temperature history. Obviously, a much simpler way to reconcile the δ26Mg* of the Earth with the CAIs composition, would be to admit the existence of some heterogeneities in the distribution of 26Al in the protosolar nebula. The δ26Mg*of the Earth would require for instance that its source material had once a 26Al/27Al ratio of ≈4.5 × 10−5 for a δ26Mg* of −0.031‰ (Fig. 3), which would correspond to a ≈25% variation in the abundance of 26Al. Such variations seem quite logical if several sources exist for 26Al, irradiation by the young active Sun producing 26Al in the inner region of the protosolar nebula only. Alternatively, heterogeneities of the Mg isotopic composition could also be present in the solar nebula.
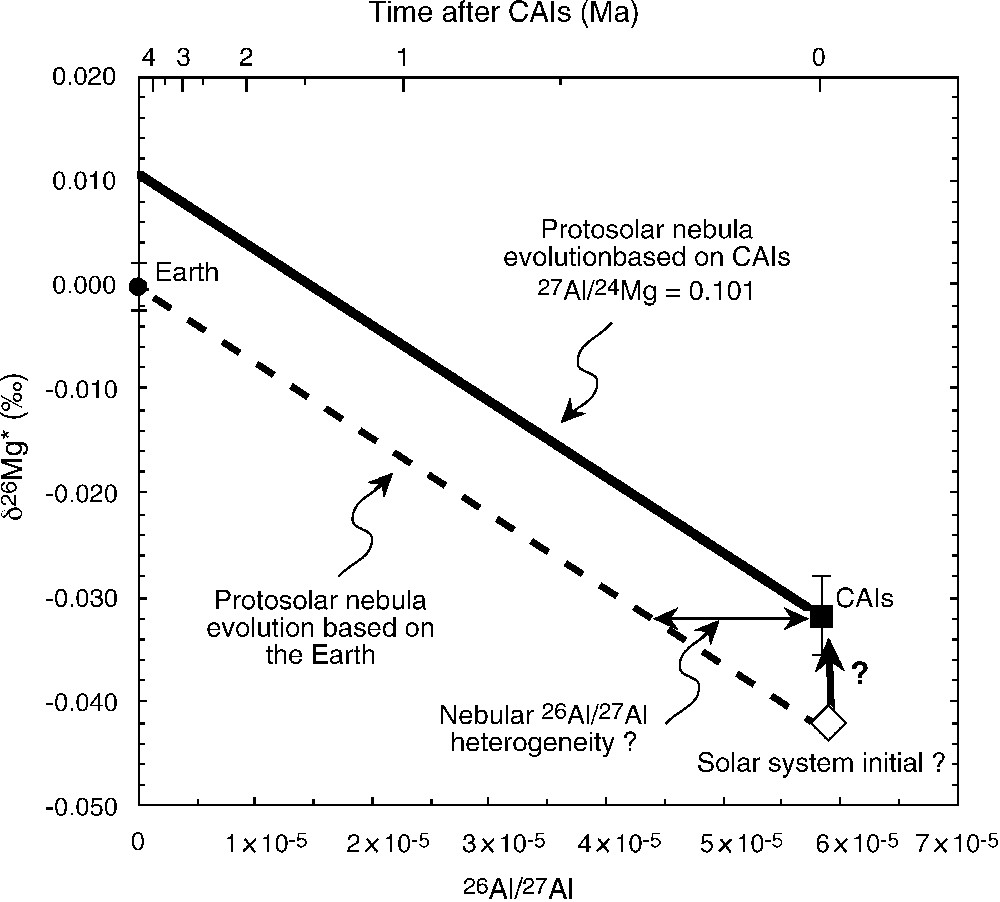
Evolution of the Mg isotopic composition of the solar nebula calculated from the 26Al/27Al ratio and the initial δ26Mg* value determined from the bulk CAIs isochron measured by [89]. The composition of the Earth falls significantly off the evolution line, implying either that the solar nebula was not homogeneous for the Al and/or the Mg isotopic compositions or that CAIs underwent a short closed system evolution of their Mg isotopic composition before being quenched (see text).
