1 Introduction
Irradiation encompasses a large diversity of interaction modes of accelerated particles with solid or gaseous matter. Depending on the energy of the accelerated particles, irradiation can lead to structural damage (e.g., [80]), or to the production of new isotopes via nuclear reactions (e.g., [19]). Here we will restrict ourselves to nuclear effects, i.e. to the nucleosynthesis production of new isotopes due to the interaction of matter with MeV cosmic rays, and more specifically on the nucleosynthesis of short-lived radioisotopes (SRs) in the early solar system. Other effects of irradiation are discussed in a variety of recent reviews [5,7].
Short-lived radioisotopes (SRs) are radioactive elements with half-lives of
Initial values of radionuclides considered in the present paper
Tableau 1 Valeurs initiales des radionucléides considérés dans le présent article
R | T1/2 (Ma) | D | S | R/S | ref |
|
53 d |
|
|
|
[8] |
|
0.1 |
|
|
|
[67] a |
|
0.3 |
|
|
|
[36,46] |
|
0.74 |
|
|
|
[79] |
|
1.5 |
|
|
|
[51] |
|
1.5 |
|
|
|
[54,70] |
|
3.7 |
|
|
|
[2] |
a
The initial value of
Generally speaking, SRs can be made either by non-thermal nucleosynthesis (nuclear reactions implying cosmic rays at MeV energies), or by thermal nucleosynthesis (nuclear reactions at keV energies in the interior of stars). Short-lived radionuclides once present in the solar protoplanetary disk therefore originated from late-type stars delivering freshly made nucleosynthetic products (e.g., [4]), or by in situ irradiation of solar system dust or gas (e.g., [31]). As far as the stellar model for the origin of short-lived radionuclides is concerned, supernovae are the most likely candidates, because Asymptotic Giant Branch (AGB) stars are unlikely to be found in the vicinity of star-forming regions [37].
Among short-lived radionuclides, some play a special role, such as
The goal of the present article is to establish the irradiation origin of
2 The irradiation origin of
The first evidence for beryllium-10 in the early solar system was brought by [51]. Since then, numerous authors confirmed that beryllium-10 was abundant in the protoplanetary disk, at least at the time and spatial location of CV3 and CM2 CAIs formation [51,68,48,49,8]. Beryllium-10 is produced vastly by any irradiation model [25,45,50], and since its discovery, its presence in the early solar system has been ascribed to the irradiation of nebular dust or gas by protosolar cosmic rays (e.g., [7]). Desch et al. [10] challenged that idea, and proposed that most of the beryllium-10 observed in CAIs was made via Galactic Cosmic Ray (GCR) trapping during the molecular cloud core stage which precedes the formation of a protostar. In the following, we examine this proposal at the light of molecular cloud cores observations.
The amount of
Given the difficulty of trapping
3 Co-production of other short-lived radionuclides by irradiation
3.1 General considerations
As demonstrated above, it is likely that most of the
Beryllium-7 was discovered in the Allende CAI 3529-41 by [8]. Beryllium-7 is a SR with an extremely short half-life (
Evidence for
More generally, from the astrophysical point of view, Gounelle and Meibom [30] showed that it is extremely unlikely that a supernova exploded close enough of a newly formed planetary disk to inject the correct amount of SRs. This is mainly because massive stars evolution timescales are too long, compared to typical timescales of star formation in embedded clusters, for them to explode as supernovae within the lifetime of disks, which will be limited by UV ionization (from the massive stars) and planetary formation.
3.2 Irradiation models
Nucleosynthesis via irradiation of matter in the solar accretion disk by high energy particles (
The main parameters of any irradiation models are the proton flux, the irradiation duration, the abundance of cosmic-rays (
A model examining the possibility of producing short-lived radionuclides by irradiation of solar system dust by proton and
The irradiation model [26,31,44] in the context of the x-wind theory for low-mass star formation (e.g., [66]) introduced four conceptual modifications compared to the previously discussed models. First, irradiation takes place close to the Sun, in a gas-poor region, enhancing considerably the proton flux [44] compared to models that locate irradiation at asteroidal distances, where the proton flux is dramatically reduced by an inverse square law and the interaction with all the gas contained in the solar accretion disk. Second, it has opened the
In the x-wind model, irradiation of protoCAIs takes place in the reconnection ring where magnetic lines tying the protostar and the accretion disks reconnect, providing a powerful mechanism for accelerating
Using the same exact model as [26], Gounelle et al. [31] could reproduce the initial abundance of
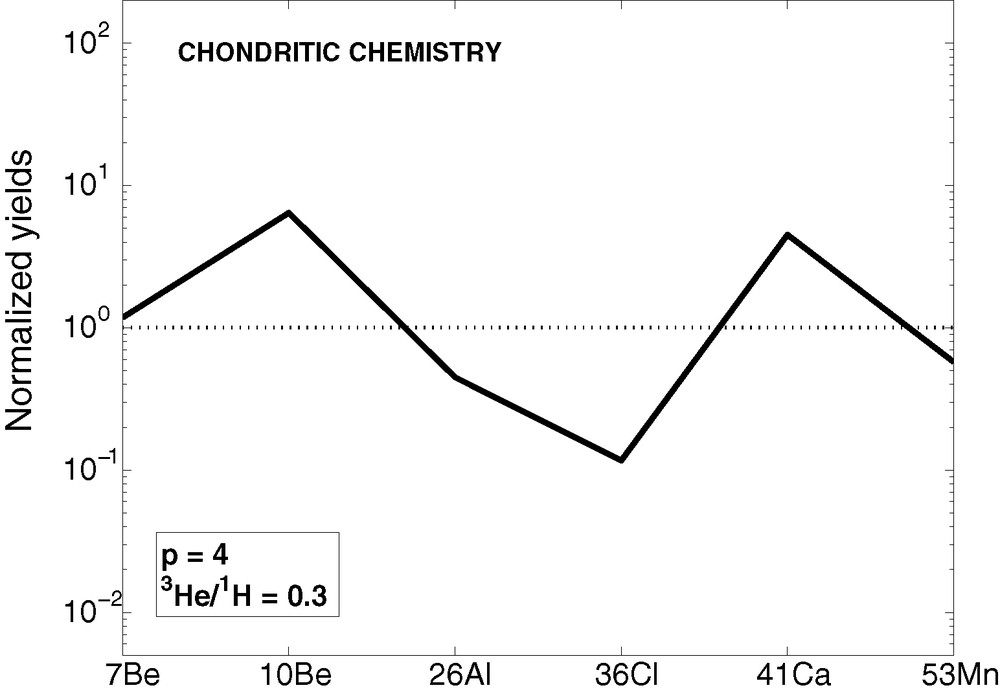
Results of the irradiation model of [31]. Yields of
Results of the irradiation model of [31]. Yields of
Fig. 1. Résultats du modèle d’irradiation de [31]. Abondances produites par irradiation de
Fig. 1. Résultats du modèle d’irradiation de [31]. Abondances produites par irradiation de
The decoupling of
Uncertainties of the model arise mainly from a poor knowledge of the nuclear cross sections, especially for the
4 X-ray evidence for protoplanetary disk irradiation
The variable, intense and ubiquitous X-ray activity of young, solar-like stars is indicative of the acceleration of high-energy particles that have the possibility to irradiate gas and dust from the protoplanetary disk [78]. Although there is no direct evidence for high-energy particles irradiation, there is a growing evidence for X-ray irradiation of the disk dust.
T Tauri stars are low-mass (
In parallel, near- to mid-IR observations, both from the ground and in space, revealed that a significant fraction of T Tauri stars displayed an IR excess, i.e., were more luminous in IR, sometimes by a large factor, than the black-body emission of their photospheres. Using a variety of arguments, it was possible to explain this excess in terms of extended circumstellar disks, with a temperature law decreasing outwards. Direct imaging of young stars, in star forming regions like Orion, proved the existence of protoplanetary disks around these stars, consistent with the laplacian view of the solar system. These disks form the reservoir out of which matter falls onto the star and makes it grow.