Fig. 3. Évolution de la composition isotopique du Mg dans la nébuleuse solaire, calculée à partir du rapport 26Al/27Al et du δ26Mg* initial déterminés à partir de l’isochrone pour les CAIs en roche totale, mesurée par [89]. La composition de la Terre se situe significativement en dehors de la droite d’évolution, ce qui implique, soit que le système solaire n’ait pas été homogène pour ses compositions isotopiques d’Al et/ou de Mg, soit que les CAIs aient subi une évolution très courte, en système fermé, de leurs compositions isotopiques de Mg, avant d’être refroidies (voir texte). Masquer
Fig. 3. Évolution de la composition isotopique du Mg dans la nébuleuse solaire, calculée à partir du rapport 26Al/27Al et du δ26Mg* initial déterminés à partir de l’isochrone pour les CAIs en roche totale, mesurée par [89]. La ... Lire la suite
5 Nebular time scales: from CAIS to chondrules and chondrites
As detailed above, CAIs are the oldest rocks known in the solar system with an age of 4568.5 ± 0.5 Ma [8] and they have the highest 26Al/27Al ratios found in chondrites. The very small scatter observed for the bulk CAI isochron, all CAIs have the same initial δ26Mg* within ±0.0038‰ [89], implies that the nebular high temperature history of CAIs, was extremely short, less than ≈20 000 years. If CAIs would have remained above the closure temperature for Mg isotopes diffusion for longer periods they would have developed significant variations in their δ26Mg*: for a 50 000 year closed system evolution, the δ26Mg* would be increased by 0.019‰, 0.038‰ and 0.058‰, for 27Al/24Mg ratios of 1, 2 and 3 respectively. If the high temperature nebular history of CAIs is so short, then the meaning of the average, or ‘canonic’ 26Al/27Al ratio of CAIs of 4.5 × 10−5 is rather difficult to explain, unless it is mostly due to secondary perturbations. Alternatively, one has to consider a longer residence time in the nebula (≈100 000 years) which could explain the supracanonic and the range of 26Al/27Al ratios found in CAIs if they underwent, during this period, numerous high temperature events [93]. These two views seem quite contradictory at present.
Chondrules, which are the major high-temperature constituent of chondrites, have mineralogical, textural and chemical characteristics which imply that they have formed during brief and incomplete melting events of solid precursors [33,36,47,94] and that they have undergone strong gas-melt interactions in the protosolar nebula [22,48]. It leaves no doubt, from recent lead isotopic dating, that chondrules are old and that their formation occurred over several millions years. Chondrules in the CR-chondrite Acfer have a Pb/Pb age of 4564.7 ± 0.6 Ma [1], i.e. postdating CAIs by ≈3.8 Ma. Chondrules in two CB Bencubbin-like chondrites have younger ages of 4562.7 ± 0.9 Ma [41], i.e. ≈5.8 Ma after CAIs. Some chondrules might have formed during nebular shock waves [18], while others might have formed in vapour-melt plumes produced during energetic collision between planetary embryos [41]. The timescales given by the Pb/Pb ages are in quite good agreement with what can be inferred from the 26Al systematics. Ion microprobe analysis has allowed to identify radiogenic 26Mg excesses in many chondrules having favourable 27Al/24Mg ratios [37,65,78]: the 26Al/27Al inferred at the time of crystallization of chondrules range from ≈0.4 ± 0.2 × 10−5 to ≈2.3 ± 0.7 × 10−5, which would correspond to an age difference from ≈1 Ma to ≈3 Ma relative to CAIs. However, as emphasized by [78], there are many chondrules which do not show any significant radiogenic 26Mg excess and any sign of metamorphic perturbations: these chondrules might be younger or have formed in regions of the nebular devoid of 26Al. On the other hand, high precision bulk Mg isotopic analyses of chondrules [4,22] show that some of them might have formed nearly contemporaneously with CAIs. The interpretation of the bulk 26Mg excesses of chondrules is complicated by the fact that the bulk 27Al/24Mg of chondrules reflects that of their precursors material and thus may ‘date’ the age of the precursors and not that of the melting event which produced the mineralogy typical of chondrule. This is further complicated by the fact that, most likely, Mg-rich chondrules contain relict olivines which did not re-equilibrated with the chondrule melt, as shown by oxygen isotope analyses [13]. No internal 26Al isochron exists yet that demonstrates chondrule formation contemporaneously with CAIs, and it is a major goal of future studies to refine the 26Al systematics of chondrules to better constrain their origins.
Obviously, chondrite accretion cannot predate formation of chondrules, though some chondrules can be made during collisions between chondritic parent bodies as suggested by the recent young ages of chondrules from two CB chondrites (see above). There is growing evidence (see the next section) that planetesimals or planetary embryos accreted and differentiated very early in the accretion disk, more or less contemporaneously with the formation of CAIs, and thus earlier than chondrites accreted. Dating the accretion of chondrites parent bodies is quite difficult because the accretion in itself does not produce strong chemical fractionations. One approach is to date early metamorphic phases produced during the cooling following the accretion. Plagioclase crystals of presumably metamorphic origin from two H4 chondrites show radiogenic 26Mg excesses corresponding to 26Al/27Al ratios of ≈1.5 ± 0.5 × 10−7 to ≈2.8 ± 0.6 × 10−7 [95], which would indicate metamorphism on this parent body from 5.6 to 6.3 Ma after the formation of CAIs.