It is now observationally proven that all T Tauri stars, whether of not they are surrounded by circumstellar disks, and for all masses, are X-ray emitters [17,21]. About 30 star-forming regions have been observed in X-rays, and they all show this same result. The X-rays are generally seen in the form of hour-long, solar-like flares, and this is interpreted as heating from reconnection of large magnetic loops (up to several stellar radii in a few cases, see, e.g., [15]). The general properties of the X-ray emission can be summarized as: the X-ray emission from T Tauri stars is qualitatively ‘solar-like’, and is a proxy for magnetic fields. It is true that X-rays from accretion shocks onto the star have been also observed, but these cases concern only a handful of stars so far, which are presumably in a state of low magnetic activity (e.g., [62]), compared with thousands of T Tauri stars showing the same magnetic activity in the form of X-ray flares (e.g., [21,32]).
Quantitatively, however, the X-ray luminosity, scaled to the stellar bolometric luminosity (the
For T Tauri stars surrounded by circumstellar disks, such high X-ray luminosities suggest the possibility to directly observe irradiation effects (Fig. 2). Glassgold et al. [24] were the first to compute the ionization effects of X-rays. They adopted a model in which the X-rays were emitted at high stellar latitudes (‘lamppost irradiation’), and the disk was ‘flared’, i.e. geometrically thicker far from the central star because of the decrease of the local gravity. The result was that, except in the equatorial plane where the disk density was highest, resulting in a column density absorbing the X-rays up to
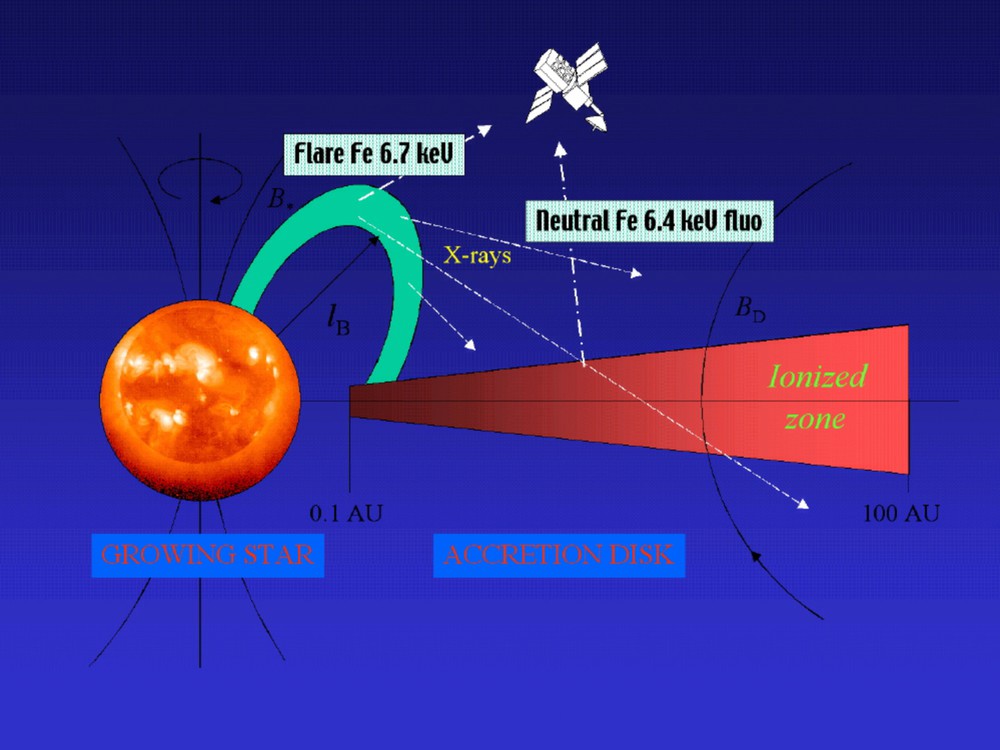
Generic sketch of the irradiation environment of circumstellar disks in protostars and T Tauri stars. The young star is magnetically active (as seen ubiquitously in X-ray observations). Based on the solar analogy, the X-ray emitting models are based on plasma confinement by large magnetic loops (height up to 1–2 stellar radii), either on the star itself or sometimes connecting the star and the disk. The magnetic loops sit at intermediate latitudes, allowing X-ray irradiation in a ‘lamppost’ fashion, out to large distances from the central star (10–20 AU). Among various irradiation effects, two have been directly observed in T Tauri stars: the fluorescence Fe K line at 6.4 keV (as shown here), and very recently the NeII 12.8
Generic sketch of the irradiation environment of circumstellar disks in protostars and T Tauri stars. The young star is magnetically active (as seen ubiquitously in X-ray observations). Based on the solar analogy, the X-ray emitting models are based on plasma ... Lire la suite