6 Short-lived nuclides constraints on early accretion and differentiation of planetesimals
Though recent models of runaway growth show that planetesimals and planetary embryos can form early in the accretion disk (in a few 104 to less than 106 years, [40,62]), there was, until very recently, very little isotopic data from meteorites allowing one to constrain these timescales. However, as pointed in the 1950s [91], because short-lived 26Al or 60Fe are potential heat sources for the melting of early accreted bodies, traces of these nuclides should be present in early differentiated meteorites. One of the first evidence for an early differentiation of some meteoritic parent bodies was the finding of 26Mg excesses in plagioclase crystals from the eucrite Piplia Kalan [84]. A 26Al/27Al ratio of ≈7.5 ± 0.9 × 10−7 was inferred from these data at the time of the crystallization of these plagioclases from melts produced during the melting and differentiation of the eucrite parent body, maybe the asteroid Vesta. This 26Al/27Al ratio would imply that this differentiation occurred ≈4.6 Ma after the crystallisation of CAIs (taking 26Al/27Al ≈5.85 × 10−5 for CAIs). A quite similar 26Al/27Al ratio (1.18 ± 0.14 × 10−6) was found for another eucrite, Asuka 881394 [67]. High-precision bulk Mg isotope analyses of samples from eucrites, mesosiderites and angrites [2,5] show the presence of 26Mg excesses, which imply that the 26Al/27Al was ranging from ≈2 × 10−6 and ≈5 × 10−6 when magmatic activity was taking place within these bodies. This corresponds to a time difference from ≈2.5 Ma to ≈3.5 Ma after CAIs. Numerical simulations show that, in order to melt at large scale (>50% melting) a small body by the heat generated from the radioactive decay of 26Al (T1/2 = 0.73 Ma) and 60Fe (T1/2 = 1.5 Ma), heat has to be accumulated for a rather long period of time: for the observed 26Al and 60Fe abundances accretion of the eucrite or angrite parent bodies should occur very early (≈0.7 Ma after CAIs), in order to have global melting of these bodies at ≈3 Ma [5].
Because reducing conditions are prevalent at high temperature, early melting of planetesimals must have resulted in metal–silicate differentiation. The 182Hf–182W system (182Hf decays to 182W with a half life of 8.9 Ma) offers a way to trace and to date this differentiation. The radiogenic 182W excesses measured in CAIs and carbonaceous chondrites [91] allow one to determine that, at the time of formation of CAIs, the 182Hf/180Hf ratio in the protosolar nebula was 1.07 ± 0.10 × 10−4 and that the W isotopic composition was depleted in 182W by ≈0.35‰ (ɛW = –3.47 ± 0.20) relative to the Earth. Magmatic iron meteorites have W isotopic composition that, after correction for burnout of W during exposure to galactic cosmic rays, is indistinguishable from that of CAIs [39,54]. In order to keep this non-radiogenic W isotopic composition, metal-silicate differentiation must have occurred on the parent body of magmatic irons no later than ≈1 Ma after CAIs [54]. This again implies the accretion of small planetary bodies very early in the protosolar disk. Recent 182Hf–182W studies of angrites, eucrites and eucrite zircons show, similarly to 26Al studies, that some of the angrites and of the eucrites are the products of igneous crystallization on their parent body ≈5 Ma after CAIs [38,55,85].
If planetesimals formed very early, during the first 0.1 to 1 Ma, it can be anticipated that most of them encountered high collision rates during the runaway growth period [62]. Because chondrules seem, in average, to have ages younger than CAIs by up to a few Ma, their precursors might have comprised fragments of the early accreted and differentiated planetesimals. The evidence for the presence of such fragments was found very recently by the study of the mineralogy and petrography of magnesian porphyritic (Type I) chondrules from the CV carbonaceous chondrite Vigarano [47]. It has been shown that these chondrules contain olivine aggregates which have relict textures (Fig. 4) and are most likely explained as being small fragments of olivine-rich differentiated planetesimals. Oxygen isotope studies [13] strongly support the idea that these type I chondrules formed by reactions between the relict olivine aggregates and the nebular gas.