Fig. 2. Illustration schématique de l’irradiation des disques circumstellaires autour des protoétoiles et des étoiles TTauri. La jeune étoile est magnétiquement active, comme l’indiquent les observations dans le domaine des rayons X. Les modèles d’émission de rayons X, en analogie avec le soleil contemporain, sont basés sur le confinement du plasma par de grandes boucles magnétiques (jusqu’à 1 à 2 rayons stellaires), attachées à l’étoile elle-même ou parfois connectant l’étoile et le disque. Les boucles magnétiques se trouvent à des latitudes moyennes, permettant une irradiation de type «réverbère» jusqu’à des distances éloignées de l’étoile centrale (10 à 20 AU). Parmi les effets de l’irradiation observés autour des étoiles jeunes, on trouve la florescence de la ligne K du fer à 6,4 keV (illustré ici), et la ligne NeII du néon à 12,8
Fig. 2. Illustration schématique de l’irradiation des disques circumstellaires autour des protoétoiles et des étoiles TTauri. La jeune étoile est magnétiquement active, comme l’indiquent les observations dans le domaine des rayons X. Les modèles d’émission de rayons X, en analogie ... Lire la suite
More recently, Glassgold and collaborators have explored further atomic ionization effects in disk atmospheres. They have shown, in particular, that X-ray irradiation would ionize neon out to 20–30 AU, with the emission of the NeII line at 12.81
Another, purely X-ray evidence for deeper disk irradiation comes from observations of the Fe K fluorescence line at 6.4 keV in a number of T Tauri stars with disks [15,73]. Here, the incoming X-rays, either more or less continuous from coronal emission, or more efficiently after a strong flare, induce a photoelectric effect directly on the ground-level electrons; the subsequent rearrangement of the upper levels by cascade, of Fe in particular, because it normally radiates at 6.7 keV, results in the emission of a 6.4 keV fluorescence photon. This effect had been predicted long ago [20], and is frequently observed in AGNs (‘active galactic nuclei’, which contain a supermassive black hole at their center). There are, however, geometric conditions involved, and the effect is best seen when the disk plane is within a few degrees of the plane of the sky (star viewed ‘pole-on’). This has a low statistical probability of occurring, so only a handful of sources displaying this fluorescence line have been discovered so far.
Even less numerous are T Tauri stars which show both the NeII IR line and the Fe K 6.4 keV fluorescence line (Ophiuchus cloud, Flaccomio et al., personal communication), but as IR and X-ray surveys of star-forming regions continue, the number of combined Ne–Fe detections should increase.
In any case, given the huge number of T Tauri stars with disks now known, which have distinctive common X-ray and IR properties, the fact that a number of them already show evidence for NeII 12.8
5 The initial abundance and origin of
The initial value of
The low part of the range is compatible with a galactic background origin for
Acknowledgments
We thank Anders Meibom for his careful reading of the manuscript and Jean Duprat for his thorough review.