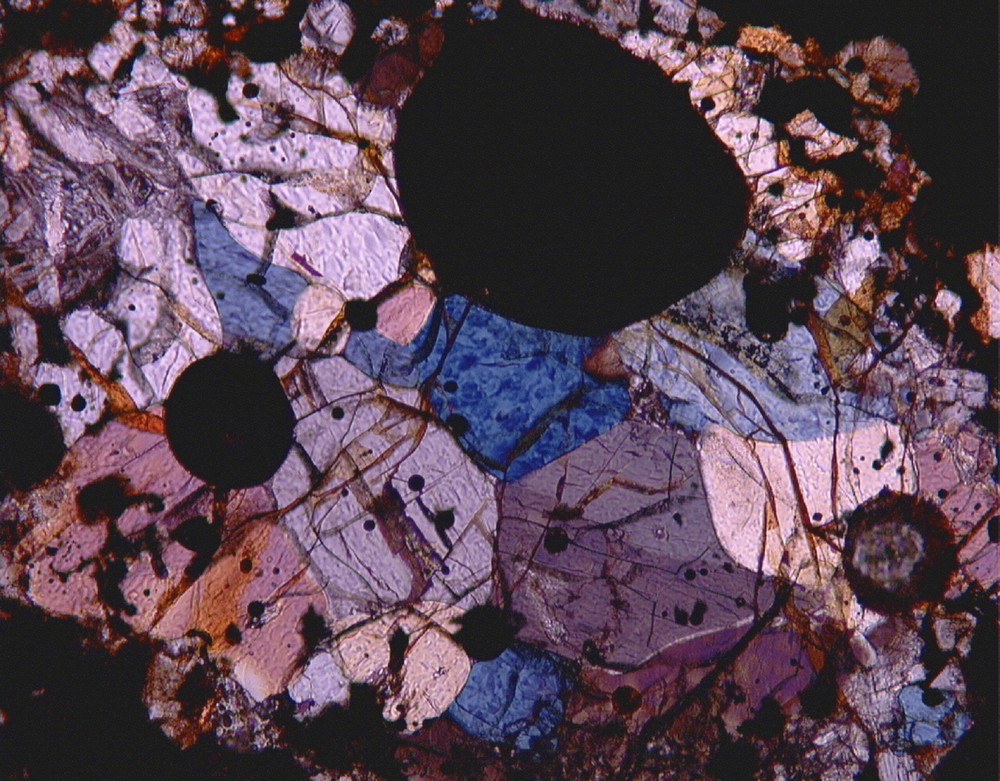
Optical image in crossed-polarized light of a magnesian type I porphyritic chondrule from the CV carbonaceous chondrite Vigarano. The chondrule contains coarse-grained aggregates composed of Fe, Ni-metal and magnesian olivines surrounded by an igneous shell composed of low-Ca pyroxene and glassy mesostasis. Some olivine–olivine grain boundaries in the aggregate lack any layer of glassy mesostasis and show triple junctions with granoblastic equilibrium textures [47]. These textures imply that the olivine are relict, in agreement with the fact that they have a different oxygen isotopic composition from the glassy mesostasis [13]. These coarse-grained aggregates are likely to be fragments of planetesimals that formed early, before chondrules. Masquer
Optical image in crossed-polarized light of a magnesian type I porphyritic chondrule from the CV carbonaceous chondrite Vigarano. The chondrule contains coarse-grained aggregates composed of Fe, Ni-metal and magnesian olivines surrounded by an igneous shell composed of low-Ca pyroxene and ... Lire la suite
Fig. 4. Image en lumière polarisée analysée d’un chondre magnésien porphyrique de type I de la chondrite carbonée Vigarano. Le chondre contient des agrégats à gros grains composés de métal riche en Fe et Ni et d’olivines magnésiennes entourées d’une couche de pyroxène pauvre en Ca et de verre. Certains des joints de grain (olivine–olivine) sont secs, sans verre, et montrent des jonctions triples caractéristiques de textures équilibrées de type granoblastique [47]. Ces textures impliquent que les olivines soient reliques, comme l’indique aussi le fait qu’elles aient des compositions isotopiques de l’oxygène, différentes de celle de la matrice vitreuse [13]. Ces agrégats à gros grain sont très probablement des fragments de planétésimaux qui se sont formés très tôt dans le disque, avant la formation des chondres. Masquer
Fig. 4. Image en lumière polarisée analysée d’un chondre magnésien porphyrique de type I de la chondrite carbonée Vigarano. Le chondre contient des agrégats à gros grains composés de métal riche en Fe et Ni et d’olivines magnésiennes entourées d’une ... Lire la suite
7 Conclusions
The presence of the traces of in situ decay of short-lived radioactive nuclides in various components of primitive chondrites and of differentiated meteorites demonstrates that, within a few million years at most, grains were condensed in the accretion disk and that planetesimals were accreted and differentiated. Very precise chronologies are being established for all these processes from the observed variations in the abundances of the various short-lived radioactive nuclides. However, it is important to stress that these chronologies rely on several assumptions which constitute some fundamental open questions:
- - what is the nucleosynthetic origin of the various short-lived radioactive nuclides: external seeding of the accretion disk from a nearby dying massive star and/or localized irradiation by the young active Sun?
- - what was the distribution of the various short-lived radioactive nuclides in the accretion disk, homogeneous, heterogeneous (at what temporal and spatial scale)?
- - to what extent were the observed short-lived radioactive nuclides abundances modified by secondary (nebular and/or parent body) processes